NCBI Bookshelf. A service of the National Library of Medicine, National Institutes of Health.
Benzie IFF, Wachtel-Galor S, editors. Herbal Medicine: Biomolecular and Clinical Aspects. 2nd edition. Boca Raton (FL): CRC Press/Taylor & Francis; 2011.
14.1. INTRODUCTION
“Wolfberry” and “goji berry” are synonyms for one of the best-known and admired berries from China, called gou qi
or kei tze in Chinese. The wolfberry fruit has been used in traditional Chinese medicine (TCM) for more than 2000 years, and its use was first recorded around 200 BCE in Shen Nong Ben Cao Jing
, an ancient book detailing the medicinal and agricultural knowledge of the mythical Chinese emperor Shen Nong. The Shen Nong Ben Cao Jing is the oldest book on Chinese herbs, and records 365 traditional herbs that are classified into three grades: (1) top, (2) middle, and (3) low. Wolfberry is one of the 120 herbs belonging to the top grade, which was believed to have remarkable health benefits and to be harmless to humans. Long-term use of wolfberry was considered beneficial for strengthening the body, keeping fit, prolonging life, and easing life through all the seasons
,
. Another classic TCM treatise, the Compendium of Materia Medica
, a collection of books by Li Shi-Zhen from the sixteenth century, is considered the first pharmacopoeia in the world and the most important one ever written on TCM; in this work, the morphological identification, health benefits, indications, and relevant prescriptions of wolfberry are particularly elaborated. Besides the fruit, use of other parts of the wolfberry plant, including flower, leaf, seed, and root bark, are also recorded. From a TCM point of view, the nature of wolfberry is “calm,” and its flavor is “sweet.” According to TCM theory and practice, wolfberry can act on both the “liver channel” and the “kidney channel,” and the major health benefits of wolfberry are its ability to nourish and tonify liver and kidney, improve jing (
, the basic elements that constitute the body and maintain life activities), and improve eye function (Chinese Pharmacopoeia 2005). It should be noted that wolfberry is used not only as a drug in TCM prescriptions to treat diseases but also as a popular food by Chinese people in their daily life for promotion of general health. According to the regulations of the China State Food and Drug Administration, wolfberry is one of the 87 TCM ingredients that can be used as both normal food and functional food.
The health benefits of wolfberry, which have been recorded from the empirical insights of Chinese doctors over hundreds of years, are today perceived by Chinese consumers as centered on age-related conditions, with benefits primarily for eyesight. This public perception is also reflected in the numerous scientific studies that have been conducted in China in the last 30 years. The earliest work to this effect was published in 1998 by Professor S. Bai of Yinchuan, Ningxia, China, in two books consisting of a total of 462 Chinese research articles on wolfberry. More recent reviews (Gross, Zhang, and Zhang 2006; Sze et al. 2008) discuss the scientific evidence for wolfberry’s bioactivities in the international literature published after the year 2000. Gaps in scientific evidence were identified, and it was cautioned that no evidence exists “to support claims for a quasi-miraculous potion for longevity” (Potterat and Hamburger 2008, 399). This is particularly true for the rather poorly defined wolfberry fraction of polysaccharides and glycoconjugates often referred to as Lycium barbarum polysaccharide (LBP), which is promoted as a unique bioactive with immuno-modulating, antitumor, and antioxidant activity.
Although the benefits of wolfberry are well known and highly thought of in TCM, scientific evidence regarding its benefits is unclear. The objective of this review is to highlight the numerous health benefits of wolfberry from a rigorous scientific and clinical perspective. Arguments and evidence are given in this chapter indicating that LBP is not that unique, and that many of the potential benefits are related to indirect evidence (Sze et al. 2008). We also draw attention to new and refreshing initiatives of researchers, such as Chang and So (2008) who are trying to identify new ways to conduct research on wolfberry, for instance, by looking at its effects in relation to preventing neuronal death in neurodegenerative diseases. New ideas regarding the delivery of bioactives from wolfberry, and other TCM herbs in general (Wang et al. 2005), are also a part of this review.
14.2. DESCRIPTION AND TRADITIONAL USE OF WOLFBERRY FRUIT
Most of the wolfberry fruit produced is dried in the sun, and in China dried berries are consumed as a usual part of the diet. Dried wolfberry is a common ingredient in commercial food products, supplements, and TCM. Wolfberry is frequently added to soups, hot pots, and herbal teas, and is also popularly soaked in wines alone or together with other TCM ingredients to make functional wines.
14.2.1. Origin, Identification, and Characterization of Wolfberry
Wolfberry occurs as a common shrub with delicate, edible leaves and small purple flowers (Figure 14.1). It grows naturally throughout the northern and western regions of China, and when cultivated, the plant reaches a height of about 2 m. The fruit is roughly spindle shaped or ellipsoidal (elliptical in long section and circular in cross section), 6–20 mm long, and 3–10 mm in diameter (Figure 14.2). The fruit contains 20–50 seeds and has a sweet and fruity taste (Zhao 2004). The two main species cultivated in China are L. barbarum L. and L. chinense Miller. The species L. barbarum is widely cultivated for medicine in north and northwest China, especially along the Yellow River in Ningxia Province. Almost half of the commercial wolfberry fruit produced in China is from Ningxia, and most of it is L. barbarum. According to TCM insight, among all regions of China, Ningxia Province has long been considered the site of “authentic origin” of wolfberry. Generally, authentic origin means that a given region has the most suitable growing environment (e.g., soil and climate), a long production history, and a large production volume of a specific herb. Accordingly, an herb produced under such conditions is called an “authentic herb” (daodi herb in Chinese,
and is considered to be of higher quality than the same type of herb produced in other regions. The reference for Ningxia as the authentic origin of wolfberry can be traced back to the Compendium of Materia Medica. This traditional knowledge has been confirmed by modern analysis; as it has been demonstrated that wolfberry from Ningxia contains higher amounts of active ingredients and nutrients (Wang 2003; Li and Zhang 2007). The species L. barbarum is also indigenous to Europe as described in Flora for Germany, Austria, and Switzerland (Thomé 1885), although there is no evidence for a traditional consumption of its fruits in Europe. L. chinense, which is a smaller relative, is widely found in China and grows also in Nepal, Pakistan, Thailand, and Europe (Wu and Raven 1994).

FIGURE 14.1
(See color insert.) Fresh, mature wolfberry fruit.

FIGURE 14.2
Wolfberry fruit drying in the sun.
14.2.2. Use of Wolfberry in Traditional Chinese Medicine
Wolfberry is mainly used in TCM for treating “yin deficiency” in liver and kidney. The dried fruit is commonly used in TCM preparations at a dose of 6–15 g, taken twice or thrice daily. It is used as a decoction in formulas for treating yin deficiency and liver “qi stagnation” (Liu and Tseng 2005). Wolfberry can also be a part of a mix of Chinese herbs that is ground to a fine powder and used in honey pills (a traditional TCM formulation in which honey is used as main excipient to make pills) of 15 g each. One of these pills is taken with bland soup in the morning and another at night on an empty stomach (Liu and Tseng 2005).
14.2.3. Use of Wolfberry inFoods, Beverages, and Nutricosmetics
Wolfberry is widely used in herbal teas aimed at weight control (with cassia, poria cocos, chrysanthemum, and other herbs), antiaging (with astragalus, ganoderma, and others), and liver protection (with licorice, ganoderma, gynostemna pentaphylla, and others). Consumer survey studies conducted among urban Chinese indicate that tea is one of the most preferred carriers for wolfberry. In South China, where awareness of Chinese herbs and their health benefits is more pronounced than in the rest of the country, wolfberry is commonly used as a tonic ingredient in soups and to make sweet or savory porridge. Wolfberry is also frequently added to traditional hot pot (spicy and nonspicy), a very popular form of healthy eating in southwest China (Sichuan, Hunan) and the big cities (Shanghai, Beijing, Guangzhou). The use of wolfberry has become prevalent in processed foods and beverages, and new product launches have multiplied in recent years in China. Even more surprising are similar product launches in the United States, where wolfberry was rather unknown before 2005 (Table 14.1).
TABLE 14.1
Number of Product Launches in Different Years for Food Products Containing Wolfberry in china and the united states.
Wolfberry is one of the most popular TCM herbs regulated as a foodstuff that is used in nutricosmetic products in China. Nutricosmetics are used for the promotion of skin and hair health. Only angelica and pearl powder are more frequently found in nutricosmetic products in China.
14.2.4. Magnitude of Wolfberry Usage
In TCM practice, one can note that herbal formulas with wolfberry do not normally contain more than 15 g of wolfberry fruit, and these preparations are taken a maximum of three times a day. Wolfberry is generally washed and cooked together with food (as soup, porridge, hot pot) or mixed with boiling or hot water to make herbal tea. It is difficult to know how much wolfberry is consumed on an individual basis. As an indication, total wolfberry fruit production in China was estimated in 2004 at 95,000 tons of dried fruit. According to Chinese practice, wolfberry is more often used by people over 50 years, as it is considered to have antiaging properties, to support immune function, and to protect eyesight. As wolfberry is one of the best-known Chinese herbs and food ingredients, and is commonly found for sale in supermarkets all over the country, it is likely that a large part of the Chinese adult population consumes some wolfberry fruit. Furthermore, wolfberry is very popular among Chinese living outside China. A study among Chinese in the Chinatowns of Oakland and San Francisco in the United States revealed that wolfberry fruit was used by 11% of respondents. It was the third most widely consumed Chinese herb among the 378 persons interviewed in this study, behind only ginseng and a popular cooling herb decoction called qing bu liang (a mixture of herbs cooked in soup that consists generally of seven standard herbs: dioscorea, lily bulb, dried polygonatum, fox nut, pearl barley, dried lotus seed, and dried longan; Cheng et al. 2004).
14.2.5. Evidence for Safety and Health Concerns from Diverse Human Experience
In the very long history of the traditional use of wolfberry, there are practically no reports of adverse effects. Published human safety data are rare. Wolfberry contains betaine, which is a known liver protectant, but can also be used to induce menstruation and abortion, so its use should be avoided by pregnant women. Chinese herbalists suggest abstaining from using wolfberry during times of cold and flu. Wolfberry has an estrogen-mimicking effect (Zhao 1998), so it should not be used by people who are pregnant or have diseases that are sensitive to estrogen.
14.3. IDENTIFICATION OF BIOACTIVES
The scientific literature of the last 20 years has documented that wolfberry fruit contains several potentially bioactive components (Gross, Zhang, and Zhang 2006; Potterat and Hamburger 2008; Sze et al. 2008). In Sections 14.3.1 through 14.3.3, we focus on three of these: arabinogalactanproteins (AGPs), the carotenoid zeaxanthin, and the vitamin C precursor 2-O-(β-D-glucopyranosyl) ascorbic acid, and discuss their potential functionality and nutritional relevance.
14.3.1. Arabinogalactan-Proteins: Bioactive Glycoconjugates in Lycium Barbarum L.
The literature documents that wolfberry fruit contains soluble macromolecules that exhibit a range of bioactivities. These properties are attributed to soluble glycoconjugates that were identified as “arabinogalactan-proteins” (AGPs), but are mostly referred in the Chinese literature as “L. barbarum polysaccharide” (LBP). Different molecular forms of wolfberry glycoconjugates (LbGp) have been partially characterized and their structural features and immunomodulating properties described.
14.3.1.1. Isolation and Structural Features of Glycoconjugates
Isolation of glycoconjugates is normally done from air-dried wolfberry fruit. There are no reports of their isolation from freshly harvested fruit. This may impact the reported structural features, as the postharvest history of fruit is known to be a factor in the metabolic status of endogenous enzymes. These can modify the structure of the polysaccharides during extraction unless precautions are taken to inactivate them early in the extraction process. In several publications, LbGps are reported to be extracted by a procedure developed by Huang et al. (1998). Dried and powdered wolfberries were stirred overnight in water and the supernatant concentrated and treated with four volumes of absolute alcohol to precipitate the polysaccharides. Proteins were removed by several washes with chloroform:butanol (4:1 v/v). The crude polysaccharide fraction (LBP) was then subjected to anion exchange chromatography on diethylaminoethyl (DEAE)-cellulose and gel permeation chromatography on Sepharose 4B to yield three glycoconjugates: (1) LbGp3, (2) LbGp4, and (3) LbGp5 (Huang et al. 1998). Their molecular weights were 925, 215, and 24 kDa, respectively. The glycoconjugate LbGp3 was composed of arabinose and galactose in a ratio of 1:1. Carbohydrate accounted for 94% of the fraction. LbGp4 was composed of arabinose, galactose, rhamnose, and glucose in a molar ratio of 1.5:2.5:0.43:0.23. Carbohydrate accounted for 86% of this fraction. Finally, LbGp5 was composed of rhamnose, arabinose, xylose, galactose, mannose, and glucose in a molar ratio of 0.33:0.52:0.42:0.94:0.85:1.0. The carbohydrate content was 9% in this fraction. The elemental N content of LbGp3, LbGp4, and LbGp5 indicated protein levels of around 5%, 10%, and 60%, respectively. Subsequently, the authors investigated in more detail the structural features of each of the three glycoconjugates as well as reporting their bioactive properties. Peng, Huang et al. (2001) examined the structural features of LbGp4 by methylation analysis to identify linkage types and 1H- and 13C-NMR to establish the anomeric configuration of the sugars. The glycan moiety (LbGp4-OL) was released by β-elimination, and the monosaccharide composition showed arabinose, galactose, and rhamnose in the molar ratio of 1.33:1.0:0.05. A putative repeat unit was proposed (Figure 14.3).

FIGURE 14.3
A reported repeat unit of the wolfberry glycan chain LbGp4-OL. (From Peng, X. M., L. J. Huang, C. H. Qi, Y. K. Zhang, and G. Y. Tian. 2001. Chinese J Chem 19:1190–7. With permission.)
A similar approach was adopted with LbGp3 (Huang, Tian, and Zheng 1999), but this time the glycan was released by a pronase treatment. The glycan contained arabinose and galactose in a molar ratio of 1:1. A structure was proposed for which, although the detailed structural features of the side chains differed, the essential features were the same as the LbGp4-OL, as shown in Figure 14.4. That is, a backbone of β-(1→4)galactosyl residues with mainly arabinosyl substituents attached at O-3. Peng, Qi et al. (2001) separated the glycoconjugate LbGp5 into three additional fractions: Lbp5A, Lbp5B, and Lbp5C. No structural features were reported by the authors, but LbGp5B, which contained rhamnose, arabinose, glucose, and galactose in the molar ratio 0.1:1.0:1.0:1.2:0.3, also contained 9% galacturonic acid. The amino acid composition of the protein moiety consisted of 17 amino acids, but the presence of hydroxyproline was not reported. However, a more recent paper did report the presence of hydroxyproline in the protein moiety of a glycoconjugate isolated from wolfberry (Zhang and Chen 2006). This finding is significant as hydroxyproline is a common amino acid component of the protein associated with AGPs.

FIGURE 14.4
Structure of zeaxanthin.
The results suggest that the water-soluble polysaccharides in wolfberry are a complex mixture of several different types of polysaccharides. However, the chromatographic methods used to separate these different polymers are insufficient to completely purify the glycoconjugates. This is indicated by the presence of additional monosaccharides such as xylose, mannose, glucose, and galacturonic acid, which are not commonly found in AGPs, in some of the glycoconjugates. The cell walls of most dicotyledonous plants are rich in pectic polysaccharides and it would be expected that wolfberry also contains moderate amounts of soluble pectic polymers. A recent publication by Zhang and Zhang (2007) reported the presence of 56% galacturonic acid in LBP, as well as six different monosaccharides. It is likely that pectic polysaccharides are present in LBP and other glycoconjugates that have been prepared in the past. A matter of perhaps greater significance is that in this paper it is reported that the backbone of the polymer is composed of (1→3) glycosidic bonds (Zhang and Zhang 2007). The study of the structural features of LbGp4 conducted by Peng, Huang et al. (2001) reported that that “glycans of the arabinogalactan-protein under investigation is based on highly branched 3,4-linked galactans, a finding in contrast to most of the known AGPs which carry 3,6-galactans. It therefore represents a novel structure ...” In light of the report by Zhang and Zhang (2007), unpublished data from our laboratory, and current knowledge on the structure of AGPs, the stated novelty of L. barbarum AGPs in possessing a (1→4)-linked galactosyl backbone must be doubted.
The Yariv reagent (Jermyn and Yeow 1975) is a compound capable of specifically interacting with AGPs (Van Holst and Clarke 1985). Experiments in our laboratory used the reagent to precipitate the AGP fraction from a water-soluble extract of wolfberry. The recovered polysaccharide contained arabinose, galactose, rhamnose, and uronic acid in the molar proportions of 1.0:1.0:0.075:0.2, respectively. Most of the uronic acid was identified as glucuronic acid, which is known to occur as terminal residues on the side chains of some AGPs. Linkage analysis indicated a backbone of (1→3)-linked galactosyl residues, some of which carried substituents at the O-6 position. This structure is in line with the established structural features of many of the type II Arabinogalactans (AG)s characterized in AGPs to date. The non-Yariv precipitable polysaccharide fraction contained 40% galacturonic acid, indicating the predominant presence of pectic polysaccharides. This fraction also contained the xylose, mannose, and glucose contents of the original water-soluble fraction, evidence that they were not structural features of wolfberry AGPs.
14.3.1.2. Optimizing Lycium barbarum Polysaccharide Recovery From Wolfberry
Experiments conducted in our laboratory showed that cold water extraction can solubilize 2–3% of dried wolfberries as high-molecular-weight polysaccharides. Of this amount, around 0.4% is present as a Yariv-precipitatable fraction and, therefore, represents the glycoconjugates identified as AGPs. Pectic polysaccharides accounted for most of the remaining soluble polysaccharide. The cold water–insoluble polysaccharide fraction accounted for approximately 20% of the wolfberries and consisted of a mixture of cellulose and pectic and hemicellulosic polysaccharides, commonly occurring in the cell walls of dicotyledonous fruit. Increased yields of LBP have been reported by prolonged high-temperature extraction (Yin and Dang 2008) or enzymatic treatment (Yan et al. 2007). Since the AGPs are readily dissolved in cold water, it is unlikely that significantly greater amounts of the glycoconjugates will be solubilized by a more severe extraction procedure. Most of the increased levels of polysaccharides recovered by these approaches are likely to be pectic and hemicellulosic polymers derived from the breakdown and solubilization of the insoluble cell walls of the wolfberry fruit.
14.3.1.3. Bioactive Properties
The bioactive properties of arabinogalactans and AGPs are, in general, well documented (Redgwell and Fischer 2005). Larch arabinogalactan (not an AGP) is marketed as ImmunEnhancer AG (Larex, Inc., Minnesota). Apart from its role in digestive health promotion (prebiotic properties resulting from it being fermented to short-chain fatty acids, butyrate, and propionate), ImmunEnhancer can enhance immune system performance (D'Adamo 1996). It functions in this capacity by preventing bacteria and viruses from attaching to cell membranes on the liver and other organs, thereby stopping infections from becoming established. Tumor metastasis to the liver has been reported to be impaired by Larch AG (Beuth et al. 1987; Hagman et al. 1991).
The benefits of AG and AGPs do not appear to be related to a source but seem to be a consequence of the structural features they have in common. Therefore, it is logical that wolfberry AGPs demonstrate the same bioactive properties as those documented for AGs and AGPs from other plant sources. Since the mid-1980s, more than 200 papers have been published on the aspects of chemistry and health benefits of the fruit of L. barbarum. More than 50 of these refer to functionalities specifically related to the polysaccharide content. However, many of these studies have used a “soluble polysaccharide” extract and not a purified glycoconjugate. Therefore, not all reported bioactivities can necessarily be attributed solely to the AGP content of the fruit.
The research focus has been on mainly three interrelated areas: (1) modulation of the immune system, (2) antitumor activity, and (3) antioxidant activity. An early study (Geng et al. 1989) reported that LBPs stimulated T lymphocytes to produce interleukin (IL)-2 in the elderly. Interleukin-2 was one of the first soluble “hormonelike” mediators of the immune system discovered, and it fueled interest in the field of immunology, as the vital role of cytokines had not been realized prior to its discovery. Using a partially purified glycoconjugate (LBP3p), Gan et al. (2003, 2004) confirmed the earlier study that showed the glycoconjugate’s effectiveness in increasing, in a dose-dependent manner, the expression of the cytokines IL-2 and tumor necrosis factor α (TNF-α) at both messenger ribonucleic acid (mRNA) and protein levels. In a clinical trial conducted on 75 cancer patients, LBP was orally administered in combination with lymphokine-activated killer cells and IL-2. This treatment led to a significant regression of cancer compared to treatment in the absence of the lycium polysaccharides (Gau, Yang and Du 1994). Peng, Huang et al. (2001) demonstrated the high immunoactivity of the purified glycoconjugate LbGp4. The mechanism was investigated using a tritium thymidine incorporation assay, flow cytometry, and electrophoretical mobility assays. The glycoconjugate LbGp4 and its glycan promoted splenocyte proliferation in mice, and the effects of the glycan chain were stronger than those of the glycoconjugate. Zhang et al. (2005) showed a positive effect of an ill-defined “polysaccharide-protein complex” by lessening proliferation and increasing apoptosis of the human hepatoma cell line QGY7703 associated with liver cancer. More recently, the effect of an LBP on the growth of human prostate carcinoma PC-3 cells was studied (Luo et al. 2008). Results indicated that LBP can inhibit the growth of PC-3 cells and induce apoptosis. LBP-induced breakage of DNA strands in PC-3 cells and the ratio of Bcl-2/Bax protein decreased. The expression of Bax is upregulated by the tumor suppressor protein p53, and Bax has been reported to be involved in p53-mediated apoptosis.
Evidence for the antioxidative ability of lycium polysaccharides is documented in several publications based on in vitro and several in vivo studies, although few of them have been performed with a purified glycoconjugate fraction. Li, Peng, and Wang (2007) evaluated the antioxidative capacity of LBP by in vitro methods including superoxide radical-scavenging activity, reducing power, β-carotene linoleate model, and inhibition of mice erythrocyte hemolysis mediated by peroxyl radicals. They concluded that the data clearly established the antioxidative potency of LBPs. Studies with rats and mice have demonstrated that LBP can lessen oxidative stress and DNA damage (Li 2007; Li and Zhou 2007; Wu et al. 2006), inhibit lipid oxidation induced by free radicals in a high-fat diet (Ma et al. 2009), and protect against oxidative damage in skeletal muscle caused by exhaustive exercise (Niu et al. 2008). Zhao et al. (2005) reported that LbGp5 promotes the survival of human fibroblasts cultured in suboptimal conditions and, therefore, may have skin-protective properties. Additional claims on the benefits of LBP include antifatigue (Luo, Yan, and Zhang 1999) and cholesterol-lowering activities (Luo, Li, and Zhang 1997; Yu, Wu, and Niu 2009).
It must be kept in mind that wolfberry is rich in a number of potent antioxidants that are not polysaccharides, such as zeaxanthin and polyphenols. The phenolic derivatives in particular are likely to interact with and bind to the polysaccharides and proteins during the extraction of LBP, and they could subsequently copurify with the LBPs during alcohol precipitation, as for tea polysaccharides of an AGP nature (Zhou et al. 2009). It is a definite possibility that some of the reported antioxidant effects of wolfberry polysaccharides are in part the result of contaminating low-molecular phenolic compounds.
In summary, polysaccharide extracts from the fruit of L. barbarum L. demonstrate a broad spectrum of bioactive properties, many of which are attributed to the presence of AGPs or glycoconjugates, although it is noted that antioxidant activity may be due to the contamination of polysaccharides fractions with phenolic compounds. Polysaccharides from wolfberry appear to possess several of the properties already claimed for AGPs and AGs from other plant sources. Whether LBPs possess additional benefits or possess more potent bioactivity than AGPs from other plants remains to be proved. Ongoing research to refine the isolation and purification of the several polysaccharide types of L. barbarum will clarify the specific role played by glycoconjugates and the other polysaccharides in these benefits.
14.3.2. Zeaxanthin
Zeaxanthin is a xanthophyll, an oxygenated carotenoid (Molecular weight (MW) 568.85 gmol-1) with antioxidant and blue light-absorbing properties (Figure 14.4). Zeaxanthin is found in many vegetables and fruits at low levels, with wolfberry being its best-known natural source (Weller and Breithaupt 2003). Earlier published works referred to β-carotene as the main carotenoid of wolfberry fruit; however, in 1999, two groups (Lam and But 1999; Zhou et al. 1999; Li et al. 1999) independently reported that the main carotenoid of wolfberry is zeaxanthin dipalmitate. The finding of high zeaxanthin content in wolfberry was substantiated by further studies, and Weller and Breithaupt (2003) confirmed that 80% of the total carotenoids in wolfberry were in the form of zeaxanthin dipalmitate. More detailed analysis revealed that zeaxanthin dipalmitate (1.14 mg/g dry weight) was present in the largest amount (88% of total carotenoids), followed by β-cryptoxanthin monopalmitate and its two isomers (2–4%), zeaxanthin monopalmitate and its two isomers (1–5%), all-trans-β-carotene (2%), and all-trans-zeaxanthin (0.1%) (Inbaraj et al. 2008).
14.3.2.1. Estimated Zeaxanthin Intake: Potential Contribution from Wolfberry
Sommerburg et al. (1998) investigated the presence of zeaxanthin in 33 vegetables and fruits. Orange pepper (37% of total carotenoids) was found to be the vegetable with the highest amount of zeaxanthin. They found that dark-green leafy vegetables, previously recommended for a higher intake of lutein and zeaxanthin, had very low amounts of zeaxanthin (0–3% of total carotenoids). By far, the richest source known for zeaxanthin is wolfberry fruit, a quasi “natural pill,” enriched in a component that appears essential for eyesight (Landrum and Bone 2001; Cheng et al. 2005). The zeaxanthin content of wolfberry depends on the stage of maturity, season, and the drying process (Ma et al. 2008), and in our studies we found that it varies from 1.18–2.41 mg/g dried fruit.
Data on estimated zeaxanthin intake and bioavailability are scarce, because before the year 2000 the two carotenoids lutein and zeaxanthin were not measured separately by high-performance liquid chromatography (HPLC), and much of the data available are expressed as combined intake of both lutein and zeaxanthin (Table 14.2). It appears that estimated zeaxanthin intake is generally <1 mg/day. From the fact that wolfberry zeaxanthin content is approximately 0.8 mg/g, the richness of wolfberry as a source of zeaxanthin is clear.
TABLE 14.2
Referenced Data on Estimated Daily intake of Zeaxanthin.
Eating a few grams of wolfberry each day would certainly boost plasma levels of zeaxanthin, as was reported for synthetic zeaxanthin in a human supplementation study with 1 or 10 mg/day for 42 days (Hartmann et al. 2004).
14.3.2.2. Mechanism of Intestinal Zeaxanthin Absorption
In the stomach, carotenoids and lipid molecules separate from the rest of the chyme and form oil droplets, which enter the small intestine and are mixed with pancreatic juice and bile salts (Tyssandier et al. 2001). The oil droplets get covered by bile salts, which creates a negative surface charge that permits the binding of colipase to the lipid/aqueous interphase. The colipase then binds to the pancreatic lipases, which hydrolyzes the bond between zeaxanthin and fatty acids, for instance, palmitic acid for wolfberry (Borel et al. 1996; Chitchumroonchokchai and Failla 2006). The liberated zeaxanthin is absorbed by the intestine (Perez-Galvez et al. 2004). It is unknown whether a part of zeaxanthin is re-esterified during the absorption process, but some esterified zeaxanthin has been detected in plasma and tissues (Granado et al. 1998). The micellar solubilization of zeaxanthin into lipids is mandatory for their absorption. There is increasing evidence of the existence of a facilitated, protein-carrier-mediated transport of carotenoids (Reboul et al. 2005; During, Dawson, and Harrison 2005). Once absorbed into the enterocyte, zeaxanthin is packed into new oil-droplet structures called prechylomicrons, which are transported into the extracellular space by exocytosis before entering the lymphatic system and general circulation.
14.3.3. The Vitamin C Precursor 2-O-(β-D-Glucopyranosyl) Ascorbic Acid
Plants and, in particular, their fruits are generally a good source of ascorbic acid (vitamin C). Earlier compositional data about wolfberry lacked mention of any significant amounts of ascorbic acid. The mystery was lifted only when researchers from Suntory, Ltd., Japan, isolated, identified, and synthesized an ascorbic analog as 2-O-(β-D-glucopyranosyl) ascorbic acid (Maeda, Nakao, and Fukami 2003; Toyoda-Ono et al. 2004). The levels of acid found in wolfberry (0.5%) were similar to those known for lemons, making wolfberry one of the richest sources of ascorbic acid. Glucoside was found in animal experiments to be hydrolyzed into ascorbic acid and glucose, which makes it a promising source of natural vitamin C for food and beverage applications where thermal and acid treatments are needed. Some of the physical features of this molecule can be extrapolated from a synthetic and commercially available isomer named 2-0-(α-D-glucopyranosyl) ascorbic acid (Hayashibara, Co., Okayama, Japan). Our tests with an acidified sugar-containing beverage at a pH of 3.5 revealed remarkable stability of the vitamin C precursor when compared with a similar beverage made with ascorbic acid (Figure 14.5). Although the fate and potential physiological benefits of this vitamin C precursor have not yet been reported, it cannot be denied that the presence of this precursor in TCM preparations will have a significant impact on the health status of patients or weak persons. Regularly taken, such as prescribed in TCM formulas (15–30 g/day), the precursor would add 45–90 mg of ascorbic acid equivalents to the daily intake, which is within the current recommended daily intake limit.

FIGURE 14.5
Stability of vitamin C precursor (AA2G) versus ascorbic acid (AA) in a beverage at 30°C.
14.4. BIOAVAILABILITY OF WOLFBERRY BIOACTIVES AND EFFECTS OF PROCESSING
Wolfberry is a rich source of bioactives that may act synergistically. As the fruit contains both watersoluble (e.g., LBP, vitamin C precursor) and fat-soluble (e.g., zeaxanthin) bioactives, the bioavailability and nutritional properties of wolfberry and its products are likely to be affected by the method of extraction and incorporation into a product. Conventional extraction techniques extract only water-soluble compounds of wolfberry, as described, for instance, for commercial LBP extraction (Yin and Dang 2008). Hot water extraction is a standard practice of TCM. For wolfberry, the use of hot water allows the extraction of hydrophilic compounds, but most of the lipophilic components such as zeaxanthin dipalmitates, lipophilic vitamins, and other lipids are lost. Food is an integral delivery system. Eating diets rich in fruits and vegetables is known to be beneficial to health. But the benefits of eating fruits and vegetables may not be reproduced by consuming purified extracts of phytonutrients or supplements with vitamins (Sesso et al. 2008). Evidence suggests that it is the complementary and/or synergistic effects of several of these phytonutrients that promotes health or produces protective effects against disease, and that isolating one or another of them is less beneficial for human nutrition than consuming the whole food in which a phytonutrient is found. Today, the consumer expects to get the benefits of these phytonutrients in efficient, convenient, and natural formulations. Therefore, from natural bioactives to tasty foods, a new approach must preserve the integrity of the bioactive raw materials. That is, it must be possible to deliver all intrinsic benefits of a fruit, vegetable, or other plant-based materials, including TCM herbs, in their natural, stable, and bioavailable form. To meet such an expectation, innovative ways of delivering the healthy properties of plant extracts are needed, in particular of identifying the most suitable extraction process, analyzing the chemical stability and bioavailability of the extracts, and verifying eventual interactions to the specific food matrix.
14.4.1. Lacto-Wolfberry: The Generation of a Natural, Stable, and Bioavailable Formulation
Although wolfberry is commonly used in TCM, it is challenging to include this fruit in commercial food and beverage products. The Nestlé Research Center (Lausanne, Switzerland) has developed a process that makes it possible to deliver the full benefits of the whole fruit in a simple, natural, and tasty formulation called Lacto-Wolfberry. In this process, milk is used as both an extracting agent and a carrier for the retention of water- and oil-soluble bioactives of the whole fruit (Wang et al. 2005). The process consists of the following three key steps: (1) milling the fruit in milk or in milk protein-containing solution, (2) separating insoluble fibers to obtain an aqueous suspension, and (3) optionally drying the suspension to obtain a powder (Figures 14.6 and 14.7). Such a process is particularly suitable for converting lipophilic bioactives of raw materials into water-soluble formulations. The Lacto-Wolfberry formulation has a profile advantageously close to that of the essential active components of the wolfberry fruit, and has good stability, miscibility, and dispersibility in aqueous systems. In addition, Lacto-Wolfberry was found to have enhanced nutritional value, in the form of significantly better zeaxanthin bioavailability and stability. It was demonstrated in a clinical study (Benzie et al. 2006) that the bioavailability of zeaxanthin from Lacto-Wolfberry is significantly (three times) higher than that from wolfberry powder (Figure 14.8).
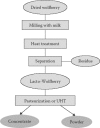
FIGURE 14.6
Flow diagram of the Nestlé Lacto-Wolfberry process.

FIGURE 14.7
Different stages of the Nestlé Lacto-Wolfberry process.
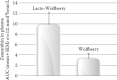
FIGURE 14.8
Mean zeaxanthin uptake in terms of area under the curve (AUC) 0–10 hrs (area under the curve in 12 healthy adults) from Lacto-Wolfberry versus a powdered wolfberry preparation, each containing 15 mg of zeaxanthin. (Adapted from Benzie, I. F. F., (more...)
14.4.2. Bioavailability of Zeaxanthin from Wolfberry
Zeaxanthin is concentrated within the central macula of the human retina (Landrum and Bone 2001). Because of the richness of wolfberry in zeaxanthin and its perceived benefits for eyesight, it was speculated that the consumption of wolfberry will affect health positively. One of the first studies that tested this hypothesis was done with rhesus monkeys (Leung et al. 2001) that were fed for 6 weeks a diet containing a carotenoid fraction extracted from wolfberry fruit (2.2 mg zeaxanthin/day).
The authors of this study observed that the serum levels and macular density of zeaxanthin were raised in these monkeys when compared with monkeys fed a control diet without carotenoids. The serum and tissue levels of zeaxanthin and lutein were studied. After feeding wolfberry extracts, serum levels and macular density of zeaxanthin were found to be increased. This observation was followed by several human intervention studies that revealed absorption of zeaxanthin. Breithaupt et al. (2004) reported the first human intervention study that used a fat-soluble fraction of wolfberry and compared it to the absorption of synthetic zeaxanthin (DSM Nutritional Products, Kaiseraugst, Switzerland). The blood plasma levels of zeaxanthin increased for both zeaxanthin supplements, showing a maximum between 9 and 24 hours after ingestion. Although the results were not statistically significant, with a high variability in the uptake of the wolfberry sample, they indicated that zeaxanthin from wolfberry was better absorbed than that from synthetic nonesterified zeaxanthin. Cheng et al. (2005) found in a 28-day supplementation trial that ingestion of 15 g of wolfberry (whole berries) per day markedly increased plasma levels of zeaxanthin (2.5-fold increase). A study by Hartmann et al. (2004) indicated that supplementation with 10 mg of synthetic zeaxanthin increased the plasma concentrations of zeaxanthin by a factor of 20.
14.5. IMMUNOMODULATING ACTIVITY
Wolfberry has been prized in China for many years for its immune system-boosting property; however, clinical data demonstrating the effects of dietary intake of wolfberry on immune responses are lacking, and there is little international scientific literature on its effects and the mechanisms by which it exerts its reputed immunological benefit. Most of the reported data about the immunomodulation effects of wolfberry come from animal, ex vivo, and in vitro studies, and are mostly attributed to its polysaccharide fraction (LBP). Nevertheless, one cannot exclude the possibility that compounds such as antioxidants and vitamins present in wolfberry could also be involved in immunomodulation. Immune cells are known to be particularly sensitive to oxidative stress because their plasma membranes contain a high percentage of polyunsaturated fatty acids. Therefore, wolfberry could exert an immune-enhancing effect through, for example, the presence of zeaxanthin, a potent lipophilic antioxidant.
Human studies of immunomodulation by wolfberry are rare. In a rodent model, subcutaneous injection of wolfberry juice resulted in an increase in the size of the thymus and the spleen, as well as an increase in T lymphocytes number and rate of T cell maturation (Wang, Xing, and Zhou 1990). Healthy mice receiving an intraperitoneal injection of wolfberry juice combined with blueberry and raspberry juices showed an increase in spleen size and in the number of splenic macrophages compared to mice treated with a saline control (Chao et al. 2004). An increase in the number of splenic macrophages in a healthy animal would indicate an increased ability to fight disease since macrophages are the main phagocytic cells in the spleen, which provide an essential defense by filtering infectious agents from the blood. But, notably, no effect was observed with injection of pure wolfberry juice.
14.5.1. Role of Lycium barbarum Polysaccharides
The immunomodulation effect of wolfberry has been mainly attributed to the presence of LBPs in the fruit. In vitro experiments show that LBPs exhibit immune-enhancing properties by increasing the proliferation of mouse spleen cells, induced or not by a T cell mitogen (i.e., ConA or PHA; Geng et al. 1989; Duan et al. 2001; Huang et al. 2001; Peng, Huang et al. 2001; Peng, Qi et al. 2001; Peng, Wang, and Tian 2001; Qi, Zhang et al. 2001; Du, Liu, and Fang 2004; Chen, Kwong Huat Tan, and Chan 2008) or a B cell mitogen (i.e., lipopolysaccharide [LPS]; Peng, Huang et al. 2001). Consistent with the in vitro data, it has been reported that LBP given either intraperitoneally or orally to mice resulted in a stronger proliferation of spleen cells after in vitro culture in the presence or absence of mitogen (Peng, Wang, and Tian 2001; Chen, Kwong Huat Tan, and Chan 2008). In addition, mice receiving intragastric administration of LBP (LBP-X at 5–20 mg/kg/day for 6 days) showed increased SRBC-specific humoral response (SRBC is a T cell-dependent antigen) and delayed-type hypersensitivity (DTH) response (Luo, Yan, and Zhang 1999), indicating that LBPs selectively strengthen T cell-mediated immune responses.
Mitogen-induced lymphocyte proliferation and IL-2 production significantly decrease with aging and are surrogate biomarkers of aging. IL-2 acts mainly on mitogen- or antigen-activated T cells to promote their survival and proliferation in vitro and to potentiate the function of cytotoxic T cells. It has been demonstrated that LBP enhances in vitro proliferation of lymphocytes from normal adult mice, aged mice (Geng et al. 1989), and senescence-accelerated mice (Huang et al. 2001). Interestingly, LBP was shown to raise the level of IL-2 produced by cells from aged mice to the same level as that produced by cells from adult mice (Geng et al. 1989). Furthermore, intragastric administration of crude LBP (100 mg/kg daily for 8 weeks) in a D-galactose-induced mouse model of aging resulted in increased proliferation of spleen cells and expression of IL-2 (Deng et al. 2003). An increased superoxide dismutase (SOD) activity of erythrocytes and a decreased level of serum advanced glycation end products (AGE) were also observed, indicating that LBP can prevent the decline of immune function observed with aging by inhibiting the production of AGE (Deng et al. 2003). Taken together, these studies support the antiaging effect of wolfberry by strengthening T cell-mediated immune responses.
The immunomodulatory effects of LBP on dendritic cells (DC), one of the most potent antigen-presenting cells that plays a pivotal role in the initiation of immune response (Banchereau and Steinman 1998; Lanzavecchia and Sallusto 2001), has been evaluated (Zhu, Zhao, and Chen 2006; Zhu et al. 2007). It was found that LBP stimulated the cell surface coexpression of MHC class II molecules and CD11c and the secretion of IL-12 p40 by bone marrow-derived DCs (BMDCs) in vitro. In addition, LBP inhibited mannose-receptor-mediated endocytosis by DCs and augmented their capacity to promote the proliferation of naive allogenic T cells (Zhu, Zhao, and Chen 2006; Zhu et al. 2007). Together, these results suggest that LBPs are capable of promoting the phenotypic and functional maturation of DCs, making them ready for T cell-mediated immune responses. Further, it was demonstrated that LBPs have immunomodulatory effects on macrophages (Peng, Wang, and Tian 2001; Li et al. 2005; Li, Ma, and Liu 2007). It was found that LBPs increased the phagocytic activity of macrophages and nitric oxide (NO), and IL-1β and TNF-α production in vitro (Li et al. 2005). Gastric administration of LBP (200–500 mg/kg for 30 days) enhanced phagocytic index and activity in a senescence-accelerated mouse model (Li, Ma, and Liu 2007). In this context, it is interesting to note that LBPs were able to activate microglial cells, the brain macrophages, as reported by Chang and So (2008). A few studies demonstrate that LBP can upregulate humoral immune responses. It has been found that LBPs enhance in vitro antibody production by splenocytes from normal mice and senescence-accelerated mice (Qi, Zhang et al. 2001). In addition, LBP given orally to mice resulted in an increase in antigen (SRBC)-specific humoral response (Luo, Yan, and Zhang 1999).
14.5.2. Influence of Glycan Composition And Protein Content of Lycium Barbarum Polysaccharide
Preparations and fractions of LBP isolated from L. barbarum were different for different groups, and this might explain the differences in the immunostimulatory effects observed. The format of linkage between different carbohydrates or among carbohydrates and proteins, and the protein content of LBP seem to determine the biological activity of LBPs. Four homogeneous preparations of LBP—LBP 1a-1, LBP 1a-2, LBP 3a-1, and LBP 3a-2—enhanced the proliferation of mouse spleen cells induced by ConA (Duan et al. 2001). The LBPs with a main chain of α-(1→4)-D-polygalacturonans (i.e., LBP 3a-1 and LBP 3a-2) demonstrated a stronger immunomodulation activity than the LBPs with α-(1→6)-D-glycans (i.e., LBP 1a-1 and LBP 1a-2).
The role of the carbohydrate was further investigated by Peng, Huang et al. (2001). Five polysaccharide components (LBP1-LBP5) were purified from the crude LBP and from some of them (i.e., LBP1, LBP3, LBP4, and LBP5) the glycoconjugates (i.e., LbGp1, LbGp3, LbGp4, and LbGp5B) and their glycan chains (i.e., LbGp1-OL, LbGp3-OL, and LbGp4-OL) were obtained. The glycoconjugate LbGp5B was found to enhance mouse spleen cell proliferation whether ConA or LPS was used or not (Peng, Qi et al. 2001). The glycoconjugates LbGp1, LbGp3, and LbGp4 and their glycan chains LbGp1-OL, LbGp3-OL, and LbGp4-OL were found to enhance mouse spleen cell proliferation (Qi, Huang et al. 2001). The effects of glycan chains were stronger than those of respective conjugates, suggesting that the glycan chains may be the important active structure of carbohydrate conjugates. The homogeneous glycoconjugate LbGp4 and its purified glycan LbGp4-OL were both found to enhance the proliferation of mouse spleen cells in the absence of mitogen (Huang et al. 2001; Peng, Huang et al. 2001). Interestingly, it has been demonstrated that although they induced in vitro the proliferation of B lymphocytes whether LPS was used or not, they had no effect on the proliferation of T lymphocytes in the presence or absence of ConA. From binding assays, it was suggested that a receptor-binding site exists on B cells for those arabinogalactans. In addition, it was demonstrated that the immunostimulatory effect of LbGp4 was associated with activation of the expression of the nuclear factor κB (NF-κB) and the activator protein-I (AP-I; Peng, Huang et al. 2001).
Among other preparations of homogeneous fractions of LBP, fractions LBPF4 and LBPF5 were reported to increase the proliferation of mouse spleen T cells, but not B cells (Chen, Kwong Huat Tan, and Chan 2008). The other three fractions, LBPF1, LBPF2, and LBPF3, were unable to activate the T cells. It was suggested that this could be due to the higher protein content of LBPF4 and LBPF5 compared to LBPF1, LBPF2, and LBPF3. This was supported by the finding that the T cell stimulatory effect of LBPF4 and LBPF5 was significantly compromised when the proteins were digested (Chen, Kwong Huat Tan, and Chan 2008). T cell activation induced by LBP was further investigated, and LBPF4 and LBPF5, as well as crude LBP, were shown to (1) activate two transcription factors NFAT and AP-1, which play important roles in T cell activation; (2) enhance the expression of the T cell activation marker CD25; and (3) induce cytokine gene transcription and protein secretion of IL-2 (an essential cytokine for T cell growth) and interferon-γ (IFN-γ, a cytokine promoting the differentiation of Th-1 cells and, therefore, a predominantly cell-based immune response; Chen, Kwong Huat Tan, and Chan 2008). Consistent with this last observation, exposure of aged T cells to the glycopeptide LbGp3 resulted in increased IFN-γ expression and decreased IL-10 expression (Yuan et al. 2008). It has been reported that LBP3p dose-dependently increased the expression of IL-2 and TNF-α at the levels of mRNA and protein in cultured human peripheral blood mononuclear cells (PBMCs; Gan et al. 2003). These LBP-induced changes in IL-2, IFN-γ, TNF-α, and IL-10 cytokine expression suggest a transition from Th-2 cells (producing IL-4, IL-5, and IL-10) to Th-1 cells (producing IL-2, IFN-γ, and TNF-α). Since during aging there is a shift toward a more pronounced Th-2-type immune response (Rink, Cakman, and Kirchner 1998), these effects of LBP on cytokine production (Gan et al. 2003; Chen, Kwong Huat Tan, and Chan 2008; Yuan et al. 2008) support an antiaging property of wolfberry.
14.5.3. Indirect Immune Benefits of Lycium Barbarum Polysaccharide
The immunomodulatory effects of wolfberry can also be evaluated through its antitumor property. Indeed, the antitumor capacity of wolfberry can be attributed to the stimulatory effects of LBP on immune cells. In a clinical trial, patients suffering from advanced cancer treated with lymphocyteactivated killer (LAK) cells and IL-2 combined with oral administration of LBP (1.7 mg/kg/day) showed a significantly higher regression of cancer and a more marked increase in natural killer cell activity than patients treated with LAK/IL-2 alone (Cao, Yang, and Du 1994), suggesting that LBP may be useful as an adjuvant for the treatment of cancer.
The antitumor effect of LBP and the mechanisms involved have been further examined in different preclinical models. In a brain G422 tumor-bearing mouse model, it was reported that LBP combined with irradiation and 1,3 bis-(2-chloroethyl)-1-nitrosourea (BCNU) not only increased the life span of mice but also improved cellular immune function (Sun 1994). Oral administration of LBP (LBP3p at 10 mg/kg for 10 days) in S180-bearing mice was found to significantly inhibit the growth of the transplantable sarcoma S180 and to improve macrophage phagocytosis, spleen cell proliferation, the activity of cytotoxic T lymphocytes, and the expression of IL-2 mRNA (Gan et al. 2004). In H22 hepatoma-bearing mice, it has been suggested that the antitumor effect of LBP given orally could be mediated by increased CD4+ and CD8+ T cell numbers in tumor-infiltrating lymphocytes (He et al. 2005). In rodent models of irradiation- and chemotherapy-induced myelosuppression, subcutaneous injection of LBP (50–200 mg/kg) promoted the recovery of PBMCs (Gong et al. 2005). Since it was reported that LBP stimulated the production of granulocyte-macrophage colony-stimulating factor (GM-CSF, a major factor that regulates hematopoiesis) by human PBMCs in vitro, it was suggested that the therapeutic effects of LBP in those models resulted from the stimulation of PBMCs to produce GM-CSF (Gong et al. 2005).
14.6. ANTIDIABETIC ACTIVITY OF WOLFBERRY
A review of the official list of TCM herbs used for treating diabetes in China (Jia, Gao, and Tang 2003) does not specially mention wolfberry; nevertheless, its use for preventing and alleviating diabetes can be traced back to the sixteenth century. In ancient times, TCM doctors called patients showing symptoms related today to diabetes xiao ke
, which literally means “leanness and thirst.” It is known today that many xiao ke patients have abnormal blood and urine sugar levels. In the TCM treatise Compendium of Materia Medica
, wolfberry has been recorded for the treatment of xiao ke, indicating its potential application in treating diabetes. With this historical background, the traditional benefits of wolfberry alone or in combination with other herbs in relation to diabetes have been investigated in scientific and clinical studies in China (Bai 1998), and there are numerous reports of studies, largely using animal models, that have demonstrated positive effects of wolfberry in relation to diabetes. For example, therapeutic effects on diabetes and related complications, such as improved hemorrheology markers (e.g., plasma viscosity, hematocrit, and equation k value of erythrocyte sedimentation rate) of diabetes mellitus and diabetic nephropathy, were reported on patients (Li, Pen, and Zhang 1999; Liu et al. 1995; Li, Ma, and Liu 2007).
A water extract of wolfberry showed a significant hypoglycemic effect in adrenalin-induced and alloxan-induced diabetic mouse models and improved sugar tolerance (Tan 2008). In another study, such an extract increased blood insulin levels and helped to improve the function and recovery of impaired β-cells of pancreatic islets (Tian and Wang 2005). Wolfberry was also found to reduce serum total cholesterol (TC) and triglyceride (TG) concentrations and, at the same time, to markedly increase high-density lipoprotein cholesterol (HDL-C) levels in hyperlipidemic or diabetic rabbits (Luo et al. 2004). LBPs have been identified as one of the most active ingredients related to wolfberry’s biological activities. For instance, LBPs remarkably lowered blood glucose in alloxaninduced diabetic rabbit and mouse models, apparently due to the cytoprotective effect of LBPs on β-cells of pancreatic islets (Luo et al. 1997; Wang et al. 1999). In the streptozotocin-induced diabetic rat model, LBPs were found to improve abnormal oxidative indices, protecting liver and kidney tissue (Li 2007). Treatment with LBP significantly lowered the levels of fasting blood glucose, NO, malondialdehyde (MDA), and NO synthase (NOS) activity in a streptozotocin-induced rat diabetes model group, whereas the level of fasting insulin, functional index of β cells, and SOD activity were significantly increased in the LBP group, suggesting that the effect of LBP on blood glucose and β cells might be related to an increase in pancreatic islet SOD activity and a decrease in NOS activity (Huang et al. 2006). LBP was also found to decrease cellular DNA damage in peripheral lymphocytes of non-insulin-dependent diabetes mellitus (NIDDM) rats, possibly via a decrease in oxidative stress levels (Wu, Guo, and Zhao 2006). LBP can alleviate insulin resistance, which is associated with increasing cell-surface level of glucose transporter 4 (GLUT 4) trafficking and intracellular insulin signaling in NIDDM rats (Zhao, Li, and Xiao 2005).
These findings indicate that wolfberry may have therapeutic effects for treating diabetes comparable to chemical drugs without causing significant side effects during short- and long-term treatment of diabetic complications. However, comparison data supporting this hypothesis have not been reported so far.
14.7. CARDIOVASCULAR BENEFITS OF WOLFBERRY
Over the last decade, some research investigations have demonstrated the cardiovascular protective potential of wolfberry or its extracts. Among other mechanisms, effects on oxidative stress may underlie the therapeutic promise for the cardiovascular benefit of wolfberry, as seen for in vitro and in vivo experimental settings. Such effects are likely related to the modulation of both neuronal- and endothelial-dependent NO pathways by wolfberry.
The NO pathway (particularly, the classical endothelial-dependent pathway) is one of the most important mechanisms for maintaining the functional and structural integrity of the vasculature and the heart (Figure 14.9). Inhibition of the pathway, especially by endogenous NOS inhibitors and reactive oxygen species (ROS; e.g., superoxide anions and hydrogen peroxide), has physiological and pathological consequences, including blood pressure elevation, thrombosis, and atherosclerosis. NO is constitutively produced by both neuronal and endothelial NOSs (i.e., nNOs and eNOs) during the conversion of the NOS amino acid substrate L-arginine to L-citrulline. Centrally, one putative notion is that neuronal NO produced in the cardiovascular control center of the autonomic nervous system is part of a signal transduction pathway that tonically restrains sympathetic outflow generated from the rostral brainstem, leading to decreased adrenergic tone in the arteries and the heart. Peripherally, endothelial-dependent NO production and its vascular dilatory action in the adjacent vascular smooth muscle cells have been established over the last two decades to be the predominant mechanisms in modulating vascular function (Thomas, Zhang, and Victor 2001; Augustyniak et al. 2005). It is reported that wolfberry contains L-arginine (Gross, Zhang, and Zhang 2006), which is a substrate for NOS (Figure 14.9). More importantly, and as noted in Section 14.3.3, wolfberry is also rich in a precursor of ascorbic acid, 2-O-(β-D-glucopyranosyl) ascorbic acid, which is very stable and detectable in the bloodstream of both artery and portal vein after oral administration (Toyoda-Ono et al. 2004) and in zeaxanthin (Breithaupt et al. 2004; Cheng et al. 2005; Benzie et al. 2006). Both the vitamin C precursor and zeaxanthin scavenge and inactivate ROS. Moreover, wolfberry is reported to increase serum levels of the antioxidant enzyme SOD and glutathione peroxidase (GPx; Amagase, Sun, and Borek 2009). Working together, these compounds can increase both the synthesis and the bioavailability of constitutive NO in the cardiovascular system directly and decrease sympathetic discharge to the peripheral organs. Because NO deficiency is involved in the pathogenesis of disorders such as hypertension and atherosclerosis, enhancing the activity of the NO pathway or increasing NO bioavailability constitutes a way of promoting cardiovascular health or lowering cardiovascular risk. In one of the rare human studies on wolfberry, 25 independently living subjects aged 64–80 years who took 50 g/ day of wolfberry fruit for 10 days showed significantly increased blood SOD and hemoglobin (by 48% and 12%, respectively), and a 65% decrease in lipid peroxides (Li et al. 1991).
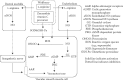
FIGURE 14.9
The NO pathway (particularly the classical endothelial-dependent pathway) plays a very important role in maintaining the functional and structural integrity of the vasculature and the heart. Wolfberry may promote cardiovascular health by inhibiting reactive (more...)
In rats that underwent two-kidney, one-clip surgery (a classical model of activated renin angiotensin system with ROS overexpression; Zhao et al. 2006), high blood pressure developed after 20–25 days. Treatment with 10% LBP (at a dose of 0.5 mL/100 g body weight) from wolfberry that was started two days postsurgery prevented the development of hypertension. Aortic rings from rats treated with LBP showed a significantly lower vasoconstrictive response in response to phenylephrine (PE) compared to aortic rings from control animals, whereas the vasodilatory response to acetylcholine (ACh), which represents the function of endothelium, was significantly increased in rats treated with LBP compared to control animals (Jia et al. 1998). In cultured cardiomyocytes, blocking L-type calcium channels glycopeptides from wolfberry lowered intracellular free calcium concentrations stimulated by hypoxia and KCl (Xu, Huang, and Tian 2005). However, in a randomized, double-blind, placebo-controlled clinical trial of 16 healthy subjects who took 120 mL of wolfberry juice for 14 days, no changes in cardiovascular parameters, that is, blood pressure and heart rate, were seen, although these subjects reported increased ratings for energy level, athletic performance, quality of sleep, ease of awakening, ability to focus on activities, mental acuity, calmness, feelings of health, and reduced fatigue and stress (Amagase and Nance 2008). Clinical investigations of wolfberry in patients with cardiovascular disorders are scant, and further study is warranted.
The protective effects of wolfberry have been seen also in other models of oxidative stress to the cardiovascular system. For example, during chemotherapy with the anticancer drug doxorubicin, ROS are generated by the drug and damage cardiomyocytes, causing myofibrillar loss and cytoplasmic vacuolization. This oxidative stress related to doxorubicin cardiotoxicity is accompanied by prolonged QT and ST intervals and an elevated ST segment, leading to arrhythmia and mortality. In a rodent study, treatment with wolfberry extract (at 25 mg/kg, per oral administration) effectively improved myocardial lesions and cardiac function and lowered mortality by 13% in doxorubicintreated animals, without affecting the anticancer property of doxorubicin (Xin et al. 2007). In rabbits fed a high-fat diet for 8 weeks, which induces aortic atherosclerosis accompanied by increased plasma cholesterol and triglycerides, decreased HDL-C, and increased oxidative stress and levels of inflammatory cytokines, treatment with an oil extract of wolfberry seeds was found to upregulate SOD and GPx and downregulate NF-κB, and TNF-α levels. The potency of the antiatherosclerotic effect of this wolfberry extract was reported to be comparable to that of lovastatin (Jiang et al. 2007).
14.8. IMPROVEMENT OF SEXUAL FUNCTION BY WOLFBERRY
Wolfberry has been used traditionally to enhance sexual function, and there is some scientific evidence for such a claim. In cultured seminiferous epithelium, ultraviolet light-induced lipid peroxidation was inhibited by LBP (Wang et al. 2002). This could indicate an antioxidative ability of LBP; however, ultraviolet exposure does not represent a direct causal factor for declined sex function or fertility. To this effect, hyperthermia is an important risk factor, as seminiferous epithelium is very sensitive to heat. A finding of interest is that hyperthermia-induced structural and functional damage was largely prevented by LBP (Wang et al. 2002; Luo et al. 2006) in vivo. Moreover, free radical-induced cytochrome c reduction and H2O2-induced DNA oxidative damage in seminiferous epithelial cells were also inhibited by polysaccharides derived from wolfberry (Wang et al. 2002; Luo et al. 2006). In vivo experiments repeated the effect of LBP on heat-induced functional and structural damage to the testis (Luo et al. 2006). Furthermore, in rats with unilateral castration, LBP improved copulatory performance and reproductive function, such as shortened penis erection latency and mount latency, increased sexual hormone levels, augmented accessory sexual organ weights, and improved sperm quantity and quality (Luo et al. 2006).
As far as the mechanism is concerned, improvement in sexual function by wolfberry is largely linked to its effect on the NO-cGMP axis by providing more substrate for NO synthesis and scavenging ROS (Figure 14.9). In a different experiment, an herbal formulation containing wolfberry seeds enhanced intracavernous pressure and NO-cGMP activity in the penile tissues of male rats (Sohn et al. 2008). Thus, the mechanism of sexual function improvement is unlike that of other erectile enhancement drugs such as sidlenafil, which are potent and selective inhibitors of cGMP-specific phosphodiesterase (PDE) type 5 (PDE5). Wolfberry is suggested to independently augment NO-cGMP bioavailability via PDE.
14.9. COGNITIVE BENEFIT OF WOLFBERRY
Traditionally, wolfberry was not particularly considered for preventing loss of cognitive function in an aged population; but this may be due to fact that our average life span did not exceed 50 years until the early twentieth century (Zhang and Zhang 2009). Amyloid-beta (Aβ or Abeta) is a peptide of 39–43 amino acids that, by increasing the activity of caspase-3 and lactate dehydrogenase (Anfuso, Lupo, and Alberghina 1999; Ho et al. 2007; Yu et al. 2007), causes neuronal apoptosis (neurotoxicity) and is suggested to be the main component of amyloid plaques in cerebral vasculature and in Alzheimer’s disease associated with aging. Extract of wolfberry prevented double-stranded RNA-dependent protein kinase (PKR) from being phosphorylated by Abeta, thereby inhibiting caspase-3 and lactate dehydrogenase (Yu et al. 2005, 2007).
A recent investigation also demonstrated the beneficial effects of a milk-based wolfberry preparation (WP) on cognitive dysfunction in a rat model of prenatal stress (Feng et al. 2010). It was found that physical restraint induced mental stress in pregnant rats, which caused a significant decrease in cognitive function in the female offspring. When the pregnant mothers were pretreated with WP, the prenatal stress-induced cognitive dysfunction in their offspring was significantly decreased. Mechanistic experiments showed that WP scavenged hydroxyl and superoxide radicals (determined by an electron spin resonance spectrometric assay) in neuronal tissue. Furthermore, FeCl2/ascorbic acid-induced dysfunction in brain mitochondria as characterized by increases in ROS and lipid peroxidation and decreases in the activities of complexes I and II, as well as decreases in glutamate cysteine ligase in vitro, were inhibited by WP (Feng et al. 2010).
These promising in vitro and animal findings raise the possibility of formulating a wolfberrybased therapy for preventing neurodegenerative disease in the elderly and for protecting early brain development in the neonate.
14.10. VISION BENEFITS OF WOLFBERRY
The consumption of wolfberry has been related for centuries by Chinese doctors and consumers to a perceived benefit to vision (Cheng et al. 2004; Potterat 2010). Despite a strong belief in the benefits of wolfberry on eyesight, very few human studies of wolfberry supplementation on visual parameters have been reported.
A 15-day regimen of wolfberry juice supplementation had no effect on visual acuity in healthy young adults (Amagase and Nance 2008). Age-related macular degeneration (AMD) is a primary cause of vision loss in the elderly, negatively impacts quality of life, and raises the risk of clinical depression, hip fractures, and placement in nursing homes (Mangione et al. 1999; Wysong, Lee, and Sloan 2009). The prevalence of AMD ranges from 1.5% in people over 40 years to over 15% in women over 80 years (Friedman et al. 2004). Unfortunately, there is no cure for AMD, and current treatment options have limited effectiveness and introduce significant patient risk (Kaufman 2009). Therefore, AMD prevention strategies should be identified and implemented.
Wolfberry is the richest natural source known of zeaxanthin, and the uptake and increase of plasma zeaxanthin concentration upon supplementation has been demonstrated (Cheng et al. 2005). However, there is little direct evidence to support the idea that consumption of wolfberry-derived zeaxanthin can subsequently increase macular pigment optical density, that is, concentrations of macular lutein and zeaxanthin, and ultimately lower AMD risk (Beatty et al. 2001; Carpentier, Knaus, and Suh 2009). It was reported for AMD subjects that lutein and zeaxanthin concentrations are lower by about 32% in three concentric regions centered in the fovea (Bernstein et al. 2002), and a positive association was found between serum concentrations of lutein and zeaxanthin and the macular pigment density (Bone et al. 2000; Gale et al. 2003). The only supplementation study with wolfberry zeaxanthin that showed such efficacy was conducted with rhesus monkeys (Leung et al. 2001). The serum and tissue levels of zeaxanthin and lutein were studied after feeding the animals an extract of wolfberry for 6 weeks as a daily supplement (equivalent of 2.2 mg zeaxanthin/day). The serum levels and macular density of zeaxanthin increased over the duration of the study, and were found to be significantly higher than those of the control monkeys. A similarly clear increase was observed in a very small human supplementation study (with two subjects and administration of 30 mg of synthetic zeaxanthin per day for 4 months; Bone et al. 2003).
Regular consumption of wolfberry may play a role in the prevention and/or stabilization of AMD and maintain macular pigment optical density (Bone et al. 2003). However, long-term clinical studies with a minimum of 4 months of supplementation will be needed to ascertain if wolfberry has a direct or indirect effect on vision in the elderly.
14.11. CONCLUSION
Wolfberry fruit has a remarkable history and a positive image in Chinese medicine and culture. Modern science indicates that its benefits, long known to the Chinese, are indeed the result of the presence and combination of several biologically active molecules. First, there is the widely studied LBP, a group of complex arabinogalactan proteins. Our insight indicates that the biological relevance of LBP may be limited as there is doubt that the identified structure and content of wolfberry LBPs are very different from those known from other plants. The preparation and analysis of LBPs are very complex; thus, it remains to be seen whether the potential benefits and efficacy of LBPs can be demonstrated in human trials. The unusual vitamin C precursor named 2-O-(β-D-glucopyranosyl) ascorbic acid and the carotenoid zeaxanthin, an essential component of the human macula, were discovered and subjected to more study recently. Their potential benefits are partially known, but only long-term supplementation studies with defined, clear outcomes will tell us more about their true benefits and mode of action. Having developed a holistic approach, similar to that used in TCM, by using the emulsifying properties of skim milk, we were able to develop a new formulation of wolfberry fruit that combines for the first time in one preparation all the bioactives from wolfberry, not only the water-soluble bioactives (as in TCM) but also the equally important fat-soluble ones such as zeaxanthin.
REFERENCES
- Amagase H, Nance D.M. A randomized, double-blind, placebo-controlled, clinical study of the general effects of a standardized Lycium barbarum (goji) juice, GoChi. J Altern Complement Med. 2008;14:403–12. [PubMed: 18447631]
- Amagase H, Sun B, Borek C. Lycium barbarum (goji) juice improves in vivo antioxidant biomarkers in serum of healthy adults. Nutr Res. 2009;29:19–25. [PubMed: 19185773]
- Anfuso C.D, Lupo G, Alberghina M. Amyloid beta but not bradykinin induces phosphatidylcholine hydrolysis in immortalized rat brain endothelial cells. Neurosci Lett. 1999;271:151–4. [PubMed: 10507691]
- Augustyniak R.A, Thomas G.D, Victor R.G, Zhang W. Nitric oxide pathway as new drug targets for refractory hypertension. Curr Pharm Des. 2005;11:3307–15. [PubMed: 16250858]
- Bai S. Research on Ningxia Wolfberry (Lycium barbar?m). Vol. 1 and 2. Yinchuan, Ningxia, China: Ningxia People's Publishing House.; 1998.
- Banchereau J, Steinman R.M. Dendritic cells and the control of immunity. Nature. 1998;392:245–52. [PubMed: 9521319]
- Beatty S, Murray I.J, Henson D.B, Carden D, Koh H, Boulton M.E. Macular pigment and risk for age-related macular degeneration in subjects from a northern European population. Invest Ophthalmol Vis Sci. 2001;42:439–46. [PubMed: 11157880]
- Benzie I.F.F, Chung W.Y, Wang J, Richelle M, Bucheli P. Enhanced bioavailability of zeaxanthin in a milk-based formulationof wolfberry (Gou Qi Zi; Fructus barbarum L.). Brit J Nut. 2006;96:154–60. [PubMed: 16870004]
- Bernstein P.S, Zhao D.Y, Wintch S.W, Ermakov I.V, McClane R.W, Gellermann W. Resonance Raman measurement of macular carotenoids in normal subjects and in age-related macular degeneration patients. Ophthalmology. 2002;109:1780–87. [PMC free article: PMC3079575] [PubMed: 12359594]
- Beuth J, Ko H.L, Oette K, Pulverer G. Inhibition of liver metastasis in mice blocking hepatocyte lectins with arabinogalactan infusions and D-galactose. J Cancer Res Clin Oncol. 1987;113:51–5. [PubMed: 3818778]
- Bone R.A, Landrum J.T, Dixon Z, Chen Y, Llerena C.M. Lutein and zeaxanthin in the eyes, serum and diet of human subjects. Experiment Eye Res. 2000;71:239–45. [PubMed: 10973733]
- Bone R.A, Landrum J.T, Guerra L.H, Ruiz C.A. Lutein and zeaxanthin dietary supplements raise macular pigment density and serum concentrations of these carotenoids in humans. J Nutr. 2003;133:992–8. [PubMed: 12672909]
- Borel P, Grolier P, Armand M, editors. Carotenoids in biological emulsions: solubility, surface-to-core distribution, and release from lipid droplets. J. Lipid Res. 1996;37:250–261. [PubMed: 9026524]
- Breithaupt D.E, Weller P, Wolters M, Hahn A. Comparison of plasma responses human subjects after the ingestion of 3R,3R– -zeaxanthin dipalmitate from wolfberry (Lycium barbarum) and non-esterified 3R,3R' -zeaxanthin using chiral high-performance liquid chromatography. Brit J Nutr. 2004;91:707–13. [PubMed: 15137922]
- Cao G, Yang W, Du P. Observation of the effects of LAK/IL-2 therapy combining with Lycium barbarium polysaccharides in the treatment of 75 cancer patients. Chin J Oncol. 1994;16:428–31. [PubMed: 7720497]
- Chang R.C.C, So K.F. Use of anti-aging herbal medicine, Lycium barbarum, against aging- associated diseases. What do we know so far? Cell Molec Neurobiol. 2008;28:643–52. [PubMed: 17710531]
- Chao S, Schreuder M, Young G, Nakaoka K, Moyes L, Oberg C. Pre-clinical study: Antioxidant levels and immunomodulatory effects of wolfberry juice and other juice mixtures in mice. Jana. 2004;7:2–8.
- Carpentier S, Knaus M, Suh M. Associations between lutein, zeaxanthin, and age-related macular degeneration: An overview. Crit Rev Food Sci Nutr. 2009;49:313–26. [PubMed: 19234943]
- Chen Z, Kwong Huat Tan B, Chan S.H. Activation of T lymphocytes by polysaccharide-protein complex from Lycium barbarum L. Int Immunopharmacol. 2008;8:1663–71. [PubMed: 18755300]
- Cheng C.Y, Chung W.Y, Szeto Y.T, Benzie I.F.F. Fasting plasma zeaxanthin response to Fructus barbarum L. (wolfberry; Kei Tze) in a food-based human supplementation trial. Brit J Nutr. 2005;93:123–30. [PubMed: 15705234]
- Cheng J, Lee P, Li J, Dennehy C.E, Tsourounis C. Use of Chinese herbal products in Oakland and San Francisco Chinatowns. Am J Health-Syst Pharm. 2004;61:688–94. [PubMed: 15119575]
- Chitchumroonchokchai C, Failla M.L. Hydrolysis of zeaxanthin esters by carboxyl ester lipase during digestion facilitates micellarization and uptake of the xanthophyll by Caco-2 human intestinal cells. J Nutr. 2006;136:588–94. [PubMed: 16484529]
- D'Adamo P. Larch arabinogalactan is a novel immune modulator. J Naturopath Med. 1996;4:32–9.
- Deng H.B, Cui D.P, Jiang J.M, Cai N.S, Li D.D. Inhibiting effects of Achyranthes bidentata polysaccharide and Lycium barbarum polysaccharide on nonenzyme glycation in D-galactose induced mouse aging model. Biomed Environ Sci. 2003;16:267–75. [PubMed: 14631832]
- Du G, Liu L, Fang J. Experimental study on the enhancement of murine splenic lymphocyte pro-liferation by Lycium barbarum glycopeptide. J Huazhong Univ Sci Technol Med Sci. 2004;24:518–21. [PubMed: 15641709]
- Duan C.L, Qiao S.Y, Wang N.L, Zhao Y.M, Qi C.H, Yao X.S. Studies on the active polysac-charides from Lycium barbarum L. Yaoxue Xuebao. 2001;36:196–9. [PubMed: 12580087]
- During A, Dawson H.D, Harrison E.H. Carotenoid transport in decreased and expression of the lipid transporters SR-BI,NPC1L1, and ABCA1 is downregulated in Caco-2 cells treated with Ezetimibe. J Nutr. 2005;135:2305–12. [PubMed: 16177187]
- El-Sohemy A, Baylin A, Kabagambe E, Ascherio A, Spiegelman D, Campos H. Individual carotenoid concentrations in adipose tissue and plasma as biomarkers of dietary intake. Am J Clin Nutr. 2002;76:172–9. [PubMed: 12081831]
- Feng Z, Jia H, Li X, editors. A milk-based wolfberry preparation prevents prenatal stress-induced cogni-tiveimpairment of offspring rats, and inhibits oxidative damage and mitochondrial dysfunction in vitro. Neurochem Res. 2010;35:702–11. [PubMed: 20131093]
- Friedman D.S, O'Colmain B.J, MuÓoz B, editors. et al. Prevalence of age-related macular degeneration in the United States. Arch Ophthalmol. 2004;122:564–72. [PubMed: 15078675]
- Gale C.R, Hall N.F, Phillips D.I.W, Martyn C.N. Lutein and zeaxanthin status and risk of agerelated macular degeneration. Invest Ophthalmol Vis Sci. 2003;44:2461–65. [PubMed: 12766044]
- Gan L, Zhang S.H, Liu Q, Xu H.B. A polysaccharide-protein complex from Lycium barba-rumupregulates cytokine expression in human peripheral blood mononuclear cells. Eur J Pharmacol. 2003;471:217–22. [PubMed: 12826241]
- Gan L, Zhang S.H, Yang X.L, Xu H.B. Immunomodulation and antitumor activity by a polysaccharide-protein complex from Lycium barbarum. Internat Immunopharmacol. 2004;4:563–9. [PubMed: 15099534]
- Gau G.W, Yang W.G, Du P. Observation of the effects of Lycium barbarum polysaccharides (LBP) in combination with LAK/IL-2 therapy in the treatment of 75 cancer patients. Chin J Oncol. 1994;16:1190–7. [PubMed: 7720497]
- Geng C.S, Xing S.T, Zhou J.H, Chu B.M. Enhancing effect of Lycium barbarum polysaccharides on the interleukin-2-activity in mice. Chin J Pharmacol Toxicol. 1989;3:175–9.
- Goldbohm R.A, Brants H.A.M, Hulshof K.F.A.M, Van den Brandt P. The contribution of various foods to intake of vitamin A and carotenoids in the Netherlands. Int J Vit Nutr Res. 1998;68:378–83. [PubMed: 9857265]
- Gong H, Shen P, Jin L, Xing C, Tang F. Therapeutic effects of Lycium barbarum polysaccha- ride (LBP) onirradiation or chemotherapy-induced myelosuppressive mice. Cancer Biother Radiopharm. 2005;20:155–62. [PubMed: 15869449]
- Granado F, Olmedilla B, Gil-Martinez E, Blanco I, Millan I, Rojas-Hidalgo E. Carotenoids, retinol and tocopherols in patientswith insulin-dependent diabetes mellitus and their immediate relatives. Clin Sci. 1998;94:189–95. [PubMed: 9536928]
- Gross P.M, Zhang X, Zhang R. Wolfberry: Nature's Bounty of Nutrition & Health. Charleston, SC: Booksurge Publishing.; 2006.
- Hagman B, Ryd W, Skomedal H. Arabinogalactan blockade of experimental metastases to liver by murine hepatoma. Invasion Metastasis. 1991;11:348–55. [PubMed: 1822847]
- Hartmann D, Thürmann P.A, Spitzer V, Schalch W, Manner B, Cohn W. Plasma kinetics of zeaxanthin and 3'- dehydro-lutein after multiple oral doses of synthetic zeaxanthin. Am J Clin Nutr. 2004;7:410–7. [PubMed: 14985215]
- He Y.L, Ying Y, Xu Y.L, Su J.F, Luo H, Wang H.F. Effects of Lycium barbarum polysaccharide on tumor microenvironment T-lymphocyte subsets and dendritic cells in H22-bearing mice. Journal of Chinese Integrative Medicine. 2005;3:374–7. [PubMed: 16159572]
- Ho Y.S, Yu M.S, Lai C.S, So K.F, Yuen W.H, Chang R.C. Characterizing the neuroprotective effects of alkaline extract of Lycium barbarum on beta-amyloid peptide neurotoxicity. Brain Res. 2007;1158:123–34. [PubMed: 17568570]
- Huang C, Chen Q.L, Sun J.T, Yang W.B, Ma L.J, Wan X.D. Protective effect of Lycium bar-barumpolysaccharide and its compound recipe on pancreatic islet function in rats with streptozotocin- induced diabetes mellitus. Chin J Clin Rehabilitat. 2006;10:173–5.
- Huang L.J, Lin Y, Tian G.Y, Ji G.Z. Isolation, purification and physico-chemical properties of immunoactive constituents from the fruit of Lycium barbarum L. Acta Pharma Sinica. 1998;33:512–6. [PubMed: 12016884]
- Huang L.J, Tian G.Y, Qi C.H, Zhang Y.X. Structure elucidation and immunoactivity studies of glycan of glycoconjugate LbGp4 isolated from the fruit of Lycium barbarum L. Kao Teng Hsueh Hsiao Hua Heush Hsueh Pao. 2001;22:407–11.
- Huang L.J, Tian G.Y, Zheng G. Structure elucidation of glycan of glycoconjugate LbGp3 isolated from the fruit of Lycium barbarum L. J Asian Nat Prod Res. 1999;1:259–67. [PubMed: 11523546]
- Inbaraj B.S, Lu H, Hung C.F, Wu W.B, Lin C.L, Chen B.H. Determination of carotenoids and their esters in fruits of Lycium barbarum L. by HPLC-DAD-APCI-MS. J Pharmaceut Biomed Anal. 2008;47:812–8. [PubMed: 18486400]
- Jermyn M.A, Yeow Y.M. A class of lectins present in the tissues of seed plants. Aust J Plant Physiol. 1975;2:501–31.
- Jia Y.X, Dong J.W, Wu X.X, Ma T.M, Shi A.Y. The effect of Lycium barbarum polysaccharide on vascular tension in two-kidney, one clip model of hypertension. Sheng Li Xue Bao. 1998;50:309–14. [PubMed: 11324572]
- Jia W, Gao W.Y, Tang L. Anti-diabetic herbal drugs officially approved in China. Phytother Res. 2003;17:127–34. [PubMed: 14669243]
- Jiang Y.D, Cao J, Dong Q.Z, Wang S.R. Experimental study of anti- atherosclerosis potency by lycium seed oil and its possible mechanism. Zhong Yao Cai. 2007;30:672–7. [PubMed: 17918438]
- Kaufman S.R. Developments in age-related macular degeneration, diagnosis and treatment. Geriatrics. 2009;64:16–9. [PubMed: 19351219]
- Lam K.W, But P. The content of zeaxanthin in Gou Qi Zi, a potential health benefit to improve visual acuity. Food Chem. 1999;67:173–6.
- Landrum J.T, Bone R.A. Lutein, zeaxanthin, and the macular pigment. Arch Biochem Biophy. 2001;385:38–40. [PubMed: 11361022]
- Lanzavecchia A, Sallusto F. Regulation of T cell immunity by dendritic cells. Cell. 2001;106:263–6. [PubMed: 11509174]
- Leung I.Y.F, Tso M.O.M., Li W.W.Y, Lam T.T. Absorption and tissue distribution of zeaxanthin and lutein in rhesus monkeys after taking Fructus lycii (Qou Qi Zi) extract. Invest Ophthalmol Vis Sci. 2001;42:466–71. [PubMed: 11157884]
- Li X.M. Protective effect of Lycium barbarum polysaccharides on streptozotocin-induced oxidative stress in rats. Internat J Biol Macromolec. 2007;40:461–5. [PubMed: 17166579]
- Li W, Dai S.Z, Ma W, Gao L. Effects of oral administration of Wolfberry on blood superoxide dismutase (SOD), hemoglobin (Hb) and lipid peroxide (LPO) levels in old people. Chin Trad Herb Drugs. 1991;22:251–68.
- Li X.M, Ma Y.L, Liu X.J. Effect of the Lycium barbarum polysaccharides on age related oxidative stress in aged mice. J Ethnopharmacol. 2007;111:504–11. [PubMed: 17224253]
- Li Z, Pen G, Zhang S. Composition and content of carotenoids in Fructus lycii. J Plant Resources Environm. 1999;8:57–8. (China)
- Li H.Y, Peng L, Wang L. Comparison of trace elements and total flavone contentin Chinese wolf-berry in different regions. Stud Trace Ele Health. 2007;24:14–6.
- Li Y.J, Qi C.H, Zhao X.N, Cheng J.P, Wei C.H, Zhou W.X, Zhang Y.X. Effects of glyco-conjugate and its glycan isolated from Lycium barbarum L on macrophage function. Chin Pharmacol Bull. 2005;21:1304–8.
- Li D.K, Zhang J. Influence of Yishen decoction on hemorheological indexes in patients with dia-betesmellitus and diabetic nephropathy. J Clin Rehabil Tissue Eng Res. 2007;11:5854–6.
- Li Q, Zhang G.J, Feng R, Fu X.H, Mao J.C, Wang Y. Clinical research of diabetic retinopathy treated with Tang-an-kang. J Chengdu Univ Trad Chin Med. 1999;1:23–6.
- Li X.L, Zhou A.G. Evaluation of the antioxidant effects of polysaccharides extracted from Lycium barbarum. Medicinal Chem Res. 2007;15:471–82.
- Liu W.J, Liu H.L, Ji Y, Li Y.Y. The hypoglycemic effect of Jiangtang Huoxue capsule. Chin J Exper Trad Med Formula. 1995;3:50–1.
- Liu C.Y, Tseng A. Chinese Herbal Medicine. Modern Applications of Traditional Formulas. Boca Raton, FL: CRC Press; 2005.
- Luo Q, Cai Y, Yan J, Sun M, Corke H. Hypoglycemic and hypolipidemic effects and antioxidant activity of fruit extracts from Lycium barbarum. Life Sci. 2004;76:137–49. [PubMed: 15519360]
- Luo Q, Li Z, Huang X, Yan J, Cai Y.Z. Lycium barbarum polysaccharides: Protective effects against heat induced damage of rat testes and H2O2-induced DNA in mouse testicular cells and beneficial effect on sexual behaviour and reproductive function of hemicastrated rats. Life Sci. 2006;79:613–21. [PubMed: 16563441]
- Luo Q, Li Z.N, Yang M.L, Yan J, Cui X.Y, Jiang M. Effects of Lycium barbarum polysaccharides on human prostate carcinoma PC-3 cells and its anti-tumor effect. Acta Nutrimenta Sinica. 2008;30:78–81.
- Luo Q, Li J.W, Zhang S.H. Effect of Lycium barbarum polysaccharides-X on reducing blood glucose in diabetic rabbits. Chin J Trophology. 1997;19:173–7.
- Luo Q, Yan J, Li J, Zhang S. Effect of Lycium barbarum L. and its polysaccharides on decreasing serum lipids in rabbits. Acta Nutrimenta Sinica. 1997;19:415–7.
- Luo Q, Yan J, Li J, Zhang S. The comparative study on the antifatigue effects of crude and pure Lycium barbarum polysaccharides. Acta Nutrimenta Sinica. 1999;21:310–7.
- Luo Q, Yan J, Zhang S. Effects of pure and crude Lycium barbarum polysaccharides on immunopharmacology. Zhong Yao Cai. 1999;22:246–9. [PubMed: 12575077]
- Ma M, Lui G, Yu Z, Chen G, Zhang X. Effect of the Lycium barbarum polysaccharides administration on blood lipid metabolism and oxidative stress of mice fed high-fat diet in vivo. Food Chem. 2009;113:872–7.
- Ma W.P, Ni Z.J, Li H, Chen M. Changes of the main carotenoid pigment contents during the dryingprocesses of the different harvest stage fruits of Lycium barbarum L. Agric Sci. 2008;7(3):363–9.
- Maeda M, Nakao M, Fukami H. 2-O-(β-D-Glucopyranosyl)ascorbic acid, process for its production, and foods and cosmetics containing compositions comprising it. 2003. Patent WO03/057707.
- Mangione C.M, Gutierrez P.R, Lowe G, Orav E.J, Seddon J.M. Influence of age-related maculopathy on visual functioning and health-related quality of life. Am J Ophthalmol. 1999;128:45–53. [PubMed: 10482093]
- National Commission of Chinese Pharmacopoeia. 2005. Pharmacopoeia of People's Republic of China, Chemical Industry Press, Beijing, China. English version.
- Niu A.J, Wu J.M, Yu D.H, Wang R. Protective effect of Lycium barbarum polysaccharides on oxidative damage in skelmuscle of exhaustive exercise rats. Internat J Biol Macromolec. 2008;42:447–9. [PubMed: 18405964]
- Peng X.M, Huang L.J, Qi C.H, Zhang Y.K, Tian G.Y. Studies on chemistry and immuno- modulating mechanism of a glycoconjugate from Lycium barbarum L. Chinese J Chem. 2001;19:1190–7.
- Peng X.M, Qi C.H, Tian G.Y, Zhang Y.X. Physico-chemical properties and bioactivities of a glycoconjugate LbGp5B from Lycium barbarum L. Chin J Chem. 2001;19:842–6.
- Peng X.M, Wang Z.F, Tian G.Y. Physico-chemical properties and activity of glycoconjugate LbGp2 from Lycium barbarum L. Yaoxue Xuebao. 2001;36:601–2. [PubMed: 12579937]
- Pèrez-Gálvez A, Minguez-Mosquera M.I. Degradation, under non-oxygen-mediated autooxidation, of carotenoid profilepresent in paprika oleoresins with lipid substrates of different fatty acid com-position. J Agric Food Chem. 2004;52:632–7. [PubMed: 14759160]
- Potterat O. Goji (Lycium barbarum and L. chinense): Phytochemistry, pharmacology and safety in the perspective of traditional usesand recent popularity. Planta Med. 2010;76:7–19. [PubMed: 19844860]
- Potterat O, Hamburger M. Goji juice: A novel miraculous cure for longevity and well-being? A review of composition, pharmacology, health-related claims and benefits. Schweiz Zeitschr Ganzheits Medizin. 2008;20:399–405.
- Qi C.H, Huang L.J, Zhang Y.X, Zhao X.N, Tian G.Y, Ru X.B, Shen B.F. Chemical structure and immunoactivity of the glycoconjugates and their glycan chains from the fruit of Lycium barbarum L. Chin J Pharmacol Toxicol. 2001;15:185–90.
- Qi C.H, Zhang Y.X, Zhao X.N, editors. et al. Immunoactivity of the crude polysaccharides from the fruit of Lycium barbarum L. Chin J Pharmacol Toxicol. 2001;15:180–4.
- Reboul E, Abou L, Mikail C, editors. et al. Lutein transport by Caco-2 TC-7 cells occurs partly by a facilitated process involving the scavenger receptor class B type (SR-BI). Biochem J. 2005;15:455–61. [PMC free article: PMC1134974] [PubMed: 15554873]
- Redgwell R.J, Fischer M. Dietary fibre as a versatile food component: An industrial perspective. Mol Nutr Food Res. 2005;49:421–535. [PubMed: 15926144]
- Rink L, Cakman I, Kirchner H. Altered cytokine production in the elderly. Mech Ageing Dev. 1998;102:199–209. [PubMed: 9720652]
- Sesso H.D, Buring J.E, Christen W.G, editors. et al. Vitamin E and C in the prevention of cardiovascular disease in men. The Physicians' Health Study II randomized controlled trial. JAMA. 2008;300:2123–33. [PMC free article: PMC2586922] [PubMed: 18997197]
- Sohn D.W, Kim H.Y, Kim S.D, editors. et al. Elevation of intracavernous pressure and NO-cGMP activity by a new herbal formula in penile tissues of spontaneous hypertensive male rats. J Ethnopharmacol. 2008;120:176–80. [PubMed: 18762238]
- Sommerburg O, Keunen J.E.E, Bird A.C, van Kuijk F.J.G.M. Fruits and vegetables that are sources for lutein and zeaxanthin: The macular pigment in human eyes. Brit J Ophthalmol. 1998;82:907–10. [PMC free article: PMC1722697] [PubMed: 9828775]
- Sun W.J. Therapeutic effect of Lycium barbarum polysaccharides in combination with irradiation and BCNU in brain G422 tumour-bearing mice. Chin J Clin Oncol. 1994;21:930–2.
- Sze S.C.W, Song J, Chang R.C.C, Zhang K.Y, Wong R.N.S, Tong Y. Research advances on the anti-aging profile of Fructus lycii: An ancient Chinese herbal medicine. J Comp Int Med. 2008;5:1–17.
- Tan S.M. Study on the hypoglycemic effect of wolfberry. J Southern Medical Univ. 2008;28:2103–4.
- Thomas G.D, Zhang W, Victor R.G. Nitric oxide deficiency as a cause of clinical hypertension: Promising new drug targets for refractory hypertension. JAMA. 2001;285:2055–7. [PubMed: 11311074]
- Thomè O.W. 1885. Flora von Deutschland, Osterreich und der Schweiz. Gera, Germany.
- Tian L.M, Wang M. The hypoglycemic effect and pancreatic tissue histomorphology study of wolfberry. Trad Chin Med J. 2005;4:48–51.
- Toyoda-Ono Y, Maeda M, Nakao M, Yoshimura M, Sugiura-Tomimori N, Fukami H. 2-O-(β-D- Glucopyranosyl)ascorbic acid, a novel ascorbic acid analogue isolated from lycium fruit. J Agric Food Chem. 2004;52:2092–6. [PubMed: 15053557]
- Tucker K.L, Chen H, Vogel S, Wilson P.W.F, Schaefer E.J, Lammi-Keefe C.J. Carotenoid intakes, assessed by dietary questionnaire, are associated with plasma carotenoid concentrations in an elderly population. J Nutr. 1999;129:438–45. [PubMed: 10024624]
- Tyssandier V, Lyan B, Borel P. Main factors governing the transfer of carotenoids from emulsion lipid droplets to micelles. Biochimica et Biophysica Acta. 2001;1533:285–292. [PubMed: 11731338]
- Van Holst G.J, Clarke A.E. Quantification of AGP in plant extracts by single radial gel diffusion. Anal Biochem. 1985;148:446–50. [PubMed: 3933380]
- Wang Z.Y. Study on active component from the fruit of Lycium barbarum in different regions. Bullet Botan Res. 2003;23:337–9.
- Wang J.K, Bertholet R, Watzke H, Ducret P, Bucheli P. Delivery of functional ingredients. 2005. Patent WO2005092121 A2.
- Wang L, Dong J, Jiang L.Z, editors. et al. The effects of LBP-D, hypoglycemic agents, alone or in combination, on blood glucose and immune functions in alloxan-induced diabetes mice. J Yunnan Univ. 1999;21:186–88. (Natural Sciences Edition)
- Wang B.K, Xing S.T, Zhou J.H. Effect of Lycium barbarum polysaccharides on the immune responsesof T, CTL and NK cells in normal and cyclophosphamide-treated mice. Chin J Pharmacol Toxicol. 1990;4:39–43.
- Wang Y, Zhao H, Sheng X, Gambino P.E, Costello B, Bojanowski K. Protective effect of Fructus lycii polysaccharides against time and hyperthermia-induced damage in cultured seminiferous epithe-lium. J Ethnopharmacol. 2002;82:169–75. [PubMed: 12241992]
- Weller P, Breithaupt D.E. Identification and quantification of zeaxanthin esters in plants using liquid chromatography-mass spectrometry. J Agric Food Chem. 2003;51:7044–9. [PubMed: 14611169]
- Wu H, Guo H, Zhao R. Effect of Lycium barbarum polysaccharide on the improvement of antioxi-dantability and DNA damage in NIDDM rats. Yakugaku Zasshi. 2006;126:365–71. [PubMed: 16679745]
- Wu Z.Y, Raven P.H. Flora of China. Vol. 17. St. Louis: Science Press, Beijing, and Missouri Botanical Garden Press; 1994. 2005.
- Wysong A, Lee P, Sloan F. Longitudinal incidence of adverse outcomes of age-related macular degeneration. Arch Ophthalmol. 2009;127:320–7. [PMC free article: PMC3621973] [PubMed: 19273797]
- Xin Y.F, Zhou G.L, Deng Z.Y, editors. et al. Protective effect of Lycium barbarum on doxorubicin-induced cardiotoxicity. Phytother Res. 2007;21:1020–4. [PubMed: 17622973]
- Xu S.L, Huang J, Tian G.Y. Effects of LbGp on the intracellular free calcium concentration of cardiomyocytes induced by hypoxia and KCl. Zhongguo Zhong Yao Za Zhi. 2005;30:534–8. [PubMed: 16011102]
- Yeum K.J, Booth S.L, Sadowski J.A, editors. et al. Human plasma carotenoid response to the ingestion of controlled diets high in fruits and vegetables. Am J Clin Nutr. 1996;64:594–602. [PubMed: 8839505]
- Yin G, Dang Y. Optimisation of extraction technology of the Lycium barbarum polysaccharides by Box-Behnken statistical design. Carbohydr Polymers. 2008;44:603–10.
- Yu M.S, Lai C.S, Ho Y.S, editors. Characterization of the effects of anti-aging medicine Fructus lycii on beta-amyloid peptide neurotoxicity. Int J Mol Med. 2007;20:261–8. [PubMed: 17611646]
- Yu M.S, Leung S.K, Lai S.W, editors. et al. Neuroprotective effects of anti-aging oriental medicine Lycium barbarum against beta-amyloid peptide neurotoxicity. Exp Gerontol. 2005;40:716–27. [PubMed: 16139464]
- Yu D.H, Wu J.M, Niu A.J. Health promoting effect of LBP and healthy Qigong exercise on physiological functions in old subjects. Carbohydr Polym. 2009;75:312–6.
- Yuan L.G, Deng H.B, Chen L.H, Li D.D, He Q.Y. Reversal of apoptotic resistance by Lycium barbarum glycopeptide 3 in aged T cells. Biomed Environ Sci. 2008;21:212–7. [PubMed: 18714818]
- Zhang M, Chen H. Effects of a glycoconjugate from Lycium barbarum on body composition in growing mice. J Sci Food Agric. 2006;86:932–6.
- Zhang M, Chen H, Huang J, Zhong L, Zhu C, Zhang S. Effect of Lycium barbarum polysac-charide on human heptoma QGY7703 cells: Inhibition of proliferation and induction of apoptosis. Life Sci. 2005;76:2115–24. [PubMed: 15826878]
- Zhang M, Zhang S.H. Study on structure of Lycium barbarum L. polysaccharide. Food Res Devel. 2007;28:74–7.
- Zhang G.Q, Zhang W. Heart rate, lifespan, and mortality risk. Ageing Res. 2009;8:52–60. [PubMed: 19022405]
- Pharmacological study on water extract of wolfberry. In: Zhao C, editor; Bai S, editor. In Research on Ningxia Wolfberry (Lycium barbarum). Vol. 1. Ningxia People's Publishing House; Yinchuan, China: 1998. p. 604.
- Zhao Z. An Illustrated Chinese Materia Medica in Hong Kong. School of Chinese Medicine, Hong Kong Baptist University, Chung Hwa Book Co. Ltd; Hong Kong, China: 2004. p. 127.
- Zhao H, Alexeev A, Chang E, Greenburg G, Bojanowski K. Lycium barbarum glycoconjugates: Effect on human skin and cultured dermal fibroblasts. Phytomedicine (Jena). 2005;12:131–7. [PubMed: 15693720]
- Zhao R, Li Q, Xiao B. Effect of Lycium barbarum polysaccharide on the improvement of insulin resistance in NIDDM rats. Yakugaku Zasshi. 2005;125:981–8. [PubMed: 16327243]
- Zhao W, Swanson S.A, Ye J, editors. et al. Reactive oxygen species impair sympathetic vasoregulation in skelmuscle in angiotensin II-dependent hypertension. Hypertension. 2006;48:637–43. [PubMed: 16940212]
- Zhou L, Leung I, Tso M.O.M, Lam K.W. The identification of dipalmityl zeaxanthin as the major carotenoid in Gou Qi Zi by high pressure liquid chromatography and mass spectrometry. J Ocular Pharmacol Therapeut. 1999;15:557–65. [PubMed: 10609778]
- Zhou X.L, Sun P.N, Bucheli P, Huang T.H, Wang D.F. FT-IR methodology for quality control of arabinogalactan protein (AGP) extracted from green tea (Camellia sinensis). J Agric Food Chem. 2009;57:5121–8. [PubMed: 19456132]
- Zhu J, Zhao L.H, Chen Z. Stimulation by Lycium barbarum polysaccharides of the maturation of dendritic cells in murine bone marrow. J Zhejiang University Medical Sciences. 2006;35:648–52. [PubMed: 17177338]
- Zhu J, Zhao L.H, Zhao X.P, Chen Z. Lycium barbarum polysaccharides regulate phenotypic and functional maturation of murine dendritic cells. Cell Biol Int. 2007;31:615–9. [PubMed: 17289406]
- INTRODUCTION
- DESCRIPTION AND TRADITIONAL USE OF WOLFBERRY FRUIT
- IDENTIFICATION OF BIOACTIVES
- BIOAVAILABILITY OF WOLFBERRY BIOACTIVES AND EFFECTS OF PROCESSING
- IMMUNOMODULATING ACTIVITY
- ANTIDIABETIC ACTIVITY OF WOLFBERRY
- CARDIOVASCULAR BENEFITS OF WOLFBERRY
- IMPROVEMENT OF SEXUAL FUNCTION BY WOLFBERRY
- COGNITIVE BENEFIT OF WOLFBERRY
- VISION BENEFITS OF WOLFBERRY
- CONCLUSION
- REFERENCES
- Review Health benefits of wolfberry (Gou Qi Zi, Fructus barbarum L.) on the basis of ancient Chineseherbalism and Western modern medicine.[Avicenna J Phytomed. 2021]Review Health benefits of wolfberry (Gou Qi Zi, Fructus barbarum L.) on the basis of ancient Chineseherbalism and Western modern medicine.Wenli S, Shahrajabian MH, Qi C. Avicenna J Phytomed. 2021 Mar-Apr; 11(2):109-119.
- Immunomodulatory effects of dietary supplementation with a milk-based wolfberry formulation in healthy elderly: a randomized, double-blind, placebo-controlled trial.[Rejuvenation Res. 2012]Immunomodulatory effects of dietary supplementation with a milk-based wolfberry formulation in healthy elderly: a randomized, double-blind, placebo-controlled trial.Vidal K, Bucheli P, Gao Q, Moulin J, Shen LS, Wang J, Blum S, Benyacoub J. Rejuvenation Res. 2012 Feb; 15(1):89-97.
- Review The treatment of rheumatoid arthritis using Chinese medicinal plants: From pharmacology to potential molecular mechanisms.[J Ethnopharmacol. 2015]Review The treatment of rheumatoid arthritis using Chinese medicinal plants: From pharmacology to potential molecular mechanisms.Lü S, Wang Q, Li G, Sun S, Guo Y, Kuang H. J Ethnopharmacol. 2015 Dec 24; 176:177-206. Epub 2015 Oct 22.
- Up-regulation of crystallins is involved in the neuroprotective effect of wolfberry on survival of retinal ganglion cells in rat ocular hypertension model.[J Cell Biochem. 2010]Up-regulation of crystallins is involved in the neuroprotective effect of wolfberry on survival of retinal ganglion cells in rat ocular hypertension model.Chiu K, Zhou Y, Yeung SC, Lok CK, Chan OO, Chang RC, So KF, Chiu JF. J Cell Biochem. 2010 May 15; 110(2):311-20.
- [A bibliometric overview of traditional Chinese medicine research in Medline].[Zhong Xi Yi Jie He Xue Bao. 2010][A bibliometric overview of traditional Chinese medicine research in Medline].Fu JY. Zhong Xi Yi Jie He Xue Bao. 2010 Apr; 8(4):379-84.
- Biomolecular and Clinical Aspects of Chinese Wolfberry - Herbal MedicineBiomolecular and Clinical Aspects of Chinese Wolfberry - Herbal Medicine
- MIR3677HG MIR3677 and MIR940 host gene [Homo sapiens]MIR3677HG MIR3677 and MIR940 host gene [Homo sapiens]Gene ID:106660606Gene
- SAB2413c [Staphylococcus aureus RF122]SAB2413c [Staphylococcus aureus RF122]Gene ID:3794186Gene
- Tbc1d32 TBC1 domain family, member 32 [Mus musculus]Tbc1d32 TBC1 domain family, member 32 [Mus musculus]Gene ID:544696Gene
Your browsing activity is empty.
Activity recording is turned off.
See more...