NCBI Bookshelf. A service of the National Library of Medicine, National Institutes of Health.
Oral Health in America: Advances and Challenges [Internet]. Bethesda (MD): National Institute of Dental and Craniofacial Research(US); 2021 Dec.
Chapter 1. Current Knowledge, Practices, and Perspectives
We are living in a period of transformational change in which information abounds and choices are often daunting. Advances in science and technology are driving the evolution of health care practices. However, although new scientific tools are being used to identify previously unknown mechanisms of disease and enable a fresh view of previously held beliefs, new technology and state-of-the-art scientific approaches must be adapted in a way that is well thought out, evidence-based, and available and accessible to all.
Tackling the challenge of achieving optimal health requires innovations from basic research, their translation into clinical treatments, and their effective implementation across highly diverse populations. Research takes a variety of forms including basic, translational, clinical, implementation, and health services and policy research. Both discovery and translation are needed to address the spectrum of conditions that affect the oral cavity: dental caries and periodontal disease, craniofacial diseases and disorders, oral and oropharyngeal cancers, pulpitis, salivary gland dysfunction, temporomandibular joint dysfunction, mucosal disorders, and so many others. To achieve these goals, basic science, technologic innovation, and implementation science require supported systems; a dedicated, diverse, and highly educated workforce; and attention to sustainability during the revision and reevaluation of existing knowledge.
Foundational Sciences in Oral and Craniofacial Health
Foundational sciences in oral and craniofacial health encompass basic biological and physical sciences; population, social, and behavioral sciences; and ethics, all of which provide the foundation for clinical practice. A major advance in foundational science knowledge occurred in 2003, with the completion of the Human Genome Project. The Human Genome Project sequenced the entire human genome, thereby accelerating gene mapping for complex traits that comprise oral and craniofacial conditions. The ability to analyze different cell populations at the single-cell level has furthered our understanding of variations among same cell types that can influence susceptibility to disease and treatment effectiveness. This increased understanding gives us the potential to analyze illnesses at the cellular and molecular level and to devise unique treatments designed to match individual genetic profiles within the context of environmental influences.
Omics, Gene Editing, and Single-Cell Technologies
Genomics
The Human Genome Project’s successful sequencing of the human genome made virtually all modern omics possible. The project’s offshoot, the Genome Reference Consortium (National Library of Medicine 2021a), periodically releases updated versions of the human reference genome. Publicly available DNA sequences are accessible to anyone online through GenBank®, a genetic sequence database (National Library of Medicine 2021b). Standardized genome annotation is available through the Reference Sequence collection (RefSeq), which provides a collection of reference sequences and genes against which individual variations can be compared (National Library of Medicine, 2021c)
A particularly fruitful application of these genomic tools has been genome-wide association studies (GWAS), analyses of complex human traits and diseases that allow investigators to focus on plausible areas of the genome harboring one or more risk genes for the trait of interest. Since the first successful genome-wide association study in 2005, thousands of GWAS have been carried out for hundreds of trait phenotypes (characteristics) (Buniello et al. 2019).
Other subtypes of genomics support functional elucidation (Hasin et al. 2017), clarifying how specific genetic variants lead to the phenotype of interest. These omics include transcriptomics, which evaluates RNA levels to determine expression of particular genes in particular tissues or at particular time points (see https://www.facebase.org/); proteomics, used to evaluate post-translational modification in genes’ protein products; metabolomics, which quantifies multiple small-molecule types (metabolites) in cells or tissues; and epigenomics, which focuses on characterization of reversible modifications to DNA or its proteins. To characterize the genetic component of periodontal disease pathogenesis, several groups have utilized GWAS with large patient cohorts and have identified genetic targets associated with disease (Offenbacher et al. 2016; Zhang et al. 2016).
Phenomics
Phenomics is the systematic measurement and analysis of high-dimensional phenotypic data on an organism-wide scale (Yong et al. 2014). The phenome is the set of phenotypes (including physical, biochemical, and psychological traits) produced by an organism during its life span in response to genetic, epigenetic, and environmental factors.
This relatively new discipline offers a unique perspective within which mechanisms of oral disease can be linked to clinical impact. For example, phenomics may support the investigation of complex craniofacial traits (such as cleft lip and/or palate and chronic orofacial pain) or craniofacial-systemic links, as well as dental anthropologic and forensic dentistry analyses. Deep phenotyping requires the integration of multiple micro and macro data sources, including dental and medical electronic health records (EHRs) and health claims, laboratory and imaging databases, national vital statistics records, national cohort studies, and pharmacy and emergency medical services data. Phenomics results will then support health care transformation through the implementation of precision dentistry and medicine for diverse patient populations (Denny and Collins 2021).
Single-Cell Omics
The concept of using single-cell resolution analyses in heterogeneous cell populations has become of great interest (Irish et al. 2006; Junker and van Oudenaarden 2014). Targeted measurements of single cells’ activities further the understanding of such cells’ variabilities and explain the differences among individuals in their susceptibilities to disease and reactions to treatment. To apply this technology, single cells are isolated, observed, and analyzed in detail. Scientists have developed diverse approaches for the isolation, trapping, and manipulation of single cells in a microfluidic device (Shapiro et al. 2013; Macaulay and Voet 2014; Lo and Yao 2015). Subsequent analyses have been applied at the single-cell level to measure the molecular signatures of different aspects and functions (Wang and Bodovitz 2010). Single-cell omics rapidly collect a substantial amount of data, which can be sorted into genomics, transcriptomics, epigenomics, proteomics, and metabolomics (Prakadan et al. 2017) and subjected to further analysis using DNA and RNA sequencing and other advanced technologies, such as chromatin immunoprecipitation, mass spectrometry, and nuclear magnetic resonance. These areas of single-cell omics provide exciting potential for precision cancer detection (including detection of head and neck cancer) at various stages of disease. Single-cell analyses also are useful for identifying cells for pharmaceutical targeting in cancer and oral conditions that affect the mucous membranes.
Microbiome/Inflammasome/Virology
The human microbiome encompasses the entire microbial community, including bacteria, fungi, protozoa, and viruses associated with humans (Baker et al. 2017). The microbial community has a rich and complicated genetic content (Fischbach 2018), making the microbiome an active player in human health and disease (Proctor et al. 2017).
The oral microbiome is a distinct and diverse group of microbes that inhabit the oral cavity’s hard and soft surfaces (Takahashi 2015). Studies among humans have uncovered its vital role in the two most prevalent oral infectious diseases—dental caries and periodontitis—as well as in a wide range of physiological and pathologic processes essential to overall health (Bowen et al. 2018; Lamont et al. 2018). The oral cavity harbors more microbes than any other part of the body except the gastrointestinal tract. Most of the microbes in the mouth live within biofilms (dental plaque) attached to soft (tongue, gingiva, and oral mucous membranes) and hard (tooth) tissue surfaces.
The mouth provides distinct habitats, including saliva, tongue, mucosal, and tooth surfaces, all colonized by site-specific microbial assemblages (Human Microbiome Project Consortium 2012a). The development of high-throughput DNA sequencing has allowed these distinct groupings to be characterized. Studies have sequenced aspects of genetic regions to obtain an overview of the oral microbiome according to oral health status (Griffen et al. 2012; Gross et al. 2012), presence of systemic disease (Diaz et al. 2013; Starr et al. 2018), environmental exposures and behavioral habits (Mason et al. 2015), heritability (Demmitt et al. 2017), and a variety of genetic defects (Abusleme et al. 2018). Studies in twins show that both heritable and environmental factors shape the oral microbiome (Gomez et al. 2017). Behaviors such as smoking (Mason et al. 2015), genetic mutations (Abusleme et al. 2018), and disease (Griffen et al. 2012; Imabayashi et al. 2016) affect the homeostatic (optimally healthy) balance of the oral microbiome and host tissues.
A web-based human oral microbiome database was established and then enlarged as the expanded Human Oral Microbiome Database (eHOMD) (Escapa et al. 2018). Along with another oral microbiome database, the CORE database (Griffen et al. 2011), eHOMD provides a valuable platform for oral microbiome studies. These tools enable clinically relevant studies to be conducted by identifying new species (Beall et al. 2018a), new metabolic pathways (Verma et al. 2018), or novel biosynthetic gene clusters (Medema et al. 2014) associated with health and disease. A comprehensive road map to oral microbial assembly has emerged, revealing the oral microbiome’s resilience to a variety of environmental challenges (Gomez and Nelson 2017).
Studies of the gut (gastrointestinal) microbiome also are relevant to oral health and have led to the concept of dysbiosis, which is broadly defined as shifts in the microbial community that feature a reduction in the species diversity of microbes, changes in metabolic and signaling activity, and adverse health consequences (Honda and Littman 2016). The increase in biomarker microbe species rather than the acquisition of new species, which is observed in both dental caries and periodontitis patients, supports a current model (the polymicrobial synergy and dysbiosis model) in which imbalances and altered metabolic pathways among microbes already present in the oral cavity cause these two diseases.
The interrelationship of the oral microbiome, gut microbiome, and systemic health has received considerable attention, with several investigations reporting an association between the oral microbiome and systemic diseases with an inflammatory component, such as rheumatoid arthritis (Potempa et al. 2017), colorectal cancer (Kostic et al. 2012), oral cancer (Hayes et al. 2018), and Alzheimer’s disease (Dominy et al. 2019).
Oral Microbiome Communities as Determinants of Oral Health and Disease
Several common oral diseases, including dental caries, periodontal diseases, and oropharyngeal candidiasis (fungal infection), are microbial in origin (Lalla et al. 2013; Lamont et al. 2018) and result from the interaction of microorganisms and host factors. Dental caries are a disease triggered by a microbial community in which interactions among various microbes play a role by modulating the net effect of dietary sugars and carbohydrates on tooth demineralization. Streptococcus mutans is the dominant microbial species in patients with dental caries (Gross et al. 2012). An intriguing association also has emerged between the fungus Candida albicans and early childhood caries (Xiao et al. 2018) and suggests that, like dental caries, periodontitis is a disease that results from the interaction of individuals’ microbial communities with the inherent and unique biological characteristics of their mouths. The development of periodontitis is linked to genetic and environmental determinants that modify an individual’s inflammatory response to the microbiome (Moutsopoulos et al. 2014; Offenbacher et al. 2018). Human clinical longitudinal (across the life span) studies and investigations in experimental animal models show that a dysbiotic microbiome community with an increase in certain microbial species, virulence (harmfulness) factors, and metabolic activities is required for the development of periodontitis (Dutzan et al. 2018).
The Oral Microbiome and Systemic Disease
Oral microorganisms can influence systemic diseases. Studies suggest that the microbiome in the oral cavity can colonize and participate in disease processes in other parts of the body that become systemic (Gao et al. 2018). For example, Porphyromonas gingivalis, a bacterium strongly associated with periodontitis, has been detected in the brain of patients with Alzheimer’s disease. An animal model showed the plausibility of P. gingivalis as a contributor to the disease development (Dominy et al. 2019). In addition, protein activities associated with a dysbiotic oral microbiome have been shown to mediate autoimmunity in rheumatoid arthritis (Potempa et al. 2017). These and other findings will require deeper investigation into disease mechanisms to help us better understand complex disease interactions that would differentiate between association and causality.
The Coexistence of Viral Infections and Bacterial Infections in the Oral Cavity
Periodontitis was once thought to be exclusively a bacteria-driven disease process, but it is now understood that viruses also are frequently detected in infected tissues (Slots 2015). These viruses include herpes simplex virus, human cytomegalovirus, Epstein-Barr virus, and Kaposi sarcoma-associated herpesvirus (Slots 2015). Human papillomavirus, a potentially cancer-causing oral pathogen, has been detected in gingival disease (Hormia et al. 2005), along with DNA tumor viruses associated with cancer development. Each of these viruses replicates in the oral epithelium and is shed into saliva. Their replication may result in oral transmission of the virus, as well as the development of viral lesions. In the case of DNA tumor viruses, viral gene expression has been associated with the development of oral cancers (Speicher et al. 2016). The periodontal pocket may serve as a reservoir for multiple viral infections that influence the establishment of periodontal disease (Rickinson 2014).
There also is a need to be keenly aware of a multitude of new infectious agents as well as old organisms that mutate or resurge. Severe acute respiratory syndrome (SARS) coronavirus rose to affect localized areas in the world at the turn of the 21st century, and Ebola raised fear more than a decade later. However, neither resulted in the damage seen from the surge of coronavirus disease 2019 (COVID-19) through an unprepared world in early 2020, with an unquestionable impact on human health on many levels. A pandemic such as COVID-19 has a profound impact and requires strong science combined with strategic public health measures to minimize the damage. From an emerging science perspective, we need to realize the importance of mobilizing science and technology to ensure the country’s health and prepare for the unknown. Knowledge gained from the COVID-19 pandemic is demonstrating this and is highlighting how the emergence of new infectious agents may have major ramifications for oral health that will unfold day by day, often requiring changes in our response.
Inflammation and the Inflammasome
Inflammation science has rapidly evolved and expanded across medical fields (Medzhitov 2010), including dental medicine. The function and structure of a network of proteins, called the inflammasome, directs the processing and release of key proinflammatory cytokines (signaling cells) (interleukin [IL]-1β and IL-18) and cell death (Martinon et al. 2002; Lamkanfi and Dixit 2017). Whereas acute inflammation is part of the body’s natural protective response to ensure the removal of harmful stimuli and promote tissue repair, chronic inflammation is a central component of many diseases (Majno and Joris 2004). Chronic inflammation (Majno 1998; Hotamisligil 2006) is essential to a number of metabolic, infectious, degenerative, autoimmune, and cancerous conditions (Darveau 2010). The pathophysiological entities that underlie most dental, oral, and craniofacial diseases involve inflammation as a direct or indirect disease mechanism (Van Dyke and Kornman 2008). Periodontal diseases, dental caries, root canal infections, mucosal infections, premalignant lesions, and head and neck cancers have in common a programmed inflammatory response that regulates their initiation and severity. In the context of the whole body, oral inflammation can significantly influence systemic events and vice versa (Joshipura et al. 2000; Engebretson and Kocher 2013).
A more precise picture of inflammation’s central role in this reciprocal relationship between oral health and overall health has emerged (Barnett 2006). Our bodies have a vast immune repertoire that can sense and respond to danger signals. Among the first genes activated by disease-causing microorganisms (Darveau 2010) are proinflammatory cytokines, such as IL-1 (Dinarello 2010). Distinct patterns of IL-1 family genes and other cytokine gene networks have been associated with unique inflammatory profiles in several complex diseases, including periodontitis, cardiovascular disease, rheumatoid arthritis, and osteoporosis, and during and following damage to dental pulp (Kornman 2006; Fouad et al. 2020).
Regenerative Medicine, Dental Materials, and Bioinspired Materials
Restoration and rehabilitation of hard and soft dental, mucosal, and craniofacial tissues to functional integrity require sophisticated orchestration integrating materials, cellular elements, mediators, and technology in a temporal and directed manner. The number of new technologies and biomaterials for restoring or replacing missing dental and other oral structures has surged. The availability of durable and highly aesthetic biomaterials has revolutionized the replacement of broken and severely decayed teeth. Nanotechnologies have transformed the design and manufacture of restorative and regenerative materials.
Biomaterials are substances, other than food or drugs, contained in therapeutic or diagnostic systems in contact with tissue or biological fluids (Peppas and Langer 1994). Dental biomaterials are used to restore and replace missing tooth structure, prevent further loss or damage to tooth structure and gum tissue, and replace missing teeth and other oral structures.
Materials primarily used in dentistry today are dental adhesives that bond restorative materials to tooth structure and create a seal around the restoration, resin-based dental composite materials that restore lost or damaged tooth structure, dental ceramic materials used as dental crowns and bridges to mimic natural tooth structure, and dental implants that replace the roots of lost teeth and support single or multiple tooth restorations. The materials most often used for restoring anterior and posterior teeth are adhesive-bonded dental composites. However, these materials do not last as long as dental amalgam fillings (Rasines Alcaraz et al. 2014) and often require replacement, resulting in significant costs to patients in terms of convenience, discomfort, and expense. Dental amalgam alloy is composed of elemental mercury, which binds to a mixture of metals. Studies funded by the National Institute of Dental and Craniofacial Research (NIDCR) have shown dental amalgam to be safe for human health, but for environmental reasons there is an international effort to phase down the use of dental amalgam, as articulated in the Minamata Convention. In 2020, the U.S. Food and Drug Administration (FDA) issued further communication recommending the use of non-mercury restorations (fillings), when possible and appropriate, for individuals in certain high risk groups (U.S. Food and Drug Administration 2020a). This provides another urgent reason for research into alternative restorative materials (Ajiboye et al. 2020). The primary reasons for replacement are recurrent tooth decay near the existing restoration and fracture of the restoration itself or the surrounding or supportive tooth structure (Demarco et al. 2012; Opdam et al. 2014). As a result, new materials, including biomaterials designed to interact with biological systems, are actively being sought.
Biomaterials
There are many examples of promising new technologies designed to produce better restorative materials that are more durable, stimulate the formation of new tooth-like mineral to repair defects and replace lost tooth structure, have improved aesthetics (better mirror the properties of enamel and dentin), support function (mirror the wear of natural tooth surfaces), repel or kill damaging bacteria to stop the formation of new cavities, and regenerate lost tooth components. Structural and mechanical functions are generally considered the biomaterials’ most valuable functions or properties. However, biological properties, such as biocompatibility and biodegradability, also are important.
Biomaterials are considered to be biocompatible if they are inert and cause no undesired negative effect on the body. In contrast, bioactive materials intentionally regulate biological functions of cells or tissue. Biomimetic materials imitate specific composition, structure, or characteristics of biological materials or systems. Bioinspired materials have specific advanced properties or functions inspired by a biological system. It is critical to remember that the safety of these new materials, not only for the patient, but also for the health care provider and the environment, must be studied.
A large portion of the biomaterials used today in dental or oral health treatment are biocompatible, inert materials. However, development of bioactive materials—such as bioceramics, bioglass, and biocomposite materials—used in dental and craniofacial bone repair and restoration has been increasing. The bioactive components in these biomaterials are mainly inorganic materials, including bioceramics (crystalline) and bioglass (noncrystalline). Calcium phosphates, such as hydroxyapatite and tricalcium phosphate, are widely used bioceramics with structures similar to the minerals in bone and teeth (Chu et al. 2001; Hench 2015) and have been made into porous scaffolds for bone and dental tissue regeneration (Hench and Polak 2002; Denry and Kuhn 2016). To overcome their shortcomings, many biocompatible materials have been made into composite materials with polymers to enhance processability and mechanical properties. Such bioactive composite materials are biomimetic (designed to mimic components of natural bone and dentin) and bioinspired (inspired by the structure of biological materials).
Nanotechnology
Nanotechnology is the control of materials at dimensions between 1 and 100 nanometers. It involves imaging, measuring, modeling, and manipulating matter at this scale. Applications of nanotechnologies in dentistry include the development of tools that enable probing and imaging of biological systems with high precision, as well as designing and manufacturing preventive and restorative dental biomaterials. As a result, dental biomaterials have become more bioinspired and biomimetic (Jefferies 2014), which enhances some of their properties in comparison to the same materials at larger scales. Nanomaterials are now a part of the majority of restorative dental composites (Ferracane 2011), remineralizing agents (Hannig and Hannig 2012), and antimicrobial systems (Cheng et al. 2017).
Biodegradable Polymers and Nanofibrous Scaffolds
Biodegradable polymers, such as polylactic acid, polyglycolic acid, and their copolymers (polylactic-co-glycolic acids) have good biocompatibility, controllable biodegradability, excellent processability, and desirable initial mechanical properties. They are widely used to fabricate highly porous scaffolds, but there are other ways to make nanofibrous materials. Electrospinning is a popular technique to generate nanofibrous materials from various polymers (Doshi and Reneker 1993; Boland et al. 2001). In addition to tissue-engineering scaffolds, biodegradable polymers can be used to fabricate microparticles or nanoparticles for controlled release of drugs or molecules. For example, antibiotics have been incorporated into dissolvable particles to treat periodontitis (Williams et al. 2001), and various kinds of extracellular matrixes have been used for soft-tissue regeneration around teeth (in tooth recession defects) or around dental implants in situations involving reduced tissue thickness or implant exposures (Tavelli et al. 2020).
Periodontal Regenerative Medicine
Clinicians are currently using techniques and products created for periodontal regeneration (Larsson et al. 2016). Guided tissue regeneration (GTR), the most well-documented technique, employs a barrier membrane to promote the selective repopulation of periodontal defects using tissues capable of reattaching, such as alveolar bone and periodontal ligament (Karring et al. 1993). Attempts also have been made to use bioactive molecules, such as enamel matrix derivative and platelet-derived growth factor, to promote periodontal regeneration (Giannobile and Somerman 2003; Nevins et al. 2013). Results have been comparable to those obtained with GTR, although complete regeneration remains elusive in the vast majority of clinical cases.
Orofacial Muscle Regenerative Medicine
The face is a highly organized mixture of elements, composed of an underlying bone and cartilage framework that supports muscle and subcutaneous and skin tissue (See Figure 1, Section 3A) (Susarla et al. 2011). These components are attached to one another with ligaments and interact dynamically within a neurovascular network that allows the face’s complex animation. Each subregion of the face constitutes an aesthetic unit, so that reconstruction has to be considered on the basis of the unit (Rose 1995; Mureau and Hofer 2009). Hence, reconstruction of the maxillofacial region is challenging. Current treatments of extensive craniomaxillofacial defects include static reconstruction, free vascularized muscle transfer (Tate and Tollefson 2006; Ghali et al. 2011), and composite tissue allografting (Siemionow 2017). Tissue-engineering strategies that employ inductive scaffolds or scaffolds combined with growth factors or cells have been broadly applied to generate functional skeletal muscle (Larkin et al. 2006; Passipieri and Christ 2016). Because of the greater inflammatory and fibrotic response associated with common degradable synthetic polyesters, the field has been focusing on materials of biological origin such as alginate, gelatin or collagen, and fibrin (Passipieri and Christ 2016). The loading of specific growth factors into scaffolds has been shown in multiple cases to induce the desired response of increased angiogenesis (new blood vessels), myogenesis (new muscle tissue), or neurogenesis (new nerve cells), or in some cases, a reduction of fibrosis (scar tissue) (Passipieri and Christ 2016). In summary, orofacial regenerative medicine holds great promise for improving patients’ health. Technologies in this area will reduce the number of procedures requiring autologous (self-transplant) donor tissue, which result in significant patient morbidity. They will provide less invasive and less costly treatments without donor harvest and with targeted (personalized) replacement constructs that can lead to more predictable treatments for patients.
Stem Cell Biology
Stem cells are undifferentiated cells in embryonic and adult tissues in the body that can self-renew and differentiate into different types of cells. Mesenchymal stem cells (MSCs) have been extensively studied in craniofacial regenerative applications involving stem cell therapy. The unique properties of MSCs—namely, their ability to differentiate into multiple cell types, produce bioactive factors involved in tissue regeneration, travel to distant sites of injury, and escape the body’s immune response—have made them a desirable population for stem cell therapies. MSC therapies currently are under preclinical and clinical investigation and are being administered to specific sites to replace damaged or deficient hard (bone, cartilage) and soft (gingiva, dental pulp) tissues, promote tissue neovascularization, or reduce inflammation (Polymeri et al. 2016). Because of their therapeutic potential, identification of MSCs from craniofacial and oral tissues has created excitement in the field of regenerative dentistry.
Science and Technology for Practice
Dentistry is at a pivotal point with regard to the technology used in practice and the potential for new scientific diagnostic and therapeutic approaches that can improve oral health. Computer-aided design/computer-aided manufacturing (CAD/CAM) as well as three-dimensional (3D) printing technologies have rapidly spread worldwide. Current dental technology and practice innovations are focused on precision health, laser and light imaging, digital dentistry, and pharmacologic approaches. Sensor-based technologies have been introduced to support oral health behaviors outside the clinic setting.
Genetics- and Genomics-Based Precision Medicine
Specific gene variations have been linked to more than 10,000 human diseases (World Health Organization 2020). Powerful research approaches and large, multidisciplinary research efforts are improving our understanding of the genetic basis of health and disease (Collins and Varmus 2015; Cheifet 2019). Defining diseases by their genetic and environmental drivers and identifying the biological pathways involved will profoundly affect disease classification as well as provide the ability to develop diagnostic tests, determine susceptibility, and create variant-specific treatment intervention strategies. Until recently, genetic information has been most useful in diagnosing relatively rare Mendelian genetic disorders, rather than parsing the complex genetic origins of more common diseases, such as heart disease, diabetes, and periodontitis.
Thousands of genetic diseases manifest symptoms in the oral cavity or craniofacial complex. Major clinical findings for a number of these conditions, such as amelogenesis imperfecta or isolated cleft palate, are found in the oral cavity. For others, such as dentinogenesis imperfecta and syndromic forms of orofacial clefting, symptoms may manifest both orally and in the vascular system, bones, and kidneys. In some conditions, dental symptoms are the most prominent; therefore, a dentist may be the first to recognize the need for further testing to confirm a genetic condition (Pallos et al. 2001).
Laser- and Light-Based Imaging Technologies
Dental carious lesions are routinely detected using visual methods coupled with radiography and, when needed, complemented with tactile exploration methods. Despite their common use, these methods are insufficiently sensitive or specific for diagnosing early noncavitated carious lesions. Radiographic methods lack the sensitivity to detect early dental decay, particularly occlusal lesions, and, by the time the lesions are radiolucent, they often have progressed well into the dentin, making surgical intervention necessary. If carious lesions are detected early enough, nonsurgical means such as fluoride therapy, antibacterial therapy, or dietary changes can reverse or arrest them.
Two different fluorescence-imaging systems have been introduced commercially. The first method, commonly called quantitative light fluorescence, uses blue or ultraviolet light with green fluorescence. The loss of fluorescence from the underlying sound tissue owing to increased light scattering by demineralization is the mechanism for increased contrast between the lesion and sound areas (Ten Bosch and Angmar-Mansson 1991). Fluorescence images provide increased contrast between sound and demineralized tooth structure, but the method is limited because stains and plaque fluoresce strongly and make detection difficult (Stookey 2005). A second approach relies on the red fluorescence from bacterial-produced porphyrins (Konig et al. 1999; Lussi et al. 2004). Porphyrins accumulate in highly porous subsurface lesion areas in dentin, causing hidden occlusal lesions to fluoresce (Lussi et al. 2004). However, this method has some drawbacks. Because the dental decay-causing bacterium S. mutans does not contain porphyrins, this approach is not an effective way of monitoring cariogenic bacteria. Moreover, it has poor sensitivity for lesions confined to enamel because porphyrins do not accumulate there (Shi et al. 2000). Several clinical devices that measure both red and green fluorescence currently are commercially available.
Digital Dentistry, Computer-Aided Design/Computer-Aided Manufacturing, and 3D Bioprinting
Dental medicine widely employs digital dental technology, including intraoral scanning, 3D imaging, reconstructive processing using CAD/CAM, and artificial intelligence (AI). This boom in technology in the areas of CAD/CAM and the expanded use of 3D bioprinting to create synthetic and natural dental tissues have made the production of dental restorations faster and simpler, and often less expensive.
CAD/CAM technologies for fabricating dental restorations have rapidly spread worldwide (van Noort 2012). To date, the most commonly used CAD/CAM methods in dentistry are subtractive, such as computer-controlled milling machines that drill a block of material to achieve a desired shape. Dentistry has profited greatly from this technology (Alghazzawi 2016), which makes it possible to create accurate restorations while saving time (Bindl and Mormann 2005). Using solid blocks of material results in fewer internal defects, which are common in handmade restorations and compromise their strength (Belli et al. 2017). Significant strides have been made in the field of 3D bioprinting—an additive manufacturing process—of regenerative scaffolds and cell-laden craniofacial structures, which are being developed as the next generation of bone, vascular, periodontal, and dental grafts (Murphy and Atala 2014).
Chemotherapeutics/Drugs in Dentistry
There are many types of medications, supplements, and other chemotherapeutics available in the marketplace that are promoted as preventing or managing dental diseases. However, the majority have not been approved by the FDA. Dental drugs that have been approved by FDA since the 2000 Surgeon General’s report include, but are not limited to, (in alphabetical order): Arestin, Articaine, Atridox, Colgate’s Total, Cuvposa, Evoxac, Oraqix, Oraverse, Kepivance, Kovanaze, Orabloc, PerioChip, Periostat and Salagen. FDA has determined a number of over-the-counter (OTC) drugs for oral health as generally recognized as safe and effective for the general population without the need to seek treatment from a health professional. The most common examples of OTC drugs employed in dentistry are those used to prevent dental caries, including sodium fluoride, stannous fluoride, and sodium monofluorophosphate. Dentists can dispense or provide products with higher levels of fluoride than those available OTC, as well as prescribe chlorhexidine as an intraoral antimicrobial rinse. Noninvasive diagnostic tools with improved sensitivity and specificity in detecting active dental caries lesions are needed, as are new drugs for treating periodontitis.
The National Center for Complementary and Integrative Health defines complementary and alternative medicine as non-mainstream approaches used together (complementary) or in place of (alternative) conventional medicine. These two approaches are brought together by integrative medicine, with the aim of caring for the whole person rather than just treating disease (National Center for Complementary and Integrative Health 2021). Most complementary and alternative medicine systems in oral health care are biologically based, such as botanical products and holistic medical systems such as (e.g., Ayurveda, homeopathy, Chinese medicine). Most complementary and alternative medicine systems have insufficient clinical evidence for their safety or efficacy (Shi and Heber 2013; Rigassio Radler 2014).
Information and Data Science
Data science is a growing field built on interdisciplinary research connecting subject-matter expertise in a particular domain with mathematical statistics and computer science (Figure 1). Areas within data science such as data modeling, machine learning, deep learning, big data, and AI have developed at a rapid pace during the last 2 decades, becoming important to health care and health informatics. By facilitating more accurate diagnosis and effective treatment, data science is important to evidence-based health care.
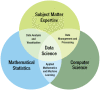
FIGURE 1
Data Science: The intersection of Subject Matter Expertise, Mathematical Statistics, and Computer Science.
Data modeling integrates the study of data and the use of oral health information by establishing relationships among data from different sources across broad patient populations. Using data modeling to store, retrieve, and analyze data for basic, translational, and clinical research studies makes clinical decision-making more effective and informative. Data modeling provides database structure diagrams upon which highly advanced computer applications can arrange complex information that characterizes oral diseases and conditions. In light of the increasing size, complexity, scope, locations, and sources of data, the National Institutes of Health (NIH) is exploring new models for data management resources, including partnerships with trusted organizations. To achieve this goal, NIH created a trusted partners federated data ecosystem for data storage and distribution of human genotype and phenotype data. This ecosystem includes, for example, BioData Catalyst, KidsFirst, AnVIL, and the Database for Genotypes and Phenotypes (dbGaP)(Office of Data Strategy, 2021). The National Library of Medicine provides support for registration and data access approval for the ecosystem.
Electronic Health Records
The implementation of EHRs is a strategy for reducing errors and improving health care delivery and outcomes (Song et al. 2011; Gold and McLaughlin 2016). Advances in the understanding of the connections between oral and total body systems and a shift toward individualized care have created a demand for an integrated medical and dental health care delivery system. The integrated medical and dental EHR has created new opportunities to exchange medical and dental information electronically, leverage resources, and improve patients’ oral and overall health care. EHRs have made it possible to develop an oral health care system that is better integrated with our country’s medical and behavioral health care systems (Acharya 2016; Faiola and Holden 2017; Shimpi et al. 2019a). Recognizing strategies to reduce the incidence of chronic diseases and improve the effectiveness and efficiency of care through the application of informatics is of paramount importance (Liu and Rubin 2012; Glurich et al. 2019; Shimpi et al. 2019b; Shimpi et al. 2019c). Unfortunately, an integrated EHR does not yet exist for most of the U.S. population.
Teledentistry
Telehealth includes telemedicine (the use of technology to deliver health care services at a distance) as well as patient and health professional education and public health and administrative activities (Daniel et al. 2015). Telemedicine has been introduced in rural and remote communities and in federal health programs to improve access to care. Various medical specialties and subspecialties in the United States and in other parts of the world now employ the technology supporting telehealth (Kvedar et al. 2014; Daniel et al. 2015). In addition to documented improvements in the flexibility of health services and high levels of acceptance among patients and providers, the benefits of telehealth include increased accessibility, improved quality of care, multidisciplinary collaboration, and education (Gilman and Stensland 2013; Banbury et al. 2014; Wade et al. 2016; Powell et al. 2017).
Although teledentistry has been slower to spread, it is now becoming more widely utilized across the country and in other parts of the world (Kopycka-Kedzierawski et al. 2008; Irving et al. 2018). The arrival of the COVID-19 pandemic has further fueled its use. See Section 4 for more information on the uses and practices of teledentistry.
Learning Health Systems
Efforts to create learning health systems (LHSs) at various levels of scale—organizations, networks of organizations, states and regions, and entire nations—promise to transform human health. The general idea is that a systems problem needs a systems solution. The National Academy of Medicine (National Academy of Engineering 2011) defines LHS as an integrated health system in which “progress in science, informatics, and care culture align to generate new knowledge as an ongoing, natural by-product of the care experience, and seamlessly refine and deliver best practices for continuous improvement in health and health care.”
The core building blocks of LHSs are learning cycles, which begin when communities share a common passion around a specific individual or population health problem and form a learning community targeting that problem. Learning communities typically consist of multiple stakeholders, including researchers, care providers, and patients and their families, and draw on expertise from diverse disciplines. From a national perspective, a large-scale learning community may consist of several entities (Figure 2).
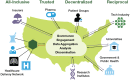
FIGURE 2
National perspective of an ultra-large scale learning health system.
Learning cycles proceed in three stages: capturing health experience in computable forms, learning from the data to generate new knowledge, and mobilizing new knowledge to inform decisions and change performance (Friedman et al. 2017). The right knowledge must be delivered to the right person, at the right time, in the right dose, through the right route, in the right form, with the right documentation, and for the right reason (Federico 2015). Each new cycle of learning begins where the previous one leaves off, making health improvement repeated and continuous in nature as exemplified in Figure 3.
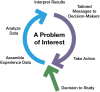
FIGURE 3
View from the ground: How learning happens: “Virtuous cycles” of study and change.
Implementation Science
Implementation science, the scientific study of methods to promote the systematic uptake of research findings and other evidence-based practices into routine care to improve the quality and effectiveness of health services (Eccles and Mittman 2006), is characterized as a series of phases ultimately leading to a change in practice and better health outcomes (Figure 4). These steps may take into account the individual patient, the complexities of health disparities, and a broad, community-level approach. An early example of an implementation science study in dental medicine, which focused on identifying effective interventions to increase providers’ implementation of dental sealants, demonstrated that a combination of evidence-based education and fee reimbursement most effectively facilitated the adoption of this new technology (Clarkson et al. 2008). This study illustrates an important aspect of implementation science research: The focus is not on identifying effective dental treatments, but on finding effective methods to increase providers’ implementation of them.

FIGURE 4
Implementation science: Turning discovery into health, benefiting all.
Evidence-Based Approaches to Introduce New Technologies into Clinical Applications
One important aspect of implementation science is evidence-based practice. Providers’ adoption of evidence-based dental practices is expanding due to the growing emphasis on improving population health, controlling cost of care, and delivering value for patients and care purchasers. Long lag times between the dissemination of evidence and its eventual adoption into routine practice limited the initial development of evidence-based practice in the 1990s. For example, even after compelling scientific evidence had accumulated in 2001 that fluoride varnish was superior to other modes of fluoride administration, 6 years later only 15.7% of general dentists and 30.8% of pediatric dentists in Texas reported that fluoride varnish was the topical fluoride they used most often (Bansal et al. 2012). Providers’ failure to change their practice patterns in response to scientific evidence demonstrates that scientific evidence alone is not sufficient to change these patterns.
Practice-Based Research Networks
Practice-based research networks (PBRNs) are an important component of NIDCR’s implementation science portfolio. A PBRN is a consortium of practitioners, clinical scientists, and health organizations focused collectively on conducting research to improve the health of their patients and communities. PBRNs link clinicians with health services and clinical researchers to improve the transfer of science to practice. This improvement is accomplished by fostering quality improvement through participation in research, addressing questions of high priority to the practitioners, and translating new knowledge into everyday clinical practice. Although medical PBRNs began in the 1970s, no oral health-focused PBRN existed in the United States before 2002. Because PBRNs can make substantial, unique improvements to clinical practice, they have continued to grow in number and breadth and now comprise a diverse array of health care practitioners.
PBRNs have as their foundation the understanding that the experience, insight, and practical wisdom of everyday practitioners and their patients, coupled with rigorous scientific studies, are powerful forces to advance population health. The oral health care system can play an active role in these advances, showing that knowledge transfer takes place not only from research to practice, but also from practice to research.
NIDCR funding has kept the National Dental PBRN in continuous operation since 2005 and has productively engaged thousands of clinicians in the research process.
Sixty studies have been conducted as of 2021, leading to more than 175 peer-reviewed scientific publications in 58 different journals, many with clinicians as coauthors. Examples of topics include risk factors for osteonecrosis of the jaw, dental material selection for crowns, and the use of rubber dams during root canal treatments. Practitioners from diverse settings are willing to engage in the excitement of discovery and to partner with fellow practitioners and academicians to improve daily clinical practice.
The National Dental PBRN also conducts studies of drug or device effectiveness as well as studies to test methods of evidence dissemination and implementation. The network continues to make significant contributions to research priorities and to help close the research-to-practice gap (Gilbert et al. 2013). In addition to exploring optimal means for disseminating evidence (Melkers et al. 2019), the network has conducted research to expand the scope of dental practice and to foster studies at the intersection of dentistry and medicine. These studies have been related to osteonecrosis of the jaw, cessation of tobacco use, blood glucose screening, human papillomavirus screening, opioid prescription patterns, and medical risk screening.
Research Workforce, Training, and Education
Studies suggest that dentists have the highest training costs of all health professionals in the United States and Canada (Gawel 2018). Skilled and effective dental faculty are essential to achieving the educational, patient-care, and research missions of dental schools and the profession (Haden et al. 2002). American dental schools face persistent faculty shortages (Kennedy 1995; Wanchek et al. 2016), worsened by the escalation in student debt, a widening income gap between private practitioners and dental faculty, and, at the time, the robust state of the U.S. economy (which favors private practice), all of which have had a negative effect on the oral health academic workforce (Wanchek et al. 2015).
The top two sources of dental faculty are advanced education programs and private practice. However, lack of exposure to and knowledge about academic careers hinders dental students in making informed decisions on this option (Schenkein and Best 2001; Rupp et al. 2006). A 2020 American Dental Education Association survey of dental school seniors from 59 of the 66 accredited U.S. dental schools found that only 0.3% had plans to enter academia (Istrate et al. 2021).
Foreign-trained dentists who have received advanced specialty training in the United States are an increasingly important source for filling vacant clinical faculty positions. They often have less student debt than U.S.-trained dentists, view faculty positions as prestigious, and can practice dentistry more easily as a faculty member than in the private sector. However, many lack research training and are unlikely to pursue independent research programs.
Students who have completed a Doctor of Philosophy (PhD) degree or combined Doctor of Dental Surgery (DDS)/Doctor of Dental Medicine degree and PhD training also are well suited for dental faculty positions. The number of U.S. dental schools increased from 54 to 66 during a 20-year period, but the number of oral health science PhD programs increased only from 16 to 17 over the same period. From 1994 to 2016, oral sciences PhD programs enrolled 33 new students on average per year, with 26 new graduates each year. Graduates were largely successful in their post-program pursuits. Many (35%) held faculty positions in U.S. academic institutions, 13% were faculty members in foreign institutions, 11% were employed by industry or government agencies, 12% worked in private practice, 12% were enrolled in postdoctoral training, and 11% were in residency or other advanced training (Herzog et al. 2018).
For many years, the general public viewed dental schools as entirely clinical entities, often with good reason. Eight of the nation’s dental schools established since 2010 are clinically focused, and a substantial number of dental schools historically have not emphasized support and recruitment of faculty members into active scientific research programs. Institutions with human capital and resources are likely to follow systematic approaches to preparing junior faculty for career success, whereas institutions with more limited resources tend to focus on their core educational mission rather than on building and retaining their faculty workforce. As a learned profession, oral health professionals have an obligation not only to generate new knowledge but to apply it to clinical care, and hence research is an integral component of a high-caliber dental education.
The single most influential organization for dental faculty workforce and oral health research is NIDCR, whose mission is to improve the nation’s dental, oral, and craniofacial health. To support and achieve this mission, NIDCR invests in the development of a strong and diverse workforce with the skills and knowledge to conduct oral health research across a broad range of disciplines and approaches, including new and emerging technologies. To build and sustain this workforce, NIDCR supports training opportunities at its campus in Bethesda, Maryland, and at multiple academic institutions across the United States. There are research training programs in basic, translational, clinical, and health services research for trainees from high school through established investigators.
Education and Training for Oral Health Scientists
Major advancements in knowledge in the oral health sciences have made it increasingly important to train the next generation of leaders on how to translate basic science innovations into clinical practice. Oral health scientists include PhD basic scientists, clinical research scientists, and those trained in health services and dental public health. Scientists who focus on oral health come from many diverse disciplines—sometimes with a clinical emphasis, but often without. All make essential contributions to the basic, applied, developmental, and implementation levels of research to inform clinical practice, population health management, and policy development as well as serve as educators of the workforce pipeline. Traditional dental education begins with an early focus on foundational and biological sciences and progresses to clinical focus in later years, a path that does not always lead to an appropriate level of exposure to population health issues. Integrating research experience into the predoctoral curriculum sets a foundation of critical thinking and inquiry that has value for evidence-based clinical decision-making. An ideal dental school graduate would be a highly skilled clinician with a deep understanding of the scientific foundation of dentistry, an appreciation of population health issues, and the compassion to serve. In the first 2 years of most dental schools, the vast majority of education consists of didactic instruction and simulation. Although the final 2 years are spent primarily in providing patient care, the likelihood is that most programs offer sparse instruction on understanding the health and social needs of low-income and underserved minority populations. Few dental programs offer supplemental public health electives.
To understand population-based research, oral health professionals need a basic understanding of epidemiology and biostatistics, health policy and management, health and wellness promotion, evidence-based dentistry, risk assessment at the individual and population levels, behavioral and social determinants, and health literacy. In addition, exposing future oral health professionals to real-world conditions—which can be accomplished through clinical rotations in community health centers, public health units, and other settings—provides them with experience treating low-income and underserved minority populations. Such exposure might also take place if they later enroll in Advanced Education in General Dentistry and General Practice Residency programs, which frequently serve these populations. In addition to these postdoctoral training programs, others can pursue public health training through a combined DDS and Master of Public Health or PhD program, thereby gaining valuable tools for research in underserved populations.
Imperative for preparing the next generation of scientists in oral health are core elements that the National Academies of Sciences, Engineering, and Medicine (2018a) recommend for all doctoral-level education. These include the cultivation of scientific and technologic literacy, the conduct of original research, and the development of leadership, communication, and professional competencies. PhD candidates should acquire deep, specialized expertise through the kind of original research that requires rigorous standards of investigation, as well as ethical responsibilities for the design and dissemination of science. They also should develop the ability to work collaboratively across scientific disciplines and be integral members of team science efforts (Hall et al. 2018).
Chapter 2. Advances and Challenges
Not only have substantial advances in genomics expanded our knowledge of factors affecting oral and craniofacial health in the past 20 years, new discoveries bridging microbiology and genetics have provided novel opportunities to better understand the role of microbiota in health and disease. Understanding the composition and ecology of the microbiota in our mouths opens new possibilities for treating conditions that link oral health and overall health, including systemic diseases.
Scientific breakthroughs in the past 20 years have significantly enhanced our understanding of stem cell biology and how to procure, isolate, and cultivate stem cells from embryonic and postnatal human tissues. Using stem cells to develop novel strategies for oral and craniofacial tissue regeneration is an exciting prospect. In the field of regenerative medicine, the identification of mesenchymal stem cells (MSCs) (stem cells found in skeletal and oral tissues that can be used to generate craniofacial structures or repair anomalies or damage) holds enormous promise for therapeutic applications.
In addition to scientific breakthroughs, we are in an age of accelerated technologic advancement that requires dedicated attention to make sure that new developments continue to promote health equity. Digital dentistry and the digital workflow are transforming all areas of dentistry. The health professions have opportunities to collaborate with industry, federal agencies, nonprofit organizations, and other entities to provide new strategies to improve care for all Americans.
An area of significant development since 2000 is the increasing availability of diverse data sources with the potential to inform dental care delivery and improve population oral health. The adoption of electronic health records (EHRs), including electronic dental records, during the past 2 decades has increased the availability of patient care documentation and can be used to improve communication and access to care. A growing number of social determinants of health datasets that describe communities’ social, economic, and physical environments now are available and provide context for a community’s overall and oral health (National Institutes of Health 2020a). Analysis of these datasets offers opportunities to improve oral health at the population and patient levels. The strategic inclusion of relevant oral health parameters makes it possible to provide a comprehensive picture of oral health as a part of overall health.
Foundational Sciences in Oral and Craniofacial Health
Omics/Gene Editing/Single-Cell Technologies
Single-cell technology was a conceptual vision in 2000 but has expanded into many areas of biomedical research in the past 5 years. Although oral and craniofacial research has lagged behind other types of research, some advances have been made. In the case of oral infectious diseases, the single cell sequencing of genomic amplicons (pieces of DNA or RNA that are the source or product of amplification or replication events) has been exploited to correlate the presence of uncultured bacterial species with oral health (Campbell et al. 2013; Beall et al. 2018b), and to detect severe acute respiratory syndrome coronavirus 2 (SARS-CoV-2) in various sites of the oral cavity (Huang et al. 2021), both of which open new pathways for salivary diagnostics. In looking at the levels of single-cell gene expression in patients with head and neck cancer, molecular heterogeneity among the same types of cells warrants attention because it can influence the effectiveness of an individual’s treatment strategy (Stucky et al. 2017). A single-cell analysis linked expanded salivary gland T cells to glandular dysfunction (Joachims et al. 2016) and revealed a higher frequency of activated T helper 17 cells (an immune cell population) in patients with Sjögren’s syndrome (Voigt et al. 2018).
Genome-wide association studies (GWAS) of oral and craniofacial diseases may show limited concordance among them due to random and systematic errors incorporated during study implementation (Agler and Divaris 2020). For example, in periodontal tissue studies, inherent genetic differences between the study populations, as well as inconsistencies in the precise definition of periodontitis cases and controls across studies, may be a problem. Given the multifactorial nature of periodontitis and the decisive roles of environmental factors such as bacterial exposures and cigarette use, it is reasonable to conclude that genetic information will explain only part of the variance of the disease at the population level. In the current post-genomic era, our inadequate understanding of crucial epigenetic mechanisms and transcriptional controls that regulate gene expression is slowing progress (Barros and Offenbacher 2014; Larsson et al. 2015).
To date, the majority of published single-cell techniques have been in embryology, neurology, and oncology. Although the applications of single-cell technology show promise, they have barely touched the mechanisms involved to affect applications in oral health and personalized dentistry.
Next-Generation Sequencing and Oral Diseases
During the last 20 years, next-generation sequencing (NGS) has become the norm for studying oral ecology, oral diseases, and the associations between the oral microbiome and systemic conditions. In addition to advances in understanding the basis of oral ecology, oral microbiome research has significantly expanded our knowledge of oral health and disease. In endodontics, the complexity and diversity of the microbiome associated with different root canal infections and treatment outcomes have been better defined, along with their relationships to clinical features (Sanchez-Sanhueza et al. 2018). In oral cancer research, several studies have found unique microbial signatures (Banerjee et al. 2017; Furquim et al. 2017; Zhao et al. 2017). Studies of the peri-implant microbiome have demonstrated its commonalities with the periodontal microbiome, including how smoking behaviors modulate it (Schincaglia et al. 2017). In addition, prominent members of the oral microbiome have been associated with several conditions, including colorectal cancer (Kostic et al. 2013), pancreatic cancer (Fan et al. 2018a), and lung cancer (Yan et al. 2015).
Pioneering studies of periodontal metagenomics (Duran-Pinedo et al. 2014) and metatranscriptomics (Yost et al. 2015) have confirmed the importance of classic pathogens. NGS has been an important tool for determining signature periodontal dysbiosis (Meuric et al. 2017), the microbial basis of the clinical stages of periodontitis (Boutin et al. 2017), and the latter’s initiation and progression (Yost et al. 2015). This technology has led to a broader study of the effects of periodontal treatment (Califf et al. 2016) and the individual and concomitant effects that smoking behaviors and diabetes (two risk factors for periodontitis) have in promoting an at-risk microbiome (Giannobile 2013; Ganesan et al. 2017).
Microbiome/Inflammasome/Virology
Microbiome research has grown significantly because of the availability of polymerase chain reaction (PCR) methods mentioned in the 2000 Surgeon General’s report on oral health. Over the past 20 years, there has been an evolution in methods and a revolution in knowledge. One of the greatest advances in oral health is the greatly expanded knowledge of the diversity and biology of the microorganisms found in the oral cavity.
Microbiome studies have evolved from the use of bacterial-specific probes (Dewhirst et al. 2010) to the characterization of the entire microbiome community (Escapa et al. 2018). Such in-depth characterization has led to greater understanding of the microbial community, its interactions with the host, and its contributions to health and disease. Technologic advances are revolutionizing the traditional concepts of microbial virulence and pathogenesis (Lamont et al. 2018; Al-Hebshi et al. 2019). Oral pathogens, long regarded as key contributors to the development of dental caries and periodontitis, have now been recognized as mediators in tuning microbe-host interactions and in tipping the balance between microbial synergy and dysbiosis (Lamont et al. 2018). Human microbiome studies that selectively target specific organisms are revealing conserved biochemical activities and signaling cascades (Olsen and Potempa 2014; Garcia et al. 2017), which have led to promising compounds that make precision therapy possible.
Oral Microbiome and Oral Diseases
The number of studies using molecular approaches to characterize the periodontal and dental caries microbiomes has increased enormously during the past few years. These studies indicate that a large number of non-cultivable species are associated with oral biofilms during periodontal inflammation (Wade 2013), making it clear that periodontal and dental caries pathogenesis does not result from the actions of a single or even a few bacterial species, but instead requires complex and subtle interactions between specific bacterial species and the host. The polymicrobial synergy and dysbiosis hypothesis proposes that a potentially pathogenic community arises from the actions of a few bacteria, termed keystone pathogens (Darveau et al. 2012).
Important advances for the study of the oral microbiome during the past 20 years include the development of the Human Oral Microbial Database (HOMD) (https://www.homd.org) (Chen et al. 2010), as well as myriad innovative molecular techniques to identify new and emerging microorganisms associated with disease. The HOMD catalogs partial and complete genomes of more than 800 of the most common microorganisms that colonize the oral cavity, including uncultivated taxa. As a result of collective efforts in the field, this segment of the microbiome, which was once estimated to constitute 50% of the oral microbiome, is now estimated to be 30%—in part because of the cultivation of previously uncultivated taxa (Vartoukian 2016).
As information about the composition of the oral microbiome continues to grow, its influence on other aspects of health are becoming more apparent across the life course. For instance, the relationship between the composition of the oral microbiome and adverse pregnancy outcomes continues to strengthen (Saadaoui et al. 2021). In addition, the mode of delivery (cesarean section vs. vaginal birth) (Lif Holgerson et al. 2011) and early diet (breastfeeding vs. formula), as well as the maternal microbiome and tooth eruption (Mason et al. 2018), are shown to have an impact on the structure of infants’ and children’s oral microbiomes. With regard to adults, new insights have been gained into the harmful interactions between microbial communities and heavy alcohol consumption (Fan et al. 2018b), diabetes (Ganesan et al. 2017), and possibly Alzheimer’s disease (Dominy et al. 2019), among others.
Despite major advances in microbiome research, gaps in knowledge remain. The complexity of the dental, oral, and craniofacial environments contributes to varied biological ecosystems that dictate the progress of disease. Research challenges related to unresolved dental pulp and periapical infections need to be considered. Microbial signatures of disease activity, progression, and risk have been identified, but have not yet been effectively translated into clinical use to predict disease activity, recurrence, and response to different types of treatment. In addition, even though the most common oral diseases are infectious, and knowledge about them has expanded, there is a need to better understand the basis of current antimicrobial treatments, the rise and transmission of bacterial antibiotic resistance, and the development of new antimicrobial approaches. Despite improvements in understanding the causes and progression of oral diseases and the connection between the oral microbiome and systemic health, it still is unclear whether the microbiome can be used as a tool to predict disease risk and response to specific treatments. Larger epidemiologic and mechanistic studies are needed to identify and validate early disease markers.
Current studies rely on the taxonomic grouping of microorganisms at a species level, with very scarce strain-level information. Although studies performed before the high-throughput sequencing era showed that different strains of microorganisms possess distinct pathogenic attributes, progress in understanding microbiome-wide strain associations with disease and the functional significance of strain variability has been limited.
The development of methods to manipulate the oral microbiome is a continuing challenge. Although several disease-related synergistic interactions among microbiome members have been described, little progress has been made in the development of methods to control microbiome communities that target key microorganisms or to manipulate environmental conditions to preserve health-associated communities.
There are other challenges. Most oral microbiome studies fail to reference microbiomes residing in other parts of the body (Graves et al. 2019), but given the connectivity between oral and other microbiomes, disregarding key partners may give rise to biased conclusions. Moreover, investigators involved in oral microbiome research have not directly addressed the possibility of selection bias in their samples. Reports have shown that GWAS have been overwhelmingly dependent on participants of European ancestry (Popejoy and Fullerton 2016), and that same bias may be reflected in studies of the oral microbiome. If so, those who are experiencing the greatest disparities related to oral disease, and who could most benefit from this research, may be underrepresented in key studies, thereby compromising the validity and applications of this work. Microbiome studies also yield enormous amounts of data with large variations. Finding a pattern in microbial genes, species, and pathways associated with a specific function of interest in the sea of big data is a complex task (Pinu et al. 2019). The cost and logistics involved in studying the microbiome longitudinally may prevent investigators from compiling a complete picture of how it responds to environmental and host cues over time.
Choosing the right path forward to develop microbiome-targeted therapies also is challenging. Exciting discoveries in new species (Berdy et al. 2017) and in polymicrobial and microbe-host interactions (Stacy et al. 2016; Lamont et al. 2018) offer opportunities to develop effective treatments. Designing and developing drugs that target bacterial factors or inhibit select bacteria are on the horizon (Zhu et al. 2015; Koo et al. 2017; Stone and Xu 2017). However, given the complexity of the microbiome community (Baker et al. 2017), it is challenging to predict how therapeutic options would prevail without a complete understanding of the molecular details of the targeted microbiome. The study of the microbiome and inflammasome is still in its infancy (Stulberg et al. 2016), and further basic and translational science with clinical applications is needed.
Genetics Versus Environment
The last 2 decades have provided important information in determining if inherited traits or life experiences play a greater role in health. Comparing dental plaque and saliva samples from non-Hispanic Black, White, Latino, and Chinese persons (Mason et al. 2013) showed ethnicity-specific microbial signatures. In a subsample of two large Ashkenazi Jewish families (Shaw et al. 2017), the environment (i.e., sharing the same household) was more strongly associated than genetics (i.e., kinship) with the salivary microbiome. The microbial analysis of supragingival plaque microbiomes of 485 dizygotic and monozygotic twins showed that oral microbiome similarity increased with shared host genotype (Gomez et al. 2017). In another study examining the genetics of transmission by comparing the oral microbiota of biological versus adoptive mother-child pairs, findings supported the conclusion that shared environment and contact is more important in oral bacteria acquisition than genetic susceptibility (Mukherjee et al. 2021). Collectively, these findings suggested that under high genetic similarity (i.e., kinship vs. ethnicity), the environment’s effects on disease development may prevail.
Epigenetic Modification of Viral Genomes by Oral Pathogens Links Viruses and Bacteria
The field of epigenetics, almost unknown at the time of the 2000 Surgeon General’s report on oral health, has now exploded into a highly relevant area for understanding oral disease pathogenesis. Viruses and bacteria have developed diverse mechanisms to directly affect host cell epigenetics, driving pathogenesis and cancer development.
Significant progress has been made during the past 20 years in understanding oral environmental triggers that engender epigenetic reprogramming related to tumor viral pathogenesis in the mouth. The mouth harbors billions of oral bacteria at any given time (Dewhirst et al. 2010). It is now understood that bacterial metabolites can affect virally infected cells, particularly in a setting of viral latency (Morris et al. 2007; Gorres et al. 2014). Findings have suggested that bacterially secreted products may alter both host and viral gene expression through epigenetic regulation. Active viral replication and shedding occur in the oral cavity, suggesting an oral environmental trigger that allows reactivation from a viral latent state.
Human papillomavirus (HPV) hypomethylation is associated with cervical cancer and with head and neck cancer, and progressively less methylation was seen in patients with carcinoma, compared to those with precancerous lesions and asymptomatic infections (Faraji et al. 2017). Studies have suggested that periodontopathic bacteria are a factor in the regulation of cancer-associated viral oncogenes (Cunningham-Glasspoole 2015) and have suggested an additional link between bacteria and HPV-associated cancer.
Inflammation and Inflammasome
The term inflammasome did not exist at the time of the 2000 Surgeon General’s report on oral health. Since then, more than 30,000 articles have been published with inflammasome as a keyword, and nearly 700,000 have been published with the keyword inflammation. A patient with cancer, periodontal disease, or type 2 diabetes can now be monitored and treated for uncontrolled inflammation-associated tissue by measuring inflammasome levels in saliva, gingival fluid, and oral tissues, which have positive correlations with systemic and blood inflammasome levels. Saliva is the most readily available source for testing and can be sampled noninvasively (Isaza-Guzman et al. 2017; Lee et al. 2018). Available therapeutic approaches aimed at inflammation control are focused on medications such as nonsteroidal anti-inflammatory drugs and selective anti-inflammatories that suppress essential inflammatory pathways but come with risks. Developing new strategies to activate beneficial anti-inflammatory pathways, such as pro-resolution mediators of inflammation (e.g., resolvins, transforming growth factor beta, and interleukin [IL]-10) could offer medications with fewer side effects. Successful targeted therapy against IL-1 has been developed to control inflammation in rheumatoid arthritis. Developing targeted drugs specific to dental, oral, and craniofacial diseases would be beneficial for controlling local and systemic inflammatory signals.
Inflammation accompanies disease development, tissue changes, and tissue loss. Although genetics and multiomics have advanced during the past 20 years, there have been limits to effectively applying this knowledge to clinical practice. Current methods for diagnostics and disease monitoring fail to capture the complexity of inflammation kinetics at the individual and population levels. In diagnosis and monitoring of periodontal diseases, tissue levels are taken into consideration without regard to molecular levels of inflammation. Furthermore, the translation of knowledge about mediators of inflammation into clinical applications that mitigate tissue destruction has been slow and needs to be advanced.
Regenerative Medicine, Dental Materials, and Bioinspired Materials
There has been an explosion of new technologies introduced to dentistry for regenerative applications (Bayne et al. 2019), most especially in the areas of aesthetic dentistry and in less-invasive techniques to conserve tooth structure through prevention and early treatment of diseased tissue.
Regenerative Medicine and Dental Repair
Continuing challenges regarding existing biomaterials include a relatively high incidence of recurring decay around bonded dental restorations; a higher than desirable rate of fracture of dental composite fillings because of their limited strength; a lack of ideal aesthetics for the highest strength ceramic materials, such as zirconia; and a higher than desirable incidence of infection around dental implants that may lead to premature failure. Other key challenges include the development of materials that have biological effects, such as inhibiting bacterial adhesion and colonization at sensitive tooth-adhesive interfaces, and materials that promote the production of new tooth mineral to replace lost tooth structure. The development of a material that can replace infected pulp tissue to avoid endodontic therapy or tooth extraction could be critical for saving severely damaged teeth. Despite the great success of dental implants, problems leading to failures and implant loss still occur. One area of intense interest is to better understand the incidence of peri-implantitis, in which tissues become inflamed where the implant emerges from the bone and is in contact with the gingival tissues (Berglundh et al. 2019).
Perhaps the area that appeared the most promising 20 years ago but has seen relatively little practical advancement because of its tremendous complexity is the in situ regeneration of new teeth (Monteiro and Yelick 2017). Natural-appearing teeth have been grown in small animals (Yelick and Vacanti 2006) and in larger mammals (Wu et al. 2019), but the implantation of such teeth, or the regeneration of new, anatomically correct hard tooth structure within a human mouth, remains a more distant goal.
Dental Adhesives and Dental Composites
Today, adhesive and composite materials have mostly replaced dental amalgam as the primary restorative materials. Dental adhesives and dental composites are similar polymer-based materials that restore lost tooth structure by bonding to and sealing the tooth to maintain its integrity and resist further tooth decay (Bedran-Russo et al. 2017; Pfeifer 2017). New dental adhesives and composites represent a true revolution in dental treatment as a result of intense research and product development, especially for the resin component (Fugolin and Pfeifer 2017). As a result of significant advances in the past 20 years, current materials may last for decades when placed appropriately and in patients with good oral health (van de Sande et al. 2013). Research into advanced formulations of the materials has mostly solved the situation of excessive wear on posterior teeth from chewing, a major problem 20 or more years ago (Ferracane 2006).
Many clinical studies have shown average lifetimes for restorations that approach 15–20 years or more in both anterior and posterior teeth (Bayne et al. 2019). However, other studies have shown much lower rates of success and the need for earlier intervention, possibly within 6–7 years of placement (Kopperud et al. 2012; Rho et al. 2013). The reason for this discrepancy is at least threefold. First, the most common reason for failure was secondary dental caries. Second, the adhesive and composite materials have characteristics that require a demanding, multistep placement technique that can lead to premature failures. Third, composites also shrink during curing, which may produce stresses that challenge the adhesive’s bond to the tooth. One approach to address this issue is the development of new resin formulations with reduced contraction stress (Meereis et al. 2018). Many commercial products have been produced, including easier to place bulk-fill composites (Veloso et al. 2019). Another approach has been the development of adhesives that directly bond to the tooth but do not require the tooth to be pretreated with an acid solution to promote bonding. Some of these self-etch adhesives have shown favorable clinical results (Peumans et al. 2010; van Dijken 2010). Another important factor influencing premature restoration failure is that many patients’ diets and oral habits predispose them to recurrent dental caries. In the future, it is critical that research focuses on the production of materials that are more robust, simpler to use, and inherently effective against the attachment of bacterial plaque.
Dental Ceramics
Dentists are familiar with the use of and indications for metal alloys, which have provided stable and durable restoration of tooth structures for more than 100 years (Donovan et al. 2004). Although predominantly gold-based alloys have been the primary material for dental crowns and bridges that replace one or more teeth, dental ceramics (such as dental porcelain) have emerged as a viable and sought-after option to minimize or eliminate the use of metals in the mouth (Zhang and Kelly 2017).
Dramatic advances in materials that provide strong and tough ceramics, such as lithium disilicate and zirconia, have revolutionized the replacement of broken and severely decayed teeth with materials that are durable and resemble natural teeth (Denry and Kelly 2014; Zhang and Lawn 2018). These new materials, especially lithium disilicate, are highly aesthetic and strong, although their use in heavy dental occlusion is still limited. Increased use of zirconia ceramic, which is nearly twice as strong as any other dental ceramic and requires less tooth reduction, has had a noted impact in dental care (Ghodsi and Jafarian 2018).
Dental Implants
In the past, tooth loss was treated by placing a dental bridge, which requires cutting away the two sound adjacent teeth to prepare them as abutments to hold the bridge with the replacement tooth crown. In the past 20 years, however, dental implants, which offer distinct advantages over dental bridges, have become a normal part of dental treatment (Howe et al. 2019). Dental implants typically are made from titanium because of its strength and excellent biological response, although new high-strength ceramics, such as zirconia, are increasingly being used in areas where metal may partially show in the mouth because of their high biocompatibility and improved aesthetics. Dental crowns or bridges are placed on the implant. The survival of tooth-supported and implant-supported three-unit fixed dental prostheses has been shown to be similar (Pol et al. 2018). The key to the greater success of the dental implant is that it becomes solidly anchored through the growth of new and supportive bone around the device, a process called osseointegration (Bosshardt et al. 2017). Several factors have been shown to affect this process. Effort is now focused on better understanding the optimal implant surface texture, such as roughening on the micro- or nanometer scale, to create an optimal tissue response at the implant surface (Albrektsson and Wennerberg 2019). Implant characteristics, together with local and systemic host factors, may affect susceptibility to peri-implantitis, which remains one of the most common biological complications of functional implants (Rakic et al. 2018; Hashim and Cionca 2020).
Biological factors offer significant potential for tissue regeneration around teeth and for dental implant site development (Larsson et al. 2016). Enamel matrix derivative (EMD) is the earliest-studied biologically active product for periodontal regeneration (Koop et al. 2012). EMD is available commercially as an injectable gel solution containing enamel matrix proteins (amelogenins and other enamel proteins) and a carrier, propylene glycol alginate. Systematic reviews have shown that EMD delivers improved clinical outcomes compared to open-flap debridement (Esposito et al. 2009; Koop et al. 2012). Recombinant human platelet-derived growth factor-BB, combined with β-tricalcium phosphate, is another commercially available product that has shown promising results for addressing regenerative intrabony and furcation (bone loss in the area where the tooth branches off from the root) defects in periodontal treatment (Nevins et al. 2013). Other growth factors, such as fibroblast growth factor 2, have been under clinical development and have demonstrated promising results in human clinical trials (Cochran et al. 2016).
Nanotechnology
The safety of nanoscale materials is a continuing challenge. Nanoparticle toxicology is still an emerging field, with inconsistencies in the published literature. Tests to determine nanoparticle toxicity have not reached consensus (Webster 2009). Furthermore, although many nanomaterials have been claimed to lead to unprecedented improvements in efficacy—in terms of physical properties, biological interactions, and control—dental materials and technologies that rely on nanoscale systems have yet to deliver paradigm-shifting outcomes. Improvements have mostly been incremental and on par with those from many traditional microscale technologies.
Stem Cell Biology
Over the last 2 decades, advanced molecular biology tools and bioinformatic capabilities have become available that use machine learning algorithms to rigorously characterize different stem cell populations on the basis of their molecular signatures. This deeper understanding of stem cells’ unique molecular profiles will enable more targeted therapies for specific applications.
In 2000, the fields of tissue engineering and regenerative medicine were just emerging, stem cell therapy for craniofacial regeneration was merely a concept, and bone marrow was the only known source of MSCs. Since that time, tremendous technologic, clinical, and scientific advances have led to a deeper understanding of MSC biology, including how MSCs interact with other cells, how to isolate them from different tissues, how to control their behavior with different signals and microenvironments, and how to deliver them to local and distant sites. A new paradigm has been proposed for the mechanisms of action of MSCs in the laboratory, on the basis of their potent immunomodulatory, anti-inflammatory, and multifaceted trophic (stimulatory or growth) effects, such as proangiogenic, tissue-remodeling, antioxidant, and antiapoptotic effects (Shi et al. 2018).
MSCs can migrate to injured and inflammatory sites, actively sense the signals or cues from damaged cells, and communicate with other types of cells, particularly immune cells. Once activated, MSCs respond with increased proliferation activity, including secretion of several biological factors to modulate immune cell functions, promote blood vessel growth, suppress cell death, and reduce reactive oxygen species (also sometimes called free radicals), thus establishing a regenerative microenvironment.
To date, more than 800 clinical trials using MSCs have been registered with ClinicalTrials.gov; in those trials, MSCs of different origins are being tested for their therapeutic effects on a wide spectrum of autoimmune, inflammatory, and degenerative diseases (Shi et al. 2018). Although there are a number of MSC products being used to treat patients with dental, oral, and craniofacial conditions under the practice of medicine, there are no MSC products yet approved by the U.S. Food and Drug Administration (FDA) (U.S. Food and Drug Administration 2020b).
Despite their potential to differentiate into cells of all three germ layers, the clinical use of embryonic stem cells (ESCs) faces several major challenges. There are critical ethical challenges, difficulties involved in the differentiation of transplanted ESCs into mature and physiologically functional cell types capable of integrating into the relevant damaged tissues, long-standing issues of immunogenicity, and potential tumorigenicity as a result of genomic instability (Guhr et al. 2018; Prentice 2019).
The use of MSCs of different tissue origins as therapeutic products in regenerative medicine presents many biological, manufacturing, and clinical challenges (Martin et al. 2019). More stringent methods of characterizing these heterogeneous cell populations are needed to elucidate the mechanisms underlying cell variability (Huang et al. 2009; Bianco et al. 2013). Another challenge is to determine the best modality in which to deliver stem cells. Different rigid and injectable polymers have been used as scaffolds, but information has been insufficient to drive clinical efficacy. Overcoming these challenges with robust, well-designed clinical trials utilizing fully characterized source materials is instrumental for gaining FDA approval.
Cost-effective reimbursement models and protocol logistics for the isolation, expansion, and delivery of stem cells need to be established to bring technologies to clinical adoption. Stem cell manufacturing that meets the appropriate regulatory and safety standards likely will be expensive and increase the costs for the clinician and the patient. In addition, current reimbursement by dental insurers provides insufficient coverage for regenerative therapies in dentistry. As a result, these approaches may widen health disparities. Despite a considerable market for bone (an estimated US$750 million) and dental pulp (an estimated US$200 million) regeneration, many companies are cautious about committing resources because of existing limitations and inadequacies of third-party reimbursement for new treatment procedures. Academic partnerships with industry will need to be fostered to establish cost-effective models for autologous stem cell production.
Discussions of stem cell biology would be incomplete without mentioning the progress during the past 20 years in understanding cancer stem cells’ role in the development, progression, and therapeutic resistance of cancer (Baillie et al. 2017). Cancer stem cells can self-renew and proliferate, have been identified in head and neck cancer, and are highly capable of forming tumors. Advances in their identification and characterization have stemmed from the cell and molecular technologies developed and explored during the past 20 years and are currently being expanded with new technologies such as single-cell approaches (Qi et al. 2019).
Science and Technology for Practice
Over the past 20 years, biotechnology and digital and information technology are emerging trends that have strongly influenced the diagnosis, treatment, prevention, documentation, and management of oral diseases. In the context of new technologies for clinical application, bioethical implications at both the individual and society levels need to be continually considered (Anderson and Anderson 2019; Resnik 2019; Scheper 2019). In the educational setting, academicians must be aware of potential conflicts of interest if they associate themselves with pharmaceutical or technology companies. The speed with which new technologies can be incorporated into practice needs to be tempered with accurate information and evidence-based investigation. The potential of new advances to reduce health disparities also is a critical consideration.
Genetics- and Genomics-Based Precision Health
As the costs of sequencing DNA have decreased during the past 20 years, the use of whole-exome (the part of the genome that codes for proteins) and whole-genome (the entire 3 billion base pairs of DNA) sequencing has increased. These tests have revealed genetic mutations underlying dental conditions, such as amelogenesis imperfecta (Smith et al. 2019), tooth agenesis (Salvi et al. 2016), and latent transforming growth factor-betabinding protein 3 (LTBP3)-related disorders (Intarak et al. 2019). LTBP3 pathogenic variants are associated with amelogenesis imperfecta, short stature, and predisposition to thoracic aortic aneurysms and dissections (Guo et al. 2018). Many dental genetic variants are associated with more severe conditions. Dental findings, including radiolucencies (defects that appear as darkened areas on radiographs), have been associated with some types of mucopolysaccharidosis and Gaucher disease (Saranjam et al. 2013). Oligodontia (an absence of six or more teeth) can be associated with colon cancer (Lammi et al. 2004). One genetic variant of amelogenesis imperfecta with gingival hyperplasia is associated with nephrocalcinosis (an increase of calcium levels in the kidneys). These findings suggest that persons with these oral signs should be referred for a renal workup (Koruyucu et al. 2018).
The advent of new approaches, such as clustered regularly interspaced short palindromic repeats (CRISPR)-associated protein 9 and the expanded capabilities for gene editing, including ex vivo cell therapy and in vivo gene editing (Raaijmakers et al. 2019), as well as DNA vaccines, chimeric antigen receptor therapies, pharmacoepigenetic approaches to manipulate targeted genomic expression, and gene replacement therapies show promise for improved clinical strategies in genetic conditions.
In contrast with disorders caused by a single genetic variant, most chronic diseases are complex and caused by the interactions of multiple genes, environmental exposures, and lifestyle factors. These multifactorial origins characterize conditions such as diabetes, heart disease, hypertension, and common cancers.
Environmental factors can modify DNA or the histone proteins in chromatin, altering gene regulation and expression and influencing disease (Berdasco and Esteller 2019).
Factors other than the actual nucleotide sequence play a role in gene expression. Changes that do not affect the actual DNA sequence are epigenetic in nature and involve methylation of DNA or histone modifications. The environment can cause epigenetic modifications, for example, through diet, smoking behaviors, exposure to microbial agents, gender, aging, drugs, and toxins (Niller and Minarovits 2016; Berdasco and Esteller 2019). Such environmental exposures are important in the development of many conditions dentists encounter, such as dental caries, periodontal disease, and oral cancers. Many pathologies of the oral cavity are linked to systemic conditions such as diabetes, heart disease, and obesity, which also have epigenetic components in their development.
Advances in understanding genomic architecture and the role of RNA biology have altered our perception of how the genome functions and interacts with genetic and environmental factors to regulate health and disease, yet much is still unknown (Jukam et al. 2019). To fully participate in these genomics initiatives, the dental community will need to overcome barriers to the integration of all health care records and interprofessional training (Regier and Hart 2016; Weitzel et al. 2016).
Pharmacogenetics, which is increasingly playing a role in dental care, uses information about an individual’s genome to choose drugs and drug doses likely to work best for that person’s unique genetic profile—a departure from the one-drug/one-dosage-fits-all approach. Pharmacoepigenomics target pharmacologic treatments that may reduce or reverse epigenetic effects in diseases. Clinical applications of pharmacoepigenomic interventions have increased and show promise for treating oral and craniofacial diseases that result from a combination of genomic, metabolic, epigenomic, and environmental factors (Teijido and Cacabelos 2018; Jones et al. 2019). An example of progress in which genetic strategies may be particularly valuable is a targeted approach aimed at the tumor suppressor p53 protein, which shows promise in sensitizing head and neck cancer stem cells to chemotherapy (Rodriguez-Ramirez and Nör 2018).
One of the most revolutionary advances in medicine in the last 20 years has been the incorporation of genetic information into clinical care. The dramatic reduction in the cost of acquiring genomic information and the use of information technology to understand clinically useful relationships among genomic data, health, and disease are accelerating the process. Genomic data linked to extensive phenotypic and health information are being collected on millions of individuals across ethnic groups, enabling clinical research in data biobanks and creating the possibility of asking more complex genetic questions (Tyler-Smith et al. 2015; Stark et al. 2019; Verma et al. 2019), which will help characterize healthy states and disease-associated conditions, identify robust predictive associations (clinical validity), and facilitate evaluation of clinical utility (Ioannidis and Khoury 2018). Careful clinical characterization, including longitudinal data, will facilitate identification of disease-associated genetic variants in humans. Despite promise, the genetic information revolution has had very limited impact on dental care, with yet untapped potential for managing oral health in the future.
A continuing challenge to the dental community is the integration of increasingly available genomic information into clinical practice in valid and useful ways. Barriers need to be overcome, including data collection, recording, and interpretation. Surmounting these barriers will likely require changes to health records and introduction of multidisciplinary health teams. Regulatory challenges and determination of evidence for clinical utility need to be addressed. Dental education and training will need to incorporate genetics education and interprofessional collaboration (Johnson et al. 2008).
Although big data has a role in precision medicine, certain risks do exist. Ethical, legal, and social tenets of genomics need to be applied to ensure privacy and confidentiality (Dolley 2018). Maximizing the utility of genomic information in clinical care requires integration of health records and the incorporation of genomics into EHR systems (Ohno-Machado et al. 2018). Standards for best practices in analysis and interpretation of clinical genomic information are needed (Brownstein et al. 2014; Lu et al. 2018). Because genomic factors affect many diseases across the life span and across clinical disciplines, no single professional group encompasses the entirety of this field, reinforcing the need for genomics education and interprofessional practice (Schully et al. 2015).
Diagnostics
Innovative Diagnostic Imaging Systems
Examples of the technologies currently being used in dental therapeutics include digital planar and 3D radiography, static occlusal and jaw-tracking software, and intraoral scanning of hard and soft tissues for disease diagnosis and digital treatment. In addition, computer-aided design and manufacturing workflows can assist in procedures such as endodontic access, crown fabrication, and surgical guides for complex head and neck surgeries (Shah et al. 2014). Emerging technologies, including optical coherence tomography, allow clinicians to peer through mucosal tissues to underlying teeth, bone, and cartilage. Ultrasound and magnetic resonance imaging (MRI)-based soft tissue scanning systems have been developed for use in dentistry to better assess patients’ oral health status with extensive physiological and pathologic information. Some additional tools, such as Raman spectroscopy, which reveals the molecular and related health status of scanned tissues, are being explored for their capacity to further enhance dentists’ diagnostic capabilities, accuracy, and efficiency. However, the size and cost of MRI scanners and other neuroimaging tools (e.g., positron emission tomography, magnetoencephalography) are substantial challenges that need to be overcome to realize their broader clinical applications in dental care.
Diagnostic Biomarkers in Saliva
Since 2000, omics studies have greatly accelerated the discovery of biomarkers of human genetics and the oral microbiome associated with dental diseases. Rapid sequencing has made genomic analysis of the microbiome more widely applicable. Growing databases with improved software make analyses more accessible and increase the opportunities to explore systemic linkages with oral health.
Saliva-based genomics offers unique advantages, above and beyond oral health diagnostics. The presence of our entire genome in saliva has been used to access personal genomic information pertaining to ancestry, health, and wellness. Saliva samples and NGS can reveal genomic variants (e.g., single nucleotide polymorphisms) that confer risk for health conditions (e.g., cancer, such as variants in the genes for breast cancer type 1 and type 2 susceptibility proteins) and can reveal ancestry information. Using a noninvasive approach—gathering a saliva sample—to derive genomic information for personalized and precision medicine applications has promising potential.
Liquid biopsy can now detect circulating extracellular RNA (exRNA), circulating exosomes, and circulating tumor DNA (ctDNA) in human biofluids. This approach is used to detect lung cancer cells using saliva markers of the epidermal growth factor receptor gene mutations. Anticancer drugs can target this gene and improve lung cancer patients’ survival rates (Wei et al. 2018). Cell-free DNA shed from tumor cells into the circulatory system, or ctDNA, can be detected in a number of body fluids, including blood, urine, and saliva, where its mutations distinguish it from normal cell-free DNA. The most common technologies for the detection of ctDNA are NGS and digital PCR. Salivaomics-based liquid biopsy for exosomes and exRNA and ctDNA detection has potential for clinical translation; however, challenges remain on documenting robust evidence for the sensitivity, specificity, accuracy, and reproducibility of these assays.
Digital Technology for Advancing Diagnostics
There has been dramatic progress in digitization in dentistry, which has created a new market and value network that will disrupt existing norms. Digitization has the potential to improve early detection of dental diseases, reduce cost of treatment, and increase treatment reliability by decreasing errors in dentistry. These innovations offer an opportunity to rethink oral disease prevention and health care delivery in the context of providing public health programs to underserved populations. Current versions of intraoral scanners, using a laser fluorescence detector or near-infrared light transillumination, have incorporated dental caries detection, and because they are relatively easy to use, these technologies have the potential to become important diagnostic tools for the early detection of dental diseases. However, clinical validation and accuracy assessments suggest that further development work is needed for these technologies to perform equivalently to traditional visual-tactile detection methods (Abogazalah and Ando 2017; Kocak and Cengiz-Yanardag 2020).
Current radiographic methods present challenges in their sensitivity for detecting occlusal lesions and underestimation of the depth of penetration of proximal lesions (Ricketts et al. 1997). Stain and plaque interfere with porphyrin-based fluorescence systems (Shi et al. 2000).
Although the use of fluoride has greatly reduced the prevalence of dental caries, methods are needed for the quantitative assessment of fluorosis, which has become a growing problem (Baker et al. 2017). Current methods rely on subjective visual examination and use an ordinal scale poorly suited for identifying differences in the severity of mild fluorosis among communities. Angmar-Mansson and colleagues (1994) studied several optical techniques for improving the assessment of dental fluorosis, which is characterized clinically by diffuse opacities. Quantitative light-induced fluorescence (QLF) has been used as a potential diagnostic tool for assessing fluorosis because the subsurface porosities scatter light in a manner similar to demineralized carious lesions (Li et al. 2003).
McGrady and colleagues (2012) (Pretty et al. 2012) employed QLF in an epidemiologic fluorosis survey in fluoridated and non-fluoridated communities in England and Thailand. The Centers for Disease Control and Prevention has developed a system building on that work using both QLF and polarized visible light for oral health examiners in the United States.
Unfortunately, visible and fluorescence-based optical measurements suffer from the interference of extrinsic stain, specular reflection (glare), variations in tooth color, variations in enamel thickness, and low contrast between normal and hypomineralized enamel. However, interference from stains does not affect imaging systems operating at longer wavelengths, which have advantages for assessing the severity of fluorosis (Fried et al. 2013). Optical coherence tomography at near-infrared wavelengths is ideally suited for measuring the subsurface structure of fluorotic lesions to quantify their depth and severity (Hirasuna et al. 2008). It has not been confirmed that the pattern and distribution of the lesions attributable to fluorosis are a unique occurrence. A method that can measure the depth of defects may provide a measure of severity, which can be used to determine the feasibility of removal or treatment.
Three-Dimensional Bioprinting and Additive Manufacturing
Developments in the past 20 years are shifting trends in dental reconstruction away from conventional impression technologies and toward digital imaging. Cone beam computed tomography (CBCT) and intraoral scanners are now used to design and produce dental prostheses and surgical guides that can be fabricated using additive manufacturing, as well as the more traditional subtractive manufacturing technologies (Abduo 2019). Additive manufacturing, or additive layer manufacturing, is the production name for 3D printing in which a computer-controlled process creates 3D objects by layering materials. Technologies of this type have made complex treatment feasible for most dentists and have decreased manufacturing time. Potential challenges include consistent dimensional accuracy and material suitability.
A wide selection of platforms, printable materials, and applications now is available in medical 3D printing (Tappa and Jammalamadaka 2018). Materials include most major classes: thermoplastics, thermosets, gels, ceramics, and metals. Each material requires specific processing strategies and precursor materials—for thermosets, a liquid resin; for metals, specially prepared powders. Because biomaterials have a variety of advantages and disadvantages, this lends them to specific or potential uses in health care (Table) (Tappa and Jammalamadaka 2018).
Perhaps the most widely adopted technology in dentistry is vat polymerization, in which a light source (such as a laser) is directed onto a photocurable resin to build it up layer by layer. Examples of the products of this technology include orthodontic aligners, mouthguards, and surgical guides. Several metal printers have been advocated for dental use to fabricate fixed and removable partial-denture frameworks as well as to fabricate custom implantable devices (Forrester et al. 2019). Several variations of vat photopolymerization, including continuous liquid interface production, digital light projection, and stereolithography, are used by many laboratories to print patterns for fixed partial-denture frameworks for conventional casting methods. The availability of aligner software for orthodontic movement of teeth and fabrication of provisional restorations has increased demand for faster printers (Abduo 2019; Gonzalez Guzman and Ohara 2019).
Conventional tissue engineering approaches have limitations for fabricating vascularized and functional tissues and organs (Ozbolat and Hospodiuk 2016). In the last decade, advances in stem cell biology, biomaterials science, tissue engineering, and 3D bioprinting technology have significantly advanced the fabrication of implantable artificial tissue complexes for regeneration of both soft and hard tissues (Gomes et al. 2017). Bioprinting is a computer-driven, rapid prototyping technology that utilizes computer-aided design software to generate blueprints for highly accurate biomimetic tissue constructs (Kang et al. 2016). This state-of-the-art platform for fabricating complex biomimetic tissues using biocompatible bioinks mimics the chemical, physical, and physiological properties of native tissues. Various types of synthetic or natural polymers, different types of cells, and growth factors have been implemented as the cellular and noncellular components for 3D bioprinting for regenerative purposes (Nagarajan et al. 2018). Because of their ability to become different types of cells and their regenerative potential, MSCs have been extensively explored as the seed cells for generation of tissue engineering and regenerative medicine products, including 3D bioprinting of biomimetic tissue constructs (Snyder et al. 2015).
Despite advantages, the use of printed objects in dentistry is still limited. Definitive restorations (e.g., crowns, bridges) cannot yet be manufactured because of the lack of suitable materials. In subtractive manufacturing, the most commonly used materials for definitive restorations are reinforced ceramics, metals, and composite resins. In additive manufacturing, some polymer prototypes and metals are starting to find their way into clinical practice, although 3D-printed ceramics for dental applications are still struggling to be accepted (Dehurtevent et al. 2017). Metal is commonly used for conventional prosthodontic treatment, and promising clinical outcomes have been reported with posterior single-unit metal-ceramic crowns (Abou Tara et al. 2011; Atzeni and Salmi 2015). Despite its good mechanical properties, metal is not suited for modern microinvasive and aesthetic treatments, whereas 3D-printed reinforced polymers show better potential for final restorations, but they need further development.
Table
Biomaterials classification with their advantages, disadvantages, and applications.
Complementary, Alternative, and Integrative Medicine in Dental and Oral Care
The use of complementary, alternative, and integrative medicine is extensive, but limited by issues of safety, research and evidence, and regulation. These approaches may include nutritional (e.g., herbs, dietary supplements), psychological (e.g., meditation, hypnosis) and/or physical (e.g., acupuncture, massage) interventions (National Center for Complementary and Integrative Health 2021).
Herbal preparations are commonly used by U.S. adults (Wu et al. 2014). Because herbs are regarded as food products, they are not subject to the same scrutiny and regulation as conventional medications. Various herbal supplements have been reported or are suspected to interact with certain dental drugs. The use of some herbal supplements is associated with oral manifestations including aphthous ulcers, lip and tongue irritation, swelling with fever, tongue numbness, dry mouth, oral and lingual dyskinesia, and salivation (Tachjian et al. 2010).
Information and Data Science
Big Data and Health Information Technology Systems
The generation of evidence using real-world data sources in the past 20 years has created the opportunity to transform dental care and redefine dentistry’s role within the health care system. Parallel developments include the significant strides made in analyzing genomic information and the discovery of physical, functional, and biochemical biomarkers for disease. These developments offer new approaches for diagnosing and managing diseases instead of relying exclusively on symptoms and clinical assessment. Moreover, the increasing use of electronic devices to track daily activities, such as sleep, physical activities, and diet, is generating previously unavailable data regarding lifestyle behaviors.
The Patient Protection and Affordable Care Act of 2009 and the 21st Century Cures Act of 2016 (National Institutes of Health 2020b) have resulted in dramatic progress toward increasing electronic documentation of patient care in dentistry and medicine and improving provider, patient, and researcher access to electronic health information. Access to electronic health data has led to the development and evaluation of clinical decision support systems, an appreciation for new imaging modalities such as CBCT to monitor disease progression and treatment outcomes, and a better understanding of the natural history of diseases. However, the full potential of these advances can only be accomplished through evaluating and monitoring the quality of their data.
The dental profession has been mostly solo or small group practices. Consequently, there are as many different ways of recording patient encounters as there are practices, and there has been no consensus on a standardized method of data entry into EHRs. Furthermore, data are entered differently at each dental school, and how information is gathered across schools varies, which makes data mining challenging. A data-sharing framework has yet to be developed that allows oral health professionals to send their data to a curated data warehouse.
The choice of EHR has had a limited impact on the way dental medicine is practiced. To date, 72% of U.S. dental practices use an EHR to capture at least some clinical information (Acharya et al. 2017). Momentum for improved utilization of EHRs is building as the dental profession begins to see the advantages gained from using big data in medicine. However, the need remains for a critical paradigm shift with respect to dental data, with more effective and structured pooling of data and consistent use of diagnostic codes.
Most oral health providers cannot imagine a world without EHRs, yet clinicians often struggle with the lack of user-friendliness of available programs (Jha et al. 2018). Dentists are faced with many unmet information needs when using EHRs, such as timeliness of access to information; quality of visual representations of dental problems; access to patient-specific, evidence-based information; and the accuracy, completeness, and consistency of patient records (Song et al. 2010).
Furthermore, there are unmet needs related to access to medical records, genetic data, proteomics data, microbiome data, and biospecimen data. Issues around privacy of medical and dental information also pose daunting challenges to effective data utilization.
Researchers who want to use the vast amounts of data collected in EHRs face other challenges: Clinical notes cannot easily be queried, and there are data quality issues, such as incomplete and missing information or inaccurate and inconsistent data (Song et al. 2013). Although there are many efforts in medicine to address the problem of data fitness for research projects (Zozus et al. 2014), dentistry has lagged behind when it comes to the adoption of standardized diagnostic terminologies (White et al. 2011) and the use of standardized risk assessment tools (Ismail et al. 2013).
Integrated Electronic Health Records
Progress with EHRs has been fueled by technologic developments, allowing for enhanced system features, as well as by greater depth of knowledge and the urgent need for these records. Specifically, today’s systems can provide tools for integrated care and quality care metrics across practice locations to meet growing population needs.
Following publication of the 2000 Surgeon General’s report on oral health, the Health Resources and Services Administration began to prioritize and fund medicine-focused federally qualified health centers (FQHCs) to initiate or expand dental operations; part of this expansion included funding for development of integrated EHRs. The following health informatics initiatives provide examples of translational research using EHRs to improve patient outcomes and advance the delivery of high quality, integrated care.
FQHCs are uniquely suited to serving large populations with oral health disparities because they understand the advantages of better integration across the medical, behavioral, and oral health domains and because their staffs have spent decades honing skills to eliminate barriers to care and conducting outreach to communities in support of population health objectives. Moreover, utilizing EHRs and a referral management module in FQHCs has the advantage of providing early patient triage and referral to cross-disciplinary providers.
Point-of-Care Clinical Decision Support Systems in the Electronic Health Record
Secondary use of data routinely collected at point-of-care settings in an integrated medical and dental care environment has improved understanding of oral and systemic associations (Acharya et al. 2018). For example, a growing evidence base supports a relationship between diabetes and periodontal disease driven by underlying inflammatory activity (Mealey 2006; Eke et al. 2012; Glurich et al. 2013). Few of the health systems that have EHRs have developed strategies and tools to support integrated care delivery models that might offer better outcomes for patients with diabetes (Shimpi et al. 2016; Glurich et al. 2018; Shimpi et al. 2018; Shimpi et al. 2019d). In the absence of glycemic index monitoring in the dental setting, engaging predictive modeling and artificial intelligence (AI) to create a clinical decision support tool has offered a solution for identifying at-risk individuals. Once integrated into the EHR, this tool sorts available clinical data and identifies at-risk patients in need of triage for further clinical evaluation.
Several challenges still exist for an integrated health care model. Oral health professionals sometimes struggle to fully understand patients’ underlying medical conditions because medical and dental records remain largely separate from one another. Physicians also miss critical diagnostic information when they ignore the craniofacial complex. Integrated medical and dental records help dental clinicians use medical information more effectively and make it much easier for all health care providers to understand a patient’s health and history at the point of care, which can lead to fewer errors and more individual-centered care (Figure 5). Health disparities in oral health are pervasive and compromise the ability to elevate health for all.
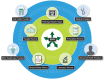
Figure 5
Integrated electronic health record (EHR).
Finally, greater exploration of the effectiveness of care delivery models that integrate oral health risk assessment tools, alerts, and reminders into medical settings is warranted. This may include integration of dental services within oncology practices to support patients’ oral health needs before, during, and following exposure to chemotherapy or radiation therapy; integration within pediatric practices to reduce the incidence and prevalence of dental caries through dental assessment and the placement of sealants and fluoride varnish applications; and integration into obstetrics to improve prenatal dental care. Health disparities in the context of oral health delivery present complex problems that rely on critical, multilevel analyses as described in the National Institute on Minority Health and Health Disparities Research Framework (National Institute on Minority Health and Health Disparities 2018).
Development of Quality Metrics and Dental Quality Dashboards
Using an EHR supports the development of quality metrics within a dental quality dashboard (Hegde et al. 2017). A dashboard’s summary format presents relevant patient data that respond to the needs of cross-disciplinary providers for patient management (e.g., diagnoses, treatment history, recent health events and procedures, medication exposure, insurance status, compliance with preventive care appointments).
A Dental Quality Analytics Dashboard (DQAD) is a web-based tool that has potential to facilitate data-driven performance reviews for use by dental providers and administrators. Its near-real-time visual analytic platform allows users to monitor, compare, and analyze key clinical, operational, and provider performance and productivity data to identify trends, set goals, and inform improvement activities across multiple providers or clinics. The application may also provide a summary view of the practice population, which may include information on socio-demographic characteristics and medical conditions. An example of an operational DQAD is shown in Figure 6, where sealant applications are tracked against a benchmark to help improve caries prevention activities across clinics (Nycz et al. 2020).
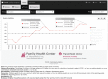
FIGURE 6
Screenshot from the Dental Quality Analytics Dashboard (DQAD).
Data Modeling
Health data analytics have grown exponentially during the last decade as electronic data have become available and accessible, and computing power has become cheaper. Today, there is no shortage of machine learning algorithms and deep learning models capable of analyzing large volumes of data of any type within a few seconds.
Multilevel models for health care data promote effective and interoperable biomedical information systems and services. For example, the Unified Medical Language System (UMLS) of the National Library of Medicine gathers several health and biomedical vocabularies as well as related items to facilitate interoperability between computer systems (National Library of Medicine 2021d). Additionally, it supports some widely used database models and multiple relational database management algorithms, including MySQL (open source) and Oracle. Computer modeling of data types contained in EHRs (biospecimen, imaging, clinical, genomic, and metadata) now includes descriptive data modeling for data processing and predictive data modeling for in-depth analytics.
Descriptive data modeling offers a system for data analytic strategies. Logical and physical data modeling defines and implements the structures of the different data elements of oral health information, setting the relationships and dependencies between them, such as functional, connectivity, and traceability relationships. The descriptive data modeling output explains the data using a structured form such as clustering or network analysis.
Descriptive modeling studies have suggested exciting associations between oral health and disease and phenotypic measures, but these findings often are not generalizable to other samples. Categorizations using descriptive models of oral conditions—temporomandibular disorders and facial pain; cleft lip, cleft palate, or both; dental caries; periodontal disease; and oral and pharyngeal cancer—often fail to achieve stated objectives because of the complex nature of clinical presentations and clinical progression, which can make the responses to therapeutic interventions unpredictable (Slavkin 2018).
Geometric or spatial descriptive models are used to represent craniofacial geometric or spatial relationships. Such 3D surface and volumetric texture models are used in dentistry to diagnose and plan treatment, assess treatment outcomes, and create physical models for 3D bioprinting of anatomy, appliances, maxillofacial grafts, and tissue engineering scaffolds. Three-dimensional geometric models that correlate facial morphology with genomic data show great promise in revealing underlying pathology. Utilizing a meta-analysis of genome-wide association studies, a complex pattern of coordinated genomic variants has emerged that governs variation in facial structures (White et al. 2021). Large-scale statistical modeling is required to control for multiple comparisons so that these studies can help provide a better understanding of the links between facial morphology and the genome.
Expert diagnostic systems driven by algorithms, that is, AI, may enhance diagnostic processes when utilized for decision support (Cabitza et al. 2017). It is imperative that researchers, clinicians, and the public engage in dialogue to explore data science’s ethical challenges and the interpretability and applicability of the underlying algorithms (Obermeyer et al. 2019). Unambiguous interpretation of the algorithms used in health care data gathering and analysis can ensure that individuals are treated equitably regardless of their race, gender, or ethnicity. Algorithms that determine treatment options, resource allocation, and prognosis of disease must be transparent to facilitate bioethical considerations and to promote public acceptability.
Teledentistry
An overview of U.S. teledentistry (Nichols 2019) describes the progress of this technology. The emergence of COVID-19 in the United States in 2020 and the resulting limitations on in-person care have demonstrated the value of telehealth, resulting in additional policies and applications being rapidly adopted. Most teledentistry functions as a public health service meant to address access-to-care issues, with a focus on risk assessment and prevention. The Center for Democracy and Technology now has specific codes for teledentistry that facilitate filing claims. By the last quarter of 2018, 49 states offered reimbursement for live video encounters, and 11 states reimbursed for store-and-forward (in which video interaction is recorded and viewed later, rather than live). A number of states now have policies allowing public health insurance reimbursement (Medicaid) for teledentistry: Arizona, California, Georgia, Minnesota (for children, pregnant women, and adults [limited benefits]), New York, North Carolina (synchronous only), Tennessee, and Washington (Center for Connected Health Policy 2020).
Teledentistry faces challenges before it can become a viable and widespread adjunct to mainstream clinical dentistry, however. These challenges include dissimilarities and conflicts in state and federal laws, reimbursement limitations, and concerns about data quality and security. Because technology can cross state borders, interstate provider licensure transferability is a key issue. Ensuring privacy and data security has become increasingly important.
Learning Health Care Systems
A learning health system (LHS) is a health care system that “learns.” In a LHS, knowledge generation is so embedded into health care practice that it becomes a natural product of the health care delivery process, leading to continual improvement in care (Institute of Medicine 2007). The LHS concept was virtually unknown in 2000. The LHS vision is more than a concept; organizations, networks, regions, and nations are actively working to become LHSs. Prominent health care systems (Ovretveit et al. 2016) and academic health centers (Pronovost et al. 2017) are launching LHS initiatives across the United States, and organizations are routinely coming together to form learning and improvement networks (Califf et al. 2016) with support from recognized public and private funders (Selby et al. 2013).
At a national level, the federal Office of the National Coordinator for Health Information Technology has made it an objective to connect LHSs across the country into a nationwide LHS by 2024 (DeSalvo and Galvez 2015). Initiatives at the Agency for Healthcare Research and Quality (Bindman 2017) and the Patient-Centered Outcomes Research Institute (Selby et al. 2013) complement and amplify this work. Globally, other countries are declaring their intentions to form nationwide LHSs (The Learning Healthcare Project 2021; Boes et al. 2018), and international networks continue to proliferate as well (Ethier et al. 2017).
The ethical, legal, and social implications of LHSs require increased attention (Platt et al. 2018), but daunting challenges exist related to cultivating the data, disseminating the knowledge, and acknowledging their benefits. A major benefit of national-scale LHSs is rapid and effective pharmacovigilance. For example, in a network the size of the U.S. population, post-marketing drug safety signals could be rapidly detected in a few weeks rather than multiple years, preventing unnecessary morbidity and deaths (Friedman et al. 2015). The underutilization of the ability of data to inform best practices and maintain optimal patient safety is an ongoing concern.
Implementation Science
Although the overall field of implementation science has grown steadily, implementation research in oral health has lagged (Clark and Ducharme 2016; Slavkin 2017). A much more systematic approach that goes beyond reimbursement profiles is needed to influence public health policies and reduce health disparities. Understanding preferences and perceived barriers to delivery of quality oral health care by multiple stakeholders is important for appropriately tailoring and effectively implementing services (Gostemeyer et al. 2019). More research is needed regarding the process and structure of implementation, such as the use of surgical checklists (Remiszewski and Bidra 2019) and decision algorithms (Asa’ad 2019; Tonetti and Sanz 2019) for promoting systematic delivery of high quality care across populations. Training curricula also are needed to address social determinants of health and the ways that social factors affect treatment engagement, preferences, decision-making, and outcomes.
Like other health professions, dentistry has not sufficiently examined system characteristics known to promote effective adoption and implementation at the organizational or community level (Ho et al. 2019; Weintraub et al. 2019), including partnerships with other types of service providers or community organizations such as primary care clinics and schools (Zhu et al. 2019a; Zhu et al. 2019b). There also is a need to better understand the impact of local, state, or federal policies on the implementation, funding, and reimbursement of specific oral health practices or programs (Chi et al. 2019; Peck et al. 2019; Reynolds et al. 2019).
Evidence-Based Approach to Introduction of New Technologies into Clinical Applications
The transition from a clinic-centric, business-driven model to one that is patient-centered, that is, more focused on individualized approaches to patient care, is influencing current trends in clinical research. The National Institute of Dental and Craniofacial Research (NIDCR) supports research in implementation science through National Institutes of Health (NIH)-wide and institute-specific dissemination and implementation funding opportunity announcements. Funded provider-related studies have addressed, for example, the use of a clinical decision support tool to aid in prescribing opioid and non-opioid analgesics for pain management following dental extractions (U.S. National Library of Medicine 2018); Rindal et al. 2021); the use of sealants to arrest noncavitated carious lesions (de Assunção et al. 2014); the use of an EHR system to enhance care provided to an ethnic minority population (Northridge et al. 2018); and engagement in an organizational change model to decrease appointment no-show rates (U.S. National Library of Medicine 2017).
Many illnesses have symptoms that present in the mouth. This means dentists and hygienists can help recognize early signs of disease, as well as spot life-threatening conditions such as diabetes and oral cancers at the earliest stage because they see many patients on a routine, rather than problem-driven, basis. Early detection improves the patient’s prognosis and helps control costs. For example, one medical insurance company conducted a 3-year retrospective study of 23,441 insured women, analyzing pregnancy outcomes and dental care using the health system’s combined medical-dental database. Mothers who received preventive dental treatment while pregnant had 25% lower preterm birthrates and 34% fewer incidents of low birth weight (Albert et al. 2011). However, this conflicts with the results of another NIDCR-funded study examining women in the second trimester of pregnancy that found no effect (Offenbacher et al. 2009).
The 21st Century Cures Act (U.S. Food and Drug Administration 2020c) has significantly modernized the field of clinical research. This Act recognizes the need to expedite the development of investigational medical products (drugs, biologic products, and medical devices) and implement faster and more efficient approaches to patient care. The Act provides the legal framework enabling the FDA to include patients’ perspectives in developing investigational medical products.
Implementation science as a field has seen significant growth in the past 2 decades (Norton et al. 2017), including development of guidelines and assessment tools for interventions appropriate for diverse populations, as well as models of community partnership and stakeholder engagement to ensure use of evidence-based practices (Damschroder et al. 2009; Barrera et al. 2013; Stirman et al. 2013; Anderson et al. 2015; Napoles and Stewart 2018). Innovative study designs that test components of complex interventions (Collins et al. 2016) were developed as well as system science approaches that examine the effectiveness of evidence-based practices at the population level (Green 2006). Finally, research methods such as hybrid effectiveness-implementation designs (Curran et al. 2012; Landes et al. 2019) have been developed to accelerate progress through the research pipeline from efficacy through implementation research when used appropriately.
Practice-Based Research Networks
To catalyze the development of dental practice-based research networks (PBRNs), NIDCR funded three regional dental PBRNs from 2005 to 2012 and is supporting a single, unified PBRN (the National Dental PBRN) through 2026. By the end of their initial funding period, the regional PBRNs had conducted numerous studies with thousands of patients and hundreds of practitioners. Studies investigated numerous topics using a broad range of study designs, demonstrated rigor and impact on clinical practice, and showed that dental practitioners could effectively contribute to every step of the research process.
The National Dental PBRN has made meaningful progress in better understanding clinical issues related to oral cancer, sensitive teeth, and root canal treatment, among other oral health conditions. For example, a study of cracked tooth syndrome in 2,858 individuals revealed that a large proportion of these teeth are asymptomatic and may remain stable, with little progression of cracks or symptoms during a year of follow-up (Hilton et al. 2020).
Delays in study implementation as a result of institutional review board requirements and slow initial recruitment of practitioners were early challenges in the development of oral health PBRNs. Many lessons were learned to inform future recruitment (Gilbert et al. 2011; Mungia et al. 2018). Oral health PBRNs have since demonstrated that practitioners from a broad array of practice settings and geographic regions will readily contribute research ideas and participate in studies. Whether and how regional networks of clinicians interface with dental trainees and faculty and whether this transfer of knowledge in clinically relevant areas is integrated into dental research environments are additional challenges the National Dental PBRN faces. Although the nature of PBRN research presents many challenges, these can clearly be overcome when managed appropriately for the improvement of practices and care of patients.
Considerations and Challenges in Clinical Research
Important advances have been made over the past decades through the benefit of large clinical research trials. Such trials are typically supported by private companies (mostly pharmaceutical), government, nongovernment organizations, or are self-funded (e.g., universities). The potential for advances can be tempered by the expense of engaging in clinical trials when sponsorship for large trials is mostly limited to some private entities or governmental funding opportunities. Because the cost of a trial is closely related to the length and number of follow-up visits occurring within a trial, it is important to support robust, well-designed, and well-powered studies to yield useful information for clinical care. Additional strategies to ensure stewardship of support are human participant safety oversight, clinical study monitoring, transparency, and timely reporting of findings.
It can be challenging to recruit participants to studies, and study generalizability may be limited by the lack of representativeness on the basis of, for example, age, race/ethnicity, gender, and even other demographic characteristics such as socioeconomic factors and location of residence. In order to identify causative underpinnings of oral and craniofacial diseases and develop new therapeutics for the treatment of chronic conditions affecting this region, longitudinal studies that are incredibly demanding in terms of years of investigational investment are needed.
Research Workforce, Training, and Education
Education and Training
A number of activities in the past 20 years have addressed strengthening and sustaining a robust and diverse research workforce in oral health science. The NIH Common Fund was initiated in 2004 to address emerging scientific opportunities and pressing challenges in biomedical research and research training, including the development of clinician-scientists. The Common Fund includes programs to enhance training opportunities for early-career scientists to prepare them for a variety of career options. The Common Fund’s Diversity Program Consortium aims to enhance diversity in the biomedical research workforce by engaging, training, and mentoring students; enhancing faculty development; and strengthening institutional research training infrastructure to enhance the participation and retention of individuals from diverse backgrounds in biomedical research careers (National Institutes of Health 2021a).
During the past 20 years, academic dentistry has undergone several major reforms promoted by the American Dental Education Association (ADEA) that have focused on improving health care providers’ overall effectiveness. Exposing future oral health professionals to research can have an impact on their educational experiences. However, related outcomes are not well measured, and approaches vary among institutions (Polverini and Krebsbach 2017). Various training schemes supported by NIDCR and other stakeholders and institutions, such as combined Doctor of Dental Surgery (DDS) and Doctor of Philosophy (PhD) programs and targeted research development programs, are designed to promote entry into academia, but these have fallen short of anticipated outcomes, as shown in Figure 7 (D’Souza and Colombo 2017).
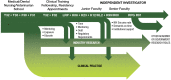
FIGURE 7
Physician-Scientist Workforce training pipeline.
The current demand for dental school faculty and research scientists is not being met. The ADEA task force report on the dental school faculty workforce showed that the number of vacant, budgeted, full-time faculty positions rose from 181 in 1993 to 374 in 2005 (Wanchek et al. 2015). A subsequent decrease to 252 in 2014 was primarily the result of permanent elimination of vacant positions (Garrison et al. 2014) as dental schools managed the shortage by adjusting curricula, increasing the teaching load of existing faculty, and hiring additional adjunct faculty. If faculty retirements and a faculty turnover rate of 33% every 5 years are factored in, it has been suggested that dental schools across the country need 208–218 new faculty members each year (Kennedy 1990). There is a critical need to create and sustain a viable pipeline of dental faculty (Wanchek et al. 2015).
Dental schools currently fail to attract more than 0.3% of their graduating seniors to academic careers (Istrate et al. 2021). Although some programs have been addressing this issue at a local level (Horvath et al. 2016), dental education in the United States now depends on the influx of foreign-trained academics to replace aging faculty. Forty percent of faculty at academic dental institutions are more than 60 years of age (Contreras et al. 2018). U.S. dental public health residency programs admit qualified international dental school graduates and have high percentages of foreign-trained dental graduate applicants and residents. During the 2019–2020 academic year, 62% of all dental public health residents were foreign trained (American Dental Association 2020).
The NIH Physician-Scientist Workforce Working Group Report identified challenges for clinically trained investigators in pursuing research careers, including educational debt as a result of high educational costs, as well as a long period of clinical and research training, which create a vulnerable transition period between research training and independent academic research careers (National Institutes of Health 2014). Competition for prestigious NIH funding has become increasingly intense (Ginther et al. 2011). The average age of first receipt of a major NIH independent research grant (the R01) rose from 36 years old in 1980 to 45 years old in 2013 (Daniels 2015), which can lead to several challenges facing younger researchers in academic settings.
The consensus study report, The Next Generation of Biomedical and Behavioral Sciences Researchers, of the National Academies of Sciences, Engineering, and Medicine, prepared in 2018 by the Committee on the Next Generation Initiative Board on Higher Education and Workforce Policy and Global Affairs, defined the core challenges that undercut the vitality, promise, and productivity of the biomedical sciences (National Academies of Sciences, Engineering, and Medicine 2018a). The percentage of tenure-track opportunities among all positions in academic dentistry has declined steadily (Chmar et al. 2006). Given that vacant faculty positions averaged 257 per year between 1994 and 2015, graduates from the existing 22 oral sciences PhD programs who entered U.S. academic institutions could have filled only 3.6% of the annual vacant dental faculty positions during this time period (Herzog et al. 2018). If the annual number of graduates continues at this low rate, the United States is at risk for a significant compromise in the size of the available academic workforce.
There are no analytic data on trends in enrollment for PhD programs or the placement of their graduates. This lack of program-specific data for oral sciences PhD programs has made it difficult to gauge how many students optimally should be trained to support the dental profession’s education and research mission. The report, Oral Sciences PhD Program Enrollment, Graduates, and Placement: 1994 to 2016, was the first attempt to summarize oral sciences PhD program outcomes. According to that report, oral sciences doctoral programs have enrolled more women (54.7%) than men (44.5%). Race or ethnicity among these enrollees was reported as White (39.1%), Asian (34.7%), Hispanic (7.1%), Middle Eastern (5.2%), Black (2.9%), Indian (2.4%), and unknown or not reported (8.6%) (Herzog et al. 2018). Clearly, diversity in these programs is less than optimal. Both the growth in student debt and the widening income gap between dental practitioners and academic professionals make recruiting and retaining dental faculty challenging. Although there is a small group of dental students interested in teaching, very few structured mentoring programs exist to guide them in pursuing academic career goals.
The financial pressures on dental schools often lead them to increase emphasis on clinical revenue generation and decrease their emphasis on research (Wanchek et al. 2015). Consequently, the pool of research faculty available to act as mentors for early-career dentist-scientists has diminished. In addition, the amount of student debt upon graduation likely presents a barrier to dentists pursuing careers in academic dentistry.
NIDCR maintains strong partnerships with dental schools and other institutions that have a vital role in educating and training oral health researchers (Feinberg et al. 2015; Ferland and Winstanley 2017; Herzog et al. 2018). However, the institute’s total investment in dental schools decreased during the past 15 years (Ferland and Winstanley 2017). These data align with long-standing concerns about dental institutions’ diminished research and research training capacities, especially in training the dentist-scientist research workforce (D’Souza and Colombo 2017). This decrease in support compromises dental schools’ abilities to generate new science to prevent and treat dental, oral, and craniofacial diseases (Feinberg et al. 2015; Polverini and Lingen 2017; Formicola et al. 2018). When adjusted for inflation, there also has been a steady decrease in NIH funding since the doubling of the NIH budget (Kaiser 2019). One of the consequences of these decreases is a hypercompetitive environment that has too many researchers vying for limited resources and discourages students and postdoctoral scientists from continuing academic research careers (Alberts et al. 2014).
Faculty impediments to conducting research include the paucity of protected time for research and inadequate formal mentoring and career guidance programs for early-stage faculty. Disciplinary silos continue to determine requirements and curricula, with little opportunity for interdisciplinary exploration, creation of scientific networks, and experience with diverse methods and working styles in research groups. In addition, little time and few opportunities exist for development of professional skills on scientific writing, public communication of science, leadership, or learning how to manage projects and staff.
Redoubling Commitments to Diversity and Inclusion
In addressing the preparation of the future scientific workforce, committed action to diversity is essential. In 2018, more than half of first-time graduate students (master’s and doctoral level) were women (Okahana and Zhou 2019), and women received more than half of the doctoral degrees in 2017, in fields related to biological and biomedical sciences, health sciences, and public health (National Academies of Sciences, Engineering, and Medicine 2018a). In contrast, reports indicate that in a number of fields related to science, technology, engineering, and mathematics (STEM), including the physical sciences and engineering, no real progress has been made in recruiting underrepresented minority students (National Academies of Sciences, Engineering, and Medicine 2018b; National Science Foundation 2019), and the number of minority doctorate recipients is far below minorities’ representation in society (National Science Foundation 2019).
Critically, the National Center for Science and Engineering Statistics lists hundreds of fields and subfields of study, yet it does not include dentistry or oral health sciences among them, making it difficult to estimate the progress, or lack thereof, in developing a diverse cadre of scientists in this area. A 2019 article in The Atlantic noted that “in more than a dozen academic disciplines—largely STEM related—not a single Black student earned a doctoral degree in 2017” (Harris 2019). If this trend continues, the diversity and inclusiveness of faculties and leadership will continue to fall. Creating safe and inclusive environments for individuals of different racial and ethnic groups, genders, and socioeconomic status, as well as those with disabilities is an ongoing challenge that requires strategic consideration and fresh approaches to diversifying the academic workforce in oral health.
NIH also has funded efforts to support diversification of the scientific workforce (National Institute of General Medical Sciences 2021a). Examples include the Building Infrastructure Leading to Diversity (BUILD) initiative and the National Research Mentoring Network (NRMN). The BUILD initiative consists of linked grants issued to undergraduate institutions to implement and study innovative approaches to engaging and retaining students from diverse backgrounds (National Institute of General Medical Sciences 2021b). The NRMN is a nationwide network of mentors and mentees from all biomedical disciplines relevant to the NIH mission of providing support to individuals spanning undergraduate education to early-faculty careers.
A special advisory group to the NIH director on diversity in the biomedical workforce was convened in 2011 (Working Group on Diversity in the Biomedical Research Workforce 2012). In addition, the topic of diversity and inclusion in the dental research workforce within the United States was covered in a special issue of Advances in Dental Research and discussed by D’Souza and colleagues (D’Souza et al. 2017; Ioannidou et al. 2019). Promising advances in these programs led to new initiatives in 2018 to sustain progress and identify methods and interventions to support sustainable models for enhancing diversity in biomedical research.
Emerging Challenges to Oral and Craniofacial Health
New challenges are continually arising that may have an impact on the oral and craniofacial complex or the manner in which oral health care is provided. Examples include environmental toxins, endocrine disruptors such as bisphenol A (and other derivatives), phthalates, lead, arsenic, and mercury. Exposure to chemicals and toxins can affect the building blocks of teeth, a process that begins during prenatal development (Jedeon et al. 2013; Andra et al. 2015).
Infectious pathogens not only impact overall health, but they also can affect oral health and disrupt dental practice. Following the World Health Organization’s declaration of an international pandemic because of COVID-19 and the implementation of emergency health measures in the United States, dental professionals were encouraged or mandated to limit their practices to emergency services. Ongoing clinical and basic research across the biomedical enterprise, including oral health sciences, were disrupted as well. Overnight, new questions emerged about the safety of dental practices relative to viral transmission between patients and clinicians. An article published very early in the pandemic described how one dental school approached a limited emergency-care operation during the outbreak (Meng et al. 2020). Previously in 2004, an article had summarized the impact of an earlier coronavirus epidemic, SARS, on dental practice, but its limited distribution did little to change dental practice, because the era of human immunodeficiency virus/acquired immunodeficiency syndrome (HIV/AIDS) had already instituted rigorous infection controls (Samaranayake and Peiris 2004).
In contrast to responses to HIV/AIDS and SARS, COVID-19 brought dental care and research to an almost total halt, creating unprecedented challenges for oral health and highlighting questions that had not previously been asked. In fact, because even the profession had not agreed upon the definition of emergency care, the American Dental Association had to distribute guidance on what constituted a dental emergency. New questions arose about how to know when and how dentistry was safe to practice. These questions included: “Do we need to consider point-of-care diagnostics to determine whether patients or clinical providers have transmissible infectious diseases?” “What will salivary diagnostic utilization look like in the future?” “What is the immunity profile of such a highly infectious novel agent and how should we gather and use such data?” and “How do we determine the course of disease and viral load for patients?” (To et al. 2020).
A continuing challenge for the profession will be how to embrace new technologies to reduce the spread of highly infectious agents such as SARS-CoV-2 and yet-unknown infectious organisms. Oral health research can play a vital role in this endeavor, as exemplified by the detection of SARS-CoV-2 in various sites of the oral cavity (Huang et al. 2021). However, this challenge is not limited to the capability of responding quickly to orchestrate scientific research to identify potentially harmful agents, but extends to the ability to develop and implement science-based, new approaches that lead to the provision of safe care to patients within the framework of these emerging threats.
Chapter 3. Promising New Directions
New scientific approaches provide opportunities to critically review previously held ideas about important health issues and to update the dental profession’s evidence base. The U.S. health care system faces increased challenges at the same time it offers new opportunities. There is an opportunity to use science and technology to spark innovation in health care delivery, with the goal of improving oral health and realizing better treatment outcomes at lower cost and greater convenience to patients. Improving the oral and craniofacial health of every American is now within reach.
Foundational Sciences in Oral Health
Omics/Gene Editing/Single-Cell Technologies
Emerging clinical innovations have already begun to employ the tools of the omics era. Notably, there have been a number of successful genome-wide association studies of phenotypes, including orofacial clefting (Leslie et al. 2016; Leslie et al. 2017; Haaland et al. 2018), dental caries (Wang et al. 2012; Haworth et al. 2018), periodontitis (Divaris et al. 2013; Haworth et al. 2018), tooth eruption and development (Geller et al. 2011; Fatemifar et al. 2013), and orofacial pain (Randall et al. 2017). There also is a long tradition of researchers utilizing microbiomics (Dewhirst et al. 2010; Escapa et al. 2018), metabolomics (Foxman et al. 2016), and metagenomics, notably focusing on dental caries and periodontal disease.
There is a major international effort to develop databases to integrate genomics into health care (Stark et al. 2019) by linking nationwide electronic health records (EHRs) and obtaining DNA samples. At least 14 countries have begun such vital endeavors (Stark et al. 2019). In the United States, the program is known as the All of Us Research Program (National Institutes of Health 2021b) and has a goal of engaging 1 million volunteers to reflect the nation’s diversity, including all life stages, health statuses, racial and ethnic groups, and geographic regions. All of Us and other endeavors, such as the National Cancer Institute’s Cancer Moonshot (National Cancer Institute 2020) and the 21st Century Cures Act (National Institutes of Health 2020b), hold great promise for the development of innovative prevention strategies, treatments, and data sharing. It is critical to integrate dental, oral, and craniofacial health phenotypes into these initiatives.
Applications of Genome Editing to Human Cells
We are in an era with access to a variety of highly versatile editing technologies that allow genetic material to be added, removed, or altered at locations across the genome. These technologies offer exciting routes to new therapeutic development by potentially allowing for correction of underlying genetic deficits that exist in a number of human diseases, as well as in microorganisms that compromise health. Several genome editing technologies may influence oral disease and congenital malformations, including zinc-finger nucleases, transcription activator-like effector nucleases, clustered regularly interspaced short palindromic repeats (CRISPR)-associated protein systems, and RNA editing (Baysal et al. 2017; Yu et al. 2019).
In the field of oral health, single-gene disorders, which are caused by mutations in a single gene, are targets of genome-editing technologies for research and therapeutic applications (Figures 8 and 9) (Patil et al. 2014). Many of these disorders feature craniofacial anomalies that can be prevented or reversed with replacement therapies that involve proteins as well as agonist small molecules and antibodies (Jia et al. 2017a; Jia et al. 2017b). Advances in genome editing technologies have raised hope for improving the clinical diagnosis, treatment, and outcomes for patients with craniofacial malformations associated with single-gene disorders (Neben et al. 2016; Yu et al. 2019).
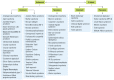
Figure 8
Working classification of single gene disorders with oral manifestations.
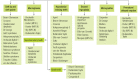
Figure 9
Single gene disorders with common oral manifestations.
In light of advances in human genome editing, it also is important to consider the potential of microbial genome editing. Although still in its infancy, this field has had some early proof-of-principle studies, including the design of a self-targeting CRISPR system in Streptococcus mutans—a primary causative agent of human dental caries—that could reduce the bacteria’s pathogenicity. Limited genetic tractability in many bacterial species is being addressed on multiple fronts with newer technologic advances in genetic engineering of bacteria (Cuiv et al. 2015; Johnston et al. 2019).
Single-Cell Technology Applications in Oral Health Research
In the emerging technologies for single-cell omics, single-cell analysis deepens our understanding of cell identity, diversity, development, and function in a way that bulk analysis cannot achieve (Junker and van Oudenaarden 2014). Combining comprehensive analyses of omics studies with single-cell resolution continues to highlight the extent, nature, and role of the cellular heterogeneity (diversity) that arises in organisms with regard to both health and disease (Shapiro et al. 2013; Wang and Navin 2015). By enhancing the ability to comprehensively define cell types and states, diseases with complex etiologies can be better conceptualized, providing more accurate diagnostic tools, prognostic biomarkers, and signaling pathways amenable to therapeutic targeting.
Microbiome/Inflammasome/Virology
The human microbiome has become an attractive drug discovery platform for new therapeutics (Milshteyn et al. 2018). There is increased recognition of the vast numbers of unidentified molecules produced by oral microorganisms that are likely to affect the oral microbiome (Edlund et al. 2017). Promising strategies to engineer and manipulate these communities are emerging. Several prebiotics, which are substances that potentially modulate the microbiome, are being investigated. For example, identifying individuals whose oral microbiomes are exceptionally robust and efficient at reducing lactic acid to protect against tooth decay could provide insight into unique preventive microbial communities. Scientists could identify persons who are genetically prone to periodontal infection but could potentially be protected by a healthy oral microbiome that keeps inflammation in check or limits keystone pathogens (Lamont et al. 2018). Such case studies would provide opportunities to uncover new therapeutic molecules made by unique biosynthetic gene clusters (Donia et al. 2014).
Basic and clinical research should incorporate evaluation of the microbiome, including clinical trials to evaluate new therapeutic regimens and engage scientists and dentists who have a wide range of expertise (Human Microbiome Project Consortium 2012a; 2012b). There also is a need to understand complex microbe-microbe interactions (Mark Welch et al. 2016; Stacy et al. 2016) and microbe-host interactions (Lamont et al. 2018).
Development of new tools would go far to advance microbiome applications. Gnotobiotic mice (animals in which all microorganisms are either known or excluded) colonized with a select group of human gut microbiota have been instrumental in determining the contribution of those microbiota to disease (Fitzgerald 1968; Kashyap et al. 2013; Smits et al. 2016). The establishment of a similar model would provide a powerful tool for evaluating contributions of the oral microbiome, help uncover new keystone players, and facilitate the development of more relevant microbiota-targeted therapeutics. Engineering of the microbiome in the oral cavity in situ remains challenging. Such microbiome engineering (Ronda et al. 2019) should enable the precision delivery of novel genetic and biochemical traits into the oral cavity and open a new frontier in synthetic biology.
Inflammation/Inflammasome
Inflammation diagnostics have advanced, and specific inflammasome sensors are being explored. Interleukin (IL)-1 blockage is an example of a successful clinical translation of basic immunology research that has clinical applications for multiple inflammatory disorders, including periodontal diseases (Mantero et al. 2018). Excitingly, a number of inflammasome inhibitors (Khalafalla et al. 2017) have been reported.
Small molecules that target the inflammasome can provide specificity and cost-effectiveness (Swanson et al. 2019). Several small-molecule inhibitors, developed to have an impact on inflammasome, IL-1, and IL-18 production (Swanson et al. 2019), have demonstrated therapeutic potential.
In addition to targeted approaches, microbiome modulation, gene therapies, and autotherapies hold promising therapeutic avenues for inflammasome advances (Gomez and Nelson 2017; Polak and Shapira 2018). The specialized proresolving mediators that control immune cells have potential as therapeutics for inflammatory diseases and are entering human clinical trials for gingivitis and periodontitis treatment (El Kholy et al. 2018). This new category of molecules includes lipoxins, resolvins, maresins, and protectins (Serhan et al. 2000; Levy et al. 2001). Their capacity for clinical impact is promising yet underexplored. With an increase in the number of individuals affected by dental and craniofacial inflammatory conditions, as well as aging populations, there will likely be a greater need for the development of new inflammasome-targeted diagnostics and therapeutics that could also provide better systemic health outcomes (Swanson et al. 2019).
Regenerative Medicine, Dental Materials, and Bioinspired Materials
Antimicrobial Materials
The addition of antimicrobial materials to existing dental materials offers an opportunity to improve their longevity and promote better oral health. Materials are being developed that incorporate antimicrobial agents to kill potentially harmful bacteria on contact or prevent them from forming biofilms (Cocco et al. 2015; Stewart and Finer 2019). One example is a biomaterial that releases fluoride into surrounding areas (Hafshejani et al. 2017). Commercial products have been developed for restorations that provide a contact-kill mechanism to keep bacteria from colonizing the area between the restoration and remaining tooth where cavities are thought to begin (Imazato 2009; Makvandi et al. 2018). Continued research into such materials is likely to lead to improved dental restoratives that preserve tooth structure and reduce the need for later intervention.
Remineralizing Materials
New remineralizing materials hold great promise for reducing restorative failure and improving oral health. Many materials have become commercially available that have the potential to promote mineral formation in the oral cavity, either through the addition of fluoride or the incorporation of calcium phosphate, calcium silicate compounds, or bioactive glasses (Taha et al. 2017). Some new materials are designed with nanotechnology-based materials that simultaneously promote remineralization and demonstrate antibacterial effects (Cheng et al. 2015; Zhang et al. 2017). These types of materials currently are primarily used for lining the deeper portions of a dental restoration for direct pulp capping (Paula et al. 2018). Promising results have been shown in promoting dentin material using new and already existing materials, such as calcium hydroxide and mineral trioxide aggregate (Didilescu et al. 2018). Continued work in this area will undoubtedly result in a host of commercial products that can be used throughout dentistry to provide more predictable responses to decayed tooth tissue treatment.
Regenerative Materials for Tooth Replacement and Alveolar Bone Repair
The use of advanced scaffold designs that can guide the spatiotemporal requirements for periodontal regeneration, which is key for new periodontal attachment formation, has the potential to significantly improve therapeutic outcomes (Ivanovski et al. 2014; Vaquette et al. 2018). In regenerative endodontics, there is strong potential in materials, typically built on resorbable polymer scaffolds (Fukushima et al. 2019; Patel et al. 2019), that can be implanted into the removed, damaged tooth pulp to restore healthy form and function. Investigators are exploring the development of materials from three-dimensional (3D)-bioprinted scaffolds implanted with undifferentiated stem cells that can generate vasculature, nerves, and structural cells capable of functioning in much the same way as a natural, healthy tooth (Athirasala et al. 2017).
3D Bioprinting and Additive Manufacturing
Emerging research into the use of 3D bioprinting technologies (also known as additive manufacturing) in dentistry includes the development of bioinspired microstructural arrangements, such as topology optimization (TO). This process maximizes physical performance by optimizing the structural arrangement within the design of an object composed of one or more materials (Bendsoe and Sigmund 2004). Initially, TO was used to modify the macroscopic geometries of objects designed using homogeneous materials (Sigmund 1994), but it is now possible to work at a much higher resolution when combining different materials into designs that incorporate specific microstructures suited to a desired performance.
Use of 3D printing is growing rapidly across many sectors. This innovation encompasses a suite of technologies for fabricating parts directly from 3D digital models. The technology’s power lies in its ability to produce high-value, complex, and individually customized parts. The integration of the different types of printable materials, as well as better software and hardware, has dramatically expanded the potential for these approaches (Ligon et al. 2017; Harun et al. 2018).
The quality of 3D products, in terms of speed of production, dimensional control, strength, and biocompatibility, is increasingly meeting the needs of dentistry. At the same time, the capabilities of scanners to generate, digitize, store, and manipulate 3D patient data in a cost-effective fashion continue to grow. In the future, dental offices will be able to produce patient-customized specific parts, such as implants and dentures, by combining the digital powers of 3D scanners and 3D printers (Figure 10) (Galante et al. 2019). This shift will have implications for clinical practice, researchers, materials producers, and equipment manufacturers—with ultimate benefit to the patient (Bhargav et al. 2018; Oberoi et al. 2018).
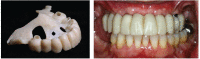
FIGURE 10
3D Printed denture teeth processed and seated on supporting dental implants. Notes: Digital denture designed with CAD/CAM software and denture teeth 3D printed. Dentures can be designed in less than 20 minutes and printed in as little as 30 minutes.
Nanotechnology
The utilization of nanotechnologies in dentistry is in a young, yet promising, stage of emerging discoveries and breakthroughs. One encouraging new direction uses intracellular nanodelivery tools, such as polymeric or lipid nanoparticles, nanoneedles, electroporation, and other similar methods, including inorganic nanoparticles, to manipulate cellular behavior in situ (Stewart et al. 2016). This will form the basis for the development of next-generation vaccines, precision therapies, and patient-specific regenerative strategies that take advantage of cell reprogramming, differentiation, and tissue engineering and regenerative medicine. Another area of development is nanotheranostics, which employs minimally invasive techniques using nanoscale materials simultaneously for early-stage disease diagnostics and therapy (Cheng et al. 2017). Two more areas that deserve attention are the rapidly emerging fields of nanofabrication and nanorobotics in medicine, as well as exosome engineering (Yim et al. 2016).
Stem Cell Biology
Innovative stem cell approaches should allow first-in-human clinical trials in dentistry for repair of bone defects around teeth, dental implants, cleft and orofacial repairs, and regenerative endodontics. A promising area of development is allogeneic stem cell therapy, which can use stem cell banks as ready sources for stem cells on an as-needed basis. Better coordination among scientists, clinicians, industry, federal regulatory agencies, and reimbursement entities would accelerate the potential for stem cell biology to provide clinical advances.
Science and Technology for Practice
Genetics- and Genomics-Based Precision Health
Technologic advances have profoundly decreased the cost of sequencing genomes, potentially enabling genetic information to be generally available to guide clinical care (Chiu and Miller 2019). During the next 10 years, genetic and genomic information will increasingly have an impact on dentistry, as they have in medicine, and will be used with other data, including diet and lifestyle, to inform clinical prevention and treatment decisions. Genomic information about an individual will increasingly be used to inform clinical care decisions (for susceptibility, diagnostic, or therapeutic decision-making) as development of national genome-medicine initiatives continue to integrate genomic, epigenomic, environmental, behavioral, and other information in a meaningful way to fulfill the promise of precision health care (Stark et al. 2019). These projects’ capacities to integrate data from millions of individuals will provide the power to identify and validate clinically useful data.
Advances in Laser- and Light-Based Imaging Technologies
Higher-performance imaging devices operating at longer wavelengths beyond 1,000 nanometers (nm) will likely become commercially available in the near future as the prices of germanium and indium gallium arsenide sensors continue to decrease. The highest contrast of demineralization on tooth surfaces occurs at longer near-infrared (IR) wavelengths greater than 1,000 nm, owing to reduced scattering in sound tissues and higher water absorption (Chung et al. 2011; Fried et al. 2013). Higher water absorption also can be exploited to yield high contrast of demineralization and dental calculus on root surfaces at wavelengths beyond 1,450 nm (Yang et al. 2018). Stains can easily be differentiated from actual demineralization, which is not possible at wavelengths less than 1,200 nm (Chung et al. 2011). At wavelengths greater than 1,300 nm, near-IR imaging is more sensitive than radiography for the detection of lesions on both occlusal and proximal tooth surfaces in vivo (Simon et al. 2016).
Optical coherence tomography (OCT), a noninvasive technique for creating cross-sectional images of internal biological structures (Bouma 2002), can be used to measure the reflectivity within dental hard tissues to a depth of up to 3–5 millimeters (mm) in enamel and 1–2 mm in dentin, with an axial (depth) resolution exceeding 10 micrometers. Commercial OCT systems are on the horizon to monitor demineralization (Fried et al. 2002; Louie et al. 2010). High-speed OCT systems are now available that can make an image of a tooth in less than a second. In the near future, systems will likely have scanning rates 10–100 times faster than today. Dedicated dental OCT imaging systems are under development and should be available in the next few years.
Neuroimaging-Based Technologies for Managing Orofacial Pain
Researchers have started to analyze the neurologic signatures of pain using neuroimaging. Portable neuroimaging devices with technical benefits similar to functional magnetic resonance imaging (fMRI) have been developed. Functional near-infrared spectroscopy (fNIRS) detects concentration variations of oxyhemoglobin and deoxyhemoglobin, such as blood-oxygen-level-dependent signal in fMRI, by measuring the absorption of near-IR light at wavelengths between 700 and 1,000 nm. fNIRS, akin to fMRI, can be used to study functional brain activity in the clinical environment. The fNIRS device has the portability, interface, and compatibility necessary for ferromagnetic and electrical environments, so it can be used in many clinical settings to monitor patients’ functional brain activity (hemodynamic responses) and functional connectivity. Future dental offices may have the opportunity to localize orofacial pain with much greater accuracy using less invasive techniques and smaller, easier to use devices to improve diagnoses related to oral pain.
New Therapeutic Approaches in Dentistry
Biomarker studies have led to the discovery of new drug targets such as microbiome-modulating compounds (C16G2) or anti-inflammation reagents (resolvins and lipoxins) to treat and prevent dental caries and periodontitis. Various new microbiome-reengineering approaches, such as probiotics, prebiotics, and phage therapy, have promise.
Innovative technologies have been reported for dental caries reduction through an oral microbiome-based preventive approach, such as topical use of the amino acid arginine (Bijle et al. 2019). Another new direction is the application of silver diamine fluoride to a decayed dental surface to stop the progression of a carious lesion (Horst and Heima 2019). Although the use of silver diamine fluoride to inhibit dental caries is not a new therapeutic approach and has been extensively used in other countries, there has been renewed interest in the United States in this product.
Areas that hold great promise for the treatment of head and neck cancers include immunotherapeutics that expand tumor-specific T cell mechanisms and radiation sensitizers that utilize DNA-damage repair agents (Heath et al. 2019; Manukian et al. 2019). Diagnostic and therapeutic precision approaches in the treatment of head and neck cancers also are under intensive investigation on the basis of the delineation of key signaling pathways, and they offer promising therapeutic opportunities (D’Silva and Gutkind 2019). New strategies for stem cell based anticancer therapies are on the horizon that build on the key concepts of precision health (Wang and Aguirre 2018).
Robotics-Assisted Dental-Surgical Treatments
Modern diagnostic tools already described, in combination with artificial intelligence (AI)-assisted surgical robotics, are helping dental surgeons automate or augment complex procedures, such as implant surgery, crown preparation, and placement of orthodontic bands and brackets (Schwendicke et al. 2020; Shan et al. 2021). Similar systems are being used to train providers or allow them to practice clinical procedures. The ability to have these systems monitor and then coach new procedures, while simulating the real-world environment, helps dental professionals learn new techniques and increase proficiency.
Innovation in Preventing Oral Diseases
Emerging preventive-practice technologies are exciting and include novel intraoral cameras, innovative, noninvasive treatments of early carious lesions, and telehealth tools. The revolution of precision medicine and successful dental innovation stories will stimulate more research institutes and scientists to develop innovative technologies and attract more investment funds to support dental technology development.
With regard to diagnostic techniques, modern ionizing and nonionizing radiation techniques with 3D-imaging capacity may supplement traditional X-ray-based tooth imaging systems, when indicated. A downside of 3D-imaging overuse is the higher radiation exposure. Dental offices will routinely use high-resolution, multifunctional, intraoral cameras and scanners. Laser and Raman spectroscopy-based functional imaging systems and ultrasound and magnetic resonance imaging-based soft tissue scanning systems will allow dentists to better assess patients’ health statuses with of-the-moment physiological and pathologic information. Furthermore, innovative neuroimaging-based technologies could transform the brain into an objective target to visualize, measure, diagnose, and localize orofacial pain in real time in the doctor’s office. Key genetic and microbiome biomarkers for the early diagnosis and prevention of dental caries and periodontal disease could be analyzed in saliva and blood through laboratory testing, chairside instant tests, or even in vivo planted biosensors.
Technologies will enable the development of preventive- and digital-dentistry-focused personalized oral health care that can be fully integrated with personalized medicine using unified and cybersecure EHRs. Ultimately, these modern technologies will allow care to be accessed in innovative ways, through new clinical service models, teledentistry, mobile dentistry, integrated clinic and home care, and full integration of clinical care with payment and reimbursement systems.
Oral Disease Prevention at Home
Preventive agents and technologies also can be applied outside of professional dental practices. U.S. health care consumers have become more empowered as they seek to gain more control over their personal health, health finances, and health care experiences. Medical solutions and services are swiftly becoming mobile and direct-to-consumer. Smart devices already are monitoring daily blood pressure, glucose and heart rate, physical activity, nutrition patterns, and conditions such as atrial fibrillation. As consumers express desires to improve oral health, more widespread access to and use of technology for oral health solutions are beginning to make their way into the home.
Emerging solutions are focused on better educating consumers and caregivers about oral health, improving self-care through smart connected technologies that measure and track personal oral health data and advancing better at-home oral hygiene. Some examples include the development of educational and caries risk assessment apps, such as Brush Up and My Smile Buddy (Chinn et al. 2013). Several companies have toothbrushes that track brushing data to improve brushing technique, frequency, and efficacy and create a connection to the dental provider. Additional strategies involve using an intraoral biosensor platform to monitor saliva, initially focusing on measuring pH levels (Choi et al. 2017).
In addition, a healthy innovation environment is emerging around personal care and consumable products, such as toothpastes, rinses, floss, gums, mints, and lozenges. These innovations are intended to improve personal oral health by encouraging good oral hygiene, aiding in tooth remineralization, and influencing the composition and behavior of oral biofilms. As the costs of these technologies decrease with greater adoption and production, preventive care at home will have an important role to play across populations for maintaining good health and creating value for consumers, providers, health care sponsors, and communities.
Information and Data Science
Big Data and Electronic Health Records
Big data gleaned from EHRs and other databases should be analyzed electronically to project attributable risk for disease emergencies using data-mining approaches to develop predictive models (Glurich et al. 2017; Hegde et al. 2017). Moreover, integration of oral and craniofacial health with general health through an integrated EHR is critical and will support advances in understanding the physiological interactions between oral and systemic health and disease.
The creation of a collaborative environment across disciplines using EHRs supports high quality health care, research, and education and connects hospitals and health care organizations. Moreover, EHRs have substantial research potential through monitoring health events and evaluating outcomes using data generated from integrated health care systems. This, in turn, can synergistically improve our understanding of the relationships between oral health and overall health and well-being within real-world environments.
Dentists will increasingly help other medical professionals provide primary care to their patients. In many dental offices, recording of vital signs is routine. Screening for diabetes, atrial fibrillation, obesity, vaccinations, mental illness, risk of falls, substance abuse, domestic abuse, and other conditions normally screened for by primary care providers also could be routinely obtained within a dental office environment. The resulting data, residing in an integrated EHR, would be updated and acted upon in a more frequent, regular, and efficient manner. A unified record has a positive impact on population-based research by making it easier to conduct longitudinal studies with greater data fidelity. The study of various oral disease processes will be better understood in the context of the larger whole.
Using EHRs to create a collaborative environment across disciplines contributes to the common goal of providing access to state-of-the-art, high quality, and holistic health care for all segments of the population. One example of an integrated model pairs a large community center (Marshfield Clinic, see Section 4) with a center-dedicated research team. This model expands access to dental care and promotes improved quality of care by generating new knowledge and creating decision support tools that can be quickly translated into practice or used to guide future planning (Acharya et al. 2012). It holds great promise for advancing oral health and integrating oral, medical, and behavioral health in ways that accelerate progress toward improved oral and general health for the patients and communities served. Encouraging national promotion of this model would be beneficial for improving patient outcomes (Acharya 2016; Shimpi et al. 2016; Shimpi et al. 2019a). The Institute for Healthcare Improvement (2003) employs a model for improvement that includes plan-do-study-act cycles for rapid tests of change to emphasize multidisciplinary innovation and learning under an umbrella of improvement science. Such an approach could prove valuable on a platform of an integrated EHR.
Incorporating Social Determinants into Electronic Health Records
Another new direction for EHRs is their use of social determinants of health. Multi-level factors (at the child, family, and community levels) have been described conceptually for influencing oral health (Fisher-Owens et al. 2007; Lee and Divaris 2014). Social determinants of health may include an individual’s health knowledge and behaviors, social resources, social support, peer pressure, norms for healthy behaviors, and adherence to treatment plans (Braveman and Gottlieb 2014). The emerging popularity of social media has created virtual communities on the internet that can significantly influence individuals’ health behaviors and social determinants. Social determinants may be key contributors to patients’ decisions on whether to return to the dental clinic to complete treatment or to adhere to treatment at home.
Incorporation of patients’ social determinants into EHRs will improve dentists’ understanding and ability to predict challenges related to oral hygiene behaviors. It also will help dentists to identify patients who may need referrals, including for social interventions and personalized oral health care, particularly among vulnerable populations.
Some factors that may not be measured and noted in the EHR and that may affect dental outcomes are referred to as unmeasured confounders. For example, EHR data usually do not include the oral health education individuals receive from schools, communities, the internet, or peers, which influences their sugar consumption and oral hygiene. The instrumental variable method is a way to understand the effects of unmeasured confounders by using a variable that influences which treatment individuals receive but is independent of unmeasured confounders and has no direct effect on the outcome except through its effect on the treatment (Baiocchi et al. 2014). This method can be used with EHR data when there are concerns about both measured and unmeasured confounders.
In the context of research, investigators need to be cognizant of the multifaceted nature of health disparities, including levels of influence (individual, interpersonal, community, societal) and domains of influence (biological, behavioral, physical, sociocultural, and health care system) that are critical to understanding minority health as outlined by the National Institute on Minority Health and Health Disparities at the National Institutes of Health (NIH) (National Institute on Minority Health and Health Disparities 2018).
Data Science
Oral health data science continues to evolve as an integral contributor to emerging technologies that advance treatment for oral diseases and disorders. However, few institutions have explored the potential for utilizing EHR data to study oral health problems that may cause or co-occur with other medical conditions.
Applying mathematical and computational approaches to complex and multidimensional data can lead to a deeper understanding of dental, oral, and craniofacial health and ultimately transform how oral health care is delivered. This outcome will involve a more comprehensive integration of basic, clinical, and population science to devise new tools and approaches. Understanding the spectrum of information and data science requires that oral health data scientists be skilled in applying advanced data-mining approaches for analyses of large amounts of data (Figure 11).
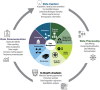
FIGURE 11
Data management life cycle.
Data Modeling
Predictive computational modeling offers a tool that can enhance research capacity, contribute to better management of health care systems, and support patient-clinician shared decision-making. Current projections suggest that the United States will spend US$6 trillion on health care in 2027, with the vast majority of those dollars spent on treatment (Keehan et al. 2020). Predictive computational modeling is critically important to making health care more efficient and less expensive because it emphasizes risk assessment and prevention of disease progression. Rigorous statistical modeling and machine learning approaches in predictive modeling offer an opportunity to make scientific advances relevant to all ages and to reduce health disparities.
Predictive computer modeling approaches offer a way to understand the relationships among genotype, phenotype, environmental factors, and the patterns of craniofacial, oral, and dental diseases and disorders (Pendergrass et al. 2011). Predictive models from larger samples are more likely to include diverse population features (Cremers et al. 2017). Prediction of individual differences using Machine learning approaches for data analytics of phenotypes from clinical, imaging, and omics data (Scheinost et al. 2019) improves the ability to uncover novel oral health associations. However, predictive modeling may explain disappointingly little of the variance in predicted variables, particularly when compared to results derived from descriptive models (Whelan and Garavan 2014; Yarkoni and Westfall 2017).
Increased predictive computer modeling and machine learning research efforts can be combined for research on dental caries, periodontal diseases, oral and pharyngeal cancers, chronic facial pain, temporomandibular joint dysfunction, Sjögren’s syndrome, and other craniofacial, oral, and dental autoimmune diseases. In addition, salivary biomarkers may allow oral health professionals to screen for risk for many systemic diseases and disorders, such as Alzheimer’s disease, cancer, and diabetes (Băbţan et al. 2019; Díez López et al. 2019; Hrubešová et al. 2019; Liu et al. 2019; Wang et al. 2019; Wunsch et al. 2019).
Future work focused on creating easy to use and understand results to support shared decision-making by clinicians and patients bears promise for improving oral health. The development of guidelines to monitor these models’ performance will be essential to improving process and patient outcomes.
Data Integration and Interoperability
The interoperability and sharing of health and health-related data are critical to improving care quality and efficiency. New information is generated during every patient encounter in a hospital, dental practice, physician’s office, pharmacy, laboratory, and public health and human services agency, or with emergency medical services. This information currently is stored in separate databases, and no single unique identifier exists to link disparate information into a single comprehensive patient record. Whether in large dental academic institutions, health maintenance organizations (HMOs), or small dental practices, the use of stand-alone systems with different patient identifiers—such as internal billing systems, electronic dental records, laboratory orders, and digital images—may result in treatment delays and prevents data aggregation for clinical decision support. This also severely limits the utility of electronic data for clinical research.
Integrating traditional data such as insurance claims, clinical research, and electronic health and dental record data with the data from these new resources has the potential to deliver precise and personalized assessment of a population’s or person’s risk for a disease and recommended treatment. To achieve this goal, it is necessary to continue developing and evaluating patient-matching algorithms to integrate data from disparate sources.
Because there is no unique patient identifier used in the United States as there is in countries with nationalized health care, U.S. health care organizations must rely on patient-matching algorithms using demographics and other identifiers. Regional health information exchanges, such as the Indiana Health Information Exchange, have been successful in transmitting messages across more than 100 hospital systems annually by using patient-matching algorithms to match patient records. However, no information exchange is occurring between electronic health and dental record systems except in HMO settings that have integrated medical and dental record systems. Future research should enhance the accuracy of patient-matching algorithms to integrate electronic health and dental record data and to facilitate exchange of information between these systems.
Establishing a common vocabulary and mapping the terminologies used in different settings to the common vocabulary can enable divergent systems to communicate. The American Dental Association (ADA) Standards Committee on Dental Informatics is a standard-setting organization accredited by the American National Standards Institute that involves researchers and public health policymakers in developing standard terminologies and a conceptual framework to promote interoperability and seamless data exchange across different health information technology systems. Expanded work to include electronic health and dental record system vendors, dental and health care organization leadership, public health and federal government officials, and oral health informatics and data science researchers will provide greater breadth and depth for effective patient care.
In addition to informatics, future work also should build on existing work in medicine, dentistry, and related fields, such as information and data science and computer science, to develop approaches that enable continuous monitoring and improvement of the quality of data gathered during provider-patient encounters. Furthermore, research is needed to develop and validate metrics for evaluating the quality of different data types from diverse data sources. It also is important to characterize patient profiles by carefully phenotyping the electronic health and dental record data using advanced data-mining approaches that leverage all data types, such as structured, unstructured, images, and data recorded in various different formats.
Data Capture
Advances in raw data capture from laboratory, fieldwork, surveys, clinical examinations, volumetric and surface imaging and sensor devices, online service companies, and simulations require emerging technologies for data management infrastructure. Both quantitative and qualitative patient-related data can arise from epidemiology, genomic analyses, clinical care processes, imaging assessments, patient-reported outcomes, and environmental exposure records, as well as a host of social indicators, such as educational records, employment history, and genealogical records. For example, the automated de-identification of images is addressed today by open-source tools such as 3D Slicer and the Medical Imaging Resource Center Clinical Trial Processor, which receive Digital Imaging and Communications in Medicine images and replace protected health information with de-identified data. Large amounts of data that do not originate from health-related activities, such as data originating from social media platforms, are currently captured for a variety of purposes. However, there could be some potential for public health use through this digital data source.
Clinical images produced by imaging devices and optical scanners are reconstructed from raw, or source, data produced by detectors. The optimal conversion of source data from these sensors into reconstructed tomographic images suitable for human interpretation or radiomics is an emerging area of value. The successes of AI techniques in analyzing the ImageNet database of more than 14 million annotated nonmedical images have led to explosive growth in the use of deep learning to analyze clinical images and other health data. These promising computer vision systems, which perform clinical image interpretation tasks at the level of expert clinicians, have the potential to transform medical and dental imaging and reduce diagnostic errors, improve patient outcomes, enhance efficiency, and reduce costs (Gulshan et al. 2016; de Dumast et al. 2018).
A picture archiving and communication system is a medical imaging technology that provides economical storage and convenient access to images from multiple types of source machines and imaging modalities. Such a system can combine all of a patient’s information, including 3D data, into one universal file to homogenize the process of image reconstruction within the different systems. It offers the potential to export data to external third parties such as dental laboratories, medical specialists, and referring practitioners, subject to regional data-protection issues. Appropriate security procedures (including establishing permissions for access to data identifiers) and novel algorithms for statistical analyses and interpretation of generated data need to be developed and implemented. In this context, legal regulations must define clear standards for the security of patient data.
Data Linkages
During the past decade, efforts at linking large data sources have been accelerating. Data linkage is simply connecting information from different sources that relate to the same person or the same construct that results in a more robust dataset. At the federal level, the National Center for Health Statistics (NCHS) has been working to provide a rich, protected set of linked data files using some NCHS health survey datasets with administrative data from other agencies to promote research (National Center for Health Statistics 2020). Using linked datasets for surveillance and assessing the effectiveness of policy interventions holds great promise. However, new data partnerships are needed not only to better capture and assess overall health and well-being, but also to facilitate the incorporation of relevant oral and craniofacial health data (Secretary’s Advisory Committee for Healthy People 2030 2019). Encouraging these data linkage activities will be a source of new information to help guide planning of oral health programs, policy, and research well into the next decade.
Artificial Intelligence and Related Information Technologies
AI takes computing beyond automation, computation, and storage and enables digital systems to analyze and make decisions resembling human thought. AI entered the lexicon in 1956 as more of an aspirational vision than a reality. Since 2000, that vision has evolved substantially and now promises the realization of exciting technologic advances for dental practice. This evolution has become possible because of enormous increases in computing power and communications coupled with miniaturization, big data aggregation and analytics, neural networks, and machine and deep learning. This ever evolving explosion in computing power, along with enormous improvements in the algorithms driving hardware and software design, are enabling huge advances in digital thinking that allow these systems to rapidly learn from experience.
AI has several potential future applications in the dental office. In the short term, AI may be used to enable patients to self-schedule and to optimize a practice’s calendar, taking into consideration such variables as the requirements for various procedures. This capacity could be coupled with ride services (e.g., Uber, Lyft) to automate the transportation of patients to and from the practice. Further out, AI software algorithms will be able to study standard dental photographs and radiographs to “see” disease—dental caries, periodontal disease, abscesses, cancers, and other hard- and soft-tissue diseases—at a very early stage, often much earlier and more reliably than a provider’s eyes alone. Another emerging application for AI is its use in the identification and interpretation of biomarkers to diagnose serious, often life-threatening, oral and systemic cancers and other diseases. Salivary diagnostics approaches are becoming more common and an expansion of their use at home is inevitable. As these new solutions achieve regulatory approval, dentistry also will be able to conduct inexpensive and easy-to-perform, noninvasive liquid biopsies on saliva samples to intercept serious local or systemic disease, often before appearance of definitive lesions and before symptoms are reportable. Finally, there are many opportunities to use AI and machine learning techniques for tracking and assessing educational outcomes.
Teledentistry
Teledentistry offers substantial potential for improving the oral health of underserved populations. The application of telehealth and teledentistry also creates opportunities for distance learning that improve dental science and oral health literacy. Similarly, mobile health care services and the use of mobile technology, such as smartphone apps and text messages to manage and track dental health conditions or promote healthy behaviors, have substantial promise for further expanding the effective utilization of teledentistry in both the private and public health sectors. Clearly, teledentistry provides a feasible choice for remote screening, diagnosis, consultation, treatment planning, and education in dentistry (Irving et al. 2018). One successful application of teledentistry to improve access to oral health specialty care for high risk rural children is the partnership among the Eastman Institute for Oral Health, the Finger Lakes Migrant HealthCare Project, the Community Health Center of the North Country, and others (Box 1).
Box 1How does a rural community ensure that high-risk children receive oral health specialty care?
Sometimes a child has such severe dental problems that treatment under anesthesia is required. Getting to a medical center that can provide this kind of treatment can be difficult for families who live far from it. The Teledentistry Initiative at the Eastman Institute for Oral Health, University of Rochester, New York, facilitates access to specialty care for these children and their families. When a community dentist in a rural setting refers a child for treatment under anesthesia, an audio and video meeting is arranged with the child’s family and a pediatric dentist at the Eastman Institute. This remote meeting serves as the initial dental examination, allows the dentist to determine whether the child needs treatment under anesthesia or other special treatment, gives the parents the chance to have their questions answered, and builds rapport between the child, family, and dentist. Since 2004, more than 1,500 children and their families have participated in this pediatric teledentistry program. In a 2014 published review of the first 251 patients needing treatment under anesthesia, the program achieved a 93% treatment completion rate. Partners with the Eastman Institute for Oral Health include the Finger Lakes Community and Migrant Health Center, North Country Federally Qualified Health Center, community dentists, the American Teledentistry Association, and the Monroe Country Department of Health.
Promising partnerships and programs such as these can serve as models for others to improve the oral health of underserved populations living in rural areas. Another example of a promising new direction in teledentistry is use of the Institute for Healthcare Improvement’s plan-do-study-act cycle to rapidly institute a teledentistry helpline at the start of the COVID-19 pandemic (Weintraub et al. 2020).
Learning Health Systems
Recognition of the roles learning health systems (LHSs) can play in eliminating health disparities, including oral health disparities, has been growing. Organizations and networks working to improve the health of underserved individuals and communities have embraced the concept and are advancing it in ways that are broadly inclusive and participant-driven.
For example, an LHS approach could be employed to address gaps in evidence and evidence-based practice by forming a multi-stakeholder learning community with shared interest in learning about and improving treatments for chronic periodontal disease. A key first step would be to capture relevant data from real-world patient care and experiences to study the problem of interest (e.g., assessing what adjuncts to supplement scaling and root planing work best for different types of patients). This could include patients’ observations of symptoms and self-reports of health-related behaviors as well. Next, analytics could be used to generate new knowledge from the data (e.g., an algorithm to predict which adjuncts are most likely to benefit different types of patients). The knowledge could then be mobilized in actionable forms to clinicians (as well as patients) and implemented into clinical practice, perhaps in the form of user-friendly applications built into clinical decision support and subsequently integrated into EHR systems. In turn, successive cycles of data capture, analyses to generate knowledge, and implementation would begin to enable continuous learning and improvement. The knowledge would be refined, optimized, and updated. Such an approach holds the potential to transform how treatment decisions are made.
Implementation Science
Implementation science holds tremendous promise for improving oral health for all, including reducing health disparities, by addressing factors at the individual, clinician, health care system, community, and societal levels that may limit the accessibility or effectiveness of evidence-based practices for disadvantaged and underserved populations (Chinman et al. 2017).
Rigorous clinical trials are necessary to test the efficacy and effectiveness of new interventions. The existence of customizable digital platforms that cover the entire spectrum of a clinical trial, including informed consent, recruiting, enrollment, adverse event monitoring, study balance and randomization, remote data portals, and validated methods for data collection (such as questionnaires) are a boon. Remote telehealth and telemedicine (e-visits) and real-time study monitoring are becoming realities. The ability to leverage more targeted recruitment tools, including social media and real-time links to patient advocacy groups, will streamline the recruitment process and save time and research costs while ensuring that the right population is used to answer the research question.
Large datasets collected during these trials and overlaid with advanced algorithms and data science capabilities allow for deeper insights and personalized prevention and treatment approaches. Opportunities to access and leverage data from multiple investigators to amplify learning and fill in knowledge gaps will create faster and ultimately better-targeted research programs. Given the need to increase the pace of innovation for oral health, clinical trials with enhanced technology for administrative activities and data collection and analysis will be even more important, especially when specialized patient populations are required.
Scientific advances in dental research are promoting dental technology development. The success of some dental product companies is encouraging more investment in dental technology. Furthermore, the U.S. Food and Drug Administration (FDA) is making a strong effort to accelerate product approval at the direction of the 21st Century Cures Act, passed in 2016, which enhances the agency’s ability to modernize clinical trial designs and clinical outcome assessments and speed the development and review of novel medical products. In addition, the Act directs the FDA to coordinate activities among the drug, biologics, and device centers. It also improves the regulation of combination products, which are products composed of any combination of a drug, biologic product, and device.
The 21st Century Cures Act also directs the FDA to include the patient’s voice in drug development and review by developing a regulatory framework to evaluate how real-world evidence (RWE)—data regarding the use, or the potential benefits or risks, of a drug derived from sources other than traditional clinical trials—can potentially be used to support approval of new indications for approved drugs or to support or satisfy post-approval study requirements. The FDA approach to incorporating patient-reported outcomes into the approval process is highly effective for measuring meaningful treatment benefits (Patrick et al. 2007).
A variety of sources relating to the delivery of health care and its outcomes can provide RWE, including EHRs, claims and billing data, and product and disease registries. Use of such evidence has the potential to allow researchers to answer questions about treatment effects and outcomes efficiently, saving time and money while yielding answers relevant to broader populations of patients than would be possible in a specialized research environment. This approach can help to streamline clinical development and inform products’ safe and effective use.
The FDA also is tasked with facilitating more efficient product development by helping sponsors incorporate complex, adaptive, and novel trial designs into proposed clinical protocols and applications for new drugs and biologic products.
Research Workforce, Education, and Training
Status of Research in Dental Educational Programs
Within the last 5–7 years, much study and discussion have addressed science, technology, engineering, and mathematics workforce needs and doctoral education (Human Microbiome Project Consortium 2012a; 2012b; National Academies of Sciences, Engineering, and Medicine 2018a). By placing increased emphasis on skills needed for working both within and beyond academic institutions, graduate education will benefit from increased focus on interdisciplinary science, collaborative team science, and technology and data science.
Comprehensive research universities, including member institutions within the Association of American Universities (AAU), prepare 70–80% of all Doctor of Philosophy graduates. Schools of dentistry are represented in about one-third of AAU member institutions. At the same time, new schools of dentistry have emerged without visible, productive research programs and lack the collaborative strengths of medical and public health sciences found in comprehensive research universities. Reports and research represented in the Bailit and Formicola (2017) project, “Advancing Dental Education in the 21st Century,” address needed changes to ensure sustainable commitments to contemporary research.
How the profession comes together to realize the future for graduate and professional education is key to sustaining these advances and making improvements in clinical care accessible. The students admitted to dental schools today, whether pursuing science or clinical care, will be working well into the second half of the 21st century. Most of this future work could very well be related to advancing science in oral-systemic connections and discovering appropriate interventions to improve oral and craniofacial health. Such work will require collaborations across medicine, engineering, and dentistry in multidisciplinary areas of precision health care, big data, systems biology, stem cell biology, biomaterials, and tissue engineering (Polverini and Krebsbach 2017).
Supporting Oral Health Science Training
Dental institutions have shared the vision of shaping dentistry’s future leaders to sustain the mission of advancing health through education, service, research, and discovery. It is time to focus on the early stages of the pipeline, with enhanced training and constant stewardship to sustain an optimal support system for young scientists and clinician-scientists.
Academia is a major pathway for oral health scientists, but recruitment alone without increasing the pool of candidates will have limited impact on solving the dental faculty workforce shortage. Evidence suggests that formal training programs that provide students with necessary skills and basic knowledge about academia enhance awareness and facilitate the development of an academic career after graduation (Roger 2008; Gironda et al. 2013; Horvath et al. 2016). Dental institutions and professional organizations have developed programs to actively promote the recruitment and retention of future faculty. ADA, the American Dental Education Association (ADEA), and the American Association for Dental, Oral, and Craniofacial Research (AADOCR) (formerly the American Association for Dental Research) offer dental careers and professional development fellowships to dental students and educators that include structured mentoring, career insights, and hands-on experiences in research, teaching, and other aspects of an academic career. ADEA’s Academic Dental Careers Fellowship Program recommends that institutions improve the climate for academic careers, promote academia’s value, and increase robust interactions among students, faculty, and the institution (Palatta 2016).
Mentoring programs in dental schools and professional organizations also will help strengthen grant-writing skills, improve young faculty retention and advancement in academic careers, and enhance workforce diversity. A national mentoring program involving many institutions will be essential to diminishing disparities among institutions and to enhancing diversity in the faculty workforce (Jones et al. 2017). Cultivating partnerships and collaborations with NIH and professional dental organizations such as ADA, ADEA, and AADOCR; enhanced utilization of the NIH Loan Repayment Program to address educational debt; and developing research training programs targeted to the unique needs and challenges of dentist-scientists would enhance their abilities to successfully compete for research funding from NIH and other federal and nonfederal sources to sustain their research careers.
Several organizational efforts are attempting to increase and diversify the oral health scientific workforce. These include the National Institute of Dental and Craniofacial Research (NIDCR) Award for Sustaining Outstanding Achievement in Research, the NIH Scientific Workforce Diversity Office, and the NIH Policy Supporting the Next Generation Researchers Initiative. NIDCR funds such programs as the Director’s Postdoctoral Fellowship to Enhance Diversity in Dental, Oral, and Craniofacial Research; the Summer Dental Student Award; the Dentist Scientist Career Transition Award for Intramural Investigators (K22); and the Dual Degree Dentist Scientist Pathway to Independence Award (K99/R00), created in 2008. These programs, along with all research training, should be monitored in partnership with the community of stakeholders to assess their effectiveness.
Chapter 4. Summary
Remarkable changes have occurred in research and technology during the last 20 years that hold promise to improve the orchestration of patient care and elevate oral and systemic health for all Americans. A concerted effort to optimize these discoveries while delving deeper into mechanisms of disease, engineering strategies, data science, and implementation science is essential to continue the momentum and translate discoveries into realities (See Box 2 for Key Summary Messages and Call to Action).
New findings about the human genome, oral microbiome, virome, and other omics are essential to transforming health care practices. Such discoveries are pivotal for improved oral and overall health. New scientific tools enable finer-detailed analyses of the underpinnings of diseases that have an impact on the oral and craniofacial region. Such approaches facilitate the ability to dig deeper in the pursuit of effective prevention and treatment strategies. Scientific advances encourage the unfurling of new discoveries, challenge present dogma, and enrich health care’s future. The emergence of novel pathogens, such as severe acute respiratory syndrome coronavirus 2 (CoV-2), which causes COVID-19, and other as-yet-unknown agents, requires ongoing efforts to strengthen the scientific base and swiftly incorporate scientific advances in technology to optimize clinicians’ abilities to provide oral health care safely and effectively.
The exciting advances regarding the microbiome’s interaction with humans provide many opportunities for a better understanding of human oral diseases and development of new therapeutic directions. The oral microbiome has huge targeting potential and offers a strategic opportunity for microbial gene editing alongside human genome editing.
The development of health data comprising microbial and clinical information, coupled with robust bioinformatics approaches, has great potential to put big data to work in new approaches to health services research and also to realize the potential of personalized oral health care. Several pieces of the oral health–disease puzzle are amenable to analysis and incorporation into valuable models, including metabolomics, proteomics, phenomics, and genomics. Ongoing efforts in biotechnology and digital and information technology are generating large datasets that can be mined to promote personalized care. The aggregation of many individuals’ data will inform clinical decision-making. These datasets will identify profiles of patients at risk for advancing dental diseases, communities at greatest risk for oral diseases, such as dental caries or oral cancer, and links with comorbid systemic diseases. Learning health systems at various levels of scale promise to transform human health by delivering knowledge to the right person, at the right time, in the right dose, through the right route, and in the right form. These innovations provide an opportunity to rethink oral health prevention and care delivery for the entire public and, most importantly, for underserved populations.
Whether science focuses on diagnostics, therapeutics, or technology, it also must address the steps needed to assist providers in adopting these new technologies and scientific approaches. The intersection of science and technologic advances presents unique opportunities for the dental profession. Science drives the need for innovative technologies, and the testing of new devices and platforms informs the gathering of evidence that can drive the best oral health practices. Dental care is constantly changing; new treatments are introduced, and old treatments are phased out. Preventive measures need to be constantly considered and new approaches adopted to address dental, oral, and craniofacial diseases and disorders. Implementation science strategies will translate the most effective preventive and interventional approaches to help providers achieve optimal standards of care.
Restorative materials that go beyond simple replacement, with bioinspired foundations to form new mineral and prevent future disease, are rapidly evolving.
Box 2Key Summary Messages for Emerging Science and Promising Technologies to Transform Oral Health
- Science and technology provide the essential foundation for preventing and treating oral disease, and research breakthroughs during the past 20 years offer exciting opportunities to improve oral health.
- Tremendous advances have occurred in the technology supporting dental practice, including new, bio-inspired dental materials, innovative chairside diagnostic strategies, 3-D bioprinting, improved digital imaging, and artificial intelligence-based models.
- Discoveries related to the microbiome, genomics, and other omics offer promise for individualizing oral health care and revealing relationships among systemic diseases, suggesting possibilities for new treatments.
- Discoveries in cellular analyses and regenerative medicine are leading to new treatments to improve oral health, including techniques and products created for bone and soft tissue regeneration that currently are being used in clinical settings.
- The field of data science has grown considerably in the past 20 years, offering improved opportunities in “big” data analytics using integrated electronic health records and other large heath databases for analyses as computing power has become more robust and efficient.
- Implementation science holds promise for more effective adoption of evidence-based oral health practices, reduction of oral health disparities, and improvement of oral health equity.
- The emergency of novel pathogens, such as COVID-19 and other as yet unknown agents, underscores the need to strengthen then scientific base and ensure the flexibility of the scientific enterprise to swiftly incorporate advances and technology necessary for addressing new health challenges.
Call to Action:
- Training, support, and mentorship of more oral health scientists and academics are needed to ensure a robust workforce who can extend and effectively use the advances in science that are so critical for delivering care in the changing landscape of oral health.
The field would benefit from robust clinical testing of oral ecosystems to better assess microbial, biochemical, and biomechanical challenges. The development of dental materials that are simpler to use, more durable, aesthetic, and effective against the attachment of plaque biofilms in the oral cavity is critical.
Advances in data science are having an impact on many facets of daily living, with promising aspects for dental care that still, however, lag in their translation to dental practice. Data capture has grown at an unprecedented rate, with collateral requirements for data management that include data warehousing, cleansing, governance, security, architecture, quality monitoring, and documentation. Data processing and in-depth analysis are needed to validate and test models before deployment and to demonstrate progress toward personalized oral health care. Health informatics, a rapidly growing area of interest in health care and public health, is driven by data science to inform timely decision-making. The conversion of dental records into a connected electronic health record framework with medical, pharmacy, and behavioral health is absolutely crucial for the integration of oral health with overall health to be fully realized.
Future work should focus on developing guidelines to monitor the performance of these models to improve process and patient outcomes. When implementing these data science approaches, it is critical to ensure that modeling and other artificial intelligence diagnostic developments using large datasets are done in an ethical way that does not discriminate against individuals based on characteristics including race, ethnicity, and gender.
There have been tremendous advances in the technology supporting dental practice, notably digital approaches, chairside diagnostic strategies, and imaging. Translational/clinical and scientific/mechanistic frontiers must converge in order for saliva-based, personalized, and precision medicine applications to be used in clinical practice. The existing clinical workforce needs to be kept abreast of new, evidence-based approaches for improving oral health care. The scientific workforce also needs to be kept aware of the scientific underpinnings of new approaches that challenge previously held tenets. New technologies should be strategized to reduce health disparities and expand the ability to provide care to a broader population. The profession needs to carefully strategize best practices for optimizing teledentistry to improve oral health care.
In the therapeutic arena, robotics will assist traditional dental surgical procedures, such as implants, in making treatment more precise and freer from side effects. Innovative new drugs, such as microbiome-modulating compounds or proresolving and anti-inflammation reagents with novel drug delivery systems, will be developed to treat and prevent major dental diseases, such as dental caries and periodontitis. Stem cell based tissue regeneration bears potential for repairing tooth, bone, and soft tissue damage, and new bioinspired dental materials will replace traditional dental materials. Most important, preventive dentistry could take center stage with the increased and improved identification of at-risk patients and those who experience disparities in care.
In the digital and information technology arena, dentistry will routinely employ digital scanning and three-dimensional bioprinting-based dental therapies. Artificial intelligence and machine learning will improve software construction of personalized, multidimensional, virtual models representing mucosa, teeth, bone, and cartilage. Multidimensional recording and the mixture of virtual reality and augmented reality will enable remote diagnostics, presurgical lifelike simulation, enhanced treatment planning, and the execution of therapy using guidance or navigation. Big data generated through nationwide datasets and integrated, pooled electronic medical records will be mined and analyzed with artificial intelligence-based data technologies to identify trends, associations, and clinical outcomes to create new types of evidence-based dentistry. Active ongoing and future studies and close partnerships among federal regulatory agencies, states, institutions, industry stakeholders, and patient advocates will optimize regenerative therapies in the next decades.
To realize optimal health in the population, the scientific workforce requires continual attention and direction. Programs should be complemented, expanded, and partnered with academic institutions to support the education of the future oral sciences workforce. Although the development of the scientific workforce has plateaued, the need for well-trained faculty members has grown. An ideal dental school graduate would be a highly skilled clinician with a deep understanding of the scientific foundation of dentistry, an appreciation of population health issues, and the compassion to serve. A necessary subset of those individuals should be cultivated to continue to focus scientific training on aspects of oral health science that are critical for the nation’s future care needs. Programs and research training of the oral health research workforce should be expanded and monitored in partnership with the stakeholder community to assess effectiveness and outcomes of ongoing and new programs, ensure diversity at all levels, embrace team science, and course-correct to achieve intended goals. Stakeholders should identify shared priorities and leverage resources to achieve common goals for developing a robust and diverse oral health research workforce that is well prepared and capable of meeting future oral health research needs.
During the past 20 years, basic scientific discoveries in such areas as the microbiome, genomics, and regenerative medicine provided potential to improve population and individual oral health. Data science and other technologies have substantially advanced, providing opportunities to help individuals access care, making dental care more patient-centered, and presenting opportunities to improve health informatics that guide decision-making. Oral health research has an important role in facilitating new knowledge that can be used not only to improve the nation’s oral health, but also to advance public health in a way that improves overall health and well-being.
References
- 7wData. Is Programming Knowledge Required to Pursue Data Science? 2019 (July 2). https://7wdata
.be/data-analysis /is-programming-knowledge-required-to-pursue-data-science/. Accessed August 12, 2021. - Abduo J. Accuracy of casts produced from conventional and digital workflows: A qualitative and quantitative analysis. Journal of Advanced Prosthodontics. 2019;11(2):138–46. [PMC free article: PMC6491364] [PubMed: 31080575]
- Abogazalah N, Ando M. Alternative methods to visual and radiographic examinations for approximal caries detection. Journal of Oral Science. 2017;59(3):315–22. [PubMed: 28529280]
- Abou Tara M, Eschbach S, Bohlsen F, Kern M. Clinical outcome of metal-ceramic crowns fabricated with laser-sintering technology. International Journal of Prosthodontics. 2011;24(1):46–8. [PubMed: 21210003]
- Abusleme L, Diaz PI, Freeman AF et al. Human defects in STAT3 promote oral mucosal fungal and bacterial dysbiosis. JCI Insight. 2018;3(17). [PMC free article: PMC6171814] [PubMed: 30185668]
- Acharya A. Marshfield Clinic Health System: Integrated care case study. Journal of the California Dental Association. 2016;44(3):177–81. [PubMed: 27044239]
- Acharya A, Cheng B, Koralkar R et al. Screening for diabetes risk using integrated dental and medical electronic health record data. JDR Clinical & Translational Research. 2018;3(2):188–94. [PMC free article: PMC5858652] [PubMed: 29568804]
- Acharya A, Schroeder D, Schwei K, Chyou PH. Update on Electronic Dental Record and clinical computing adoption among dental practices in the United States. Clinical Medicine & Research. 2017;15(3–4):59–74. [PMC free article: PMC5849439] [PubMed: 29229631]
- Acharya A, Yoder N, Nycz G. An integrated medical-dental Electronic Health Record environment: a Marshfield experience. In: Powell V, Din F, Acharya A, Torres-Urquidy M, eds. Integration of Medical and Dental Care and Patient Data. Vol 3. London: Springer; 2012:331–51.
- Agler CS, Divaris K. Sources of bias in genomics research of oral and dental traits. Community Dental Health. 2020;37(1):102–6. [PMC free article: PMC7316399] [PubMed: 32031351]
- Ajiboye SA, Mossey PA, IADR Science Information Committee, Fox CH. IADR policy and position statements on the safety of dental amalgam. Journal of Dental Research. 2020;99(7):763–8. [PubMed: 32315245]
- Al-Hebshi NN, Baraniya D, Chen T et al. Metagenome sequencing-based strain-level and functional characterization of supragingival microbiome associated with dental caries in children. Journal of Oral Microbiology. 2019;11(1):1557986. [PMC free article: PMC6327923] [PubMed: 30671194]
- Albert DA, Begg MD, Andrews HF et al. An examination of periodontal treatment, dental care, and pregnancy outcomes in an insured population in the United States. American Journal of Public Health. 2011;101(1):151–56. [PMC free article: PMC3000729] [PubMed: 21088265]
- Alberts B, Kirschner MW, Tilghman S, Varmus H. Rescuing U.S. biomedical research from its systemic flaws. Proceedings of the National Academy of Sciences. 2014;111(16):5773–7. [PMC free article: PMC4000813] [PubMed: 24733905]
- Albrektsson T, Wennerberg A. On osseointegration in relation to implant surfaces. Clinical Implant Dentistry and Related Research. 2019;21(Suppl 1):4–7. [PubMed: 30816639]
- Alghazzawi TF. Advancements in CAD/CAM technology: Options for practical implementation. Journal of Prosthodontic Research. 2016;60(2):72–84. [PubMed: 26935333]
- American Dental Association, Health Policy Institute. Dental Education. Survey of Advanced Dental Education. 2020. https://www
.ada.org/en /science-research/health-policy-institute /data-center/dental-education. Accessed August 12, 2021. - Anderson LM, Adeney KL, Shinn C, Safranek S, Buckner-Brown J, Krause LK. Community coalition-driven interventions to reduce health disparities among racial and ethnic minority populations. Cochrane Database of Systematic Reviews. 2015(6):CD009905. [PMC free article: PMC10656573] [PubMed: 26075988]
- Anderson M, Anderson SL. How should AI be developed, validated, and implemented in patient care? AMA Journal of Ethics. 2019;21(2):E125–30. [PubMed: 30794121]
- Andra SS, Austin C, Wright RO, Arora M. Reconstructing pre-natal and early childhood exposure to multi-class organic chemicals using teeth: towards a retrospective temporal exposome. Environment International. 2015;83:137–45. [PMC free article: PMC4545311] [PubMed: 26134987]
- Angmar-Mansson B, de Josselin de Jong E, Sundstrom F, ten Bosch JJ. Strategies for improving the assessment of dental fluorosis: focus on optical techniques. Advances in Dental Research. 1994;8(1):75–9. [PubMed: 7993563]
- Asa’ad F. Shared decision-making (SDM) in dentistry: a concise narrative review. Journal of Evaluation in Clinical Practice. 2019;25(6):1088–93. [PubMed: 30920092]
- Athirasala A, Lins F, Tahayeri A et al. A novel strategy to engineer pre-vascularized full-length dental pulp-like tissue constructs. Scientific Reports. 2017;7(1):3323. [PMC free article: PMC5468292] [PubMed: 28607361]
- Atzeni E, Salmi A. Evaluation of additive manufacturing (AM) techniques for the production of metal-ceramic dental restorations. Journal of Manufacturing Processes. 2015;20:40–5.
- Băbţan AM, Ilea A, Boşca BA et al. Advanced glycation end products as biomarkers in systemic diseases: premises and perspectives of salivary advanced glycation end products. Biomarkers in Medicine. 2019;13(6):479–95. [PubMed: 30968701]
- Bailit H, Formicola A. Introduction to advancing dental education in the 21st century project. Journal of Dental Education. 2017;81(8):1004–7. [PubMed: 31990407]
- Baillie R, Tan ST, Itinteang T. Cancer stem cells in oral cavity squamous cell carcinoma: a review. Frontiers in Oncology. 2017;7:112. [PMC free article: PMC5454033] [PubMed: 28626726]
- Baiocchi M, Cheng J, Small DS. Instrumental variable methods for causal inference. Statistics in Medicine. 2014;33(13):2297–2340. [PMC free article: PMC4201653] [PubMed: 24599889]
- Baker JL, Bor B, Agnello M, Shi W, He X. Ecology of the oral microbiome: beyond bacteria. Trends in Microbiology. 2017;25(5):362–74. [PMC free article: PMC5687246] [PubMed: 28089325]
- Banbury A, Roots A, Nancarrow S. Rapid review of applications of e-health and remote monitoring for rural residents. Australian Journal of Rural Health. 2014;22(5):211–22. [PubMed: 25303412]
- Banerjee S, Tian T, Wei Z et al. Microbial signatures associated with oropharyngeal and oral squamous cell carcinomas. Scientific Reports. 2017;7(1):4036. [PMC free article: PMC5481414] [PubMed: 28642609]
- Bansal R, Bolin KA, Abdellatif HM, Shulman JD. Knowledge, attitude and use of fluorides among dentists in Texas. Journal of Contemporary Dental Practice. 2012;13(3):371–5. [PubMed: 22918012]
- Barnett ML. The oral-systemic disease connection: an update for the practicing dentist. Journal of the American Dental Association. 2006;137(Suppl):5–6S. [PubMed: 17012729]
- Barrera M, Jr., Castro FG, Strycker LA, Toobert DJ. Cultural adaptations of behavioral health interventions: a progress report. Journal of Consulting and Clinical Psychology. 2013;81(2):196–205. [PMC free article: PMC3965302] [PubMed: 22289132]
- Barros SP, Offenbacher S. Modifiable risk factors in periodontal disease: epigenetic regulation of gene expression in the inflammatory response. Periodontology 2000. 2014;64(1):95–110. [PubMed: 24320958]
- Bayne SC, Ferracane JL, Marshall GW, Marshall SJ, van Noort R. The evolution of dental materials over the past century: silver and gold to tooth color and beyond. Journal of Dental Research. 2019;98(3):257–65. [PubMed: 30784370]
- Baysal BE, Sharma S, Hashemikhabir S, Janga SC. RNA editing in pathogenesis of cancer. Cancer Research. 2017;77(14):3733–9. [PubMed: 28667076]
- Beall CJ, Campbell AG, Griffen AL, Podar M, Leys EJ. Genomics of the uncultivated, periodontitis-associated bacterium Tannerella sp. BU045 (Oral Taxon 808). mSystems. 2018b;3(3). [PMC free article: PMC5989130] [PubMed: 29896567]
- Beall CJ, Mokrzan EM, Griffen AL, Leys EJ. Cultivation of Peptidiphaga gingivicola from subgingival plaque: the first representative of a novel genus of Actinomycetaceae. Molecular Oral Microbiology. 2018a;33(1):105–10. [PMC free article: PMC5771945] [PubMed: 29105370]
- Bedran-Russo A, Leme-Kraus AA, Vidal CMP, Teixeira EC. An overview of dental adhesive systems and the dynamic tooth-adhesive interface. Dental Clinics of North America. 2017;61(4):713–31. [PubMed: 28886765]
- Belli R, Wendler M, de Ligny D et al. Chairside CAD/CAM materials. Part 1: Measurement of elastic constants and microstructural characterization. Dental Materials. 2017;33(1):84–98. [PubMed: 27890354]
- Bendsoe M, Sigmund O. Topology Optimization: Theory, Methods and Applications. 2nd ed. New York: Springer-Verlag; 2004.
- Berdasco M, Esteller M. Clinical epigenetics: seizing opportunities for translation. Nature Reviews Genetics. 2019;20(2):109–27. [PubMed: 30479381]
- Berdy B, Spoering AL, Ling LL, Epstein SS. In situ cultivation of previously uncultivable microorganisms using the ichip. Nature Protocols. 2017;12(10):2232–42. [PubMed: 29532802]
- Berglundh T, Jepsen S, Stadlinger B, Terheyden H. Peri-implantitis and its prevention. Clinical Oral Implants Research. 2019;30(2):150–5. [PubMed: 30636066]
- Bhargav A, Sanjairaj V, Rosa V, Feng LW, Fuh Yh J. Applications of additive manufacturing in dentistry: a review. Journal of Biomedical Material Research Part B: Applied Biomaterials. 2018;106(5):2058–64. [PubMed: 28736923]
- Bianco P, Cao X, Frenette PS et al. The meaning, the sense and the significance: translating the science of mesenchymal stem cells into medicine. Nature Medicine. 2013;19(1):35–42. [PMC free article: PMC3998103] [PubMed: 23296015]
- Bijle MNA, Ekambaram M, Lo ECM, Yiu CKY. The combined antimicrobial effect of arginine and fluoride toothpaste. Scientific Reports. 2019;9(1):8405. [PMC free article: PMC6557824] [PubMed: 31182718]
- Bindl A, Mormann WH. Marginal and internal fit of all-ceramic CAD/CAM crown-copings on chamfer preparations. Journal of Oral Rehabilitation. 2005;32(6):441–7. [PubMed: 15899023]
- Bindman A. Bringing Moneyball to Medicine. AHRQ Views. 2017 (January 18). https://archive
.ahrq .gov/news/blog/ahrqviews /bringing-moneyball-to-medicine.html. Accessed May 14, 2021. - Boes S, Mantwill S, Kaufmann C et al. Swiss Learning Health System: a national initiative to establish learning cycles for continuous health system improvement. Learning Health Systems. 2018;2(3):e10059. [PMC free article: PMC6508820] [PubMed: 31245587]
- Boland ED, Wnek GE, Simpson DG, Pawlowski KJ, Bowlin GL. Tailoring tissue engineering scaffolds using electrostatic processing techniques: a study of poly(glycolic acid) electrospining. Journal of Macromolecular Science-Pure and Applied Chemistry. 2001;38(12):1231–43.
- Bosshardt DD, Chappuis V, Buser D. Osseointegration of titanium, titanium alloy and zirconia dental implants: current knowledge and open questions. Periodontology 2000. 2017;73(1):22–40. [PubMed: 28000277]
- Bouma BE. Handbook of Optical Coherence Tomography. New York: Marcel Dekker; 2002.
- Boutin S, Hagenfeld D, Zimmermann H et al. Clustering of subgingival microbiota reveals microbial disease ecotypes associated with clinical stages of periodontitis in a cross-sectional study. Frontiers in Microbiology. 2017;8:340. [PMC free article: PMC5331054] [PubMed: 28298910]
- Bowen WH, Burne RA, Wu H, Koo H. Oral biofilms: pathogens, matrix, and polymicrobial interactions in microenvironments. Trends in Microbiology. 2018;26(3):229–42. [PMC free article: PMC5834367] [PubMed: 29097091]
- Braveman P, Gottlieb L. The social determinants of health: it’s time to consider the causes of the causes. Public Health Reports. 2014;129:19–31. [PMC free article: PMC3863696] [PubMed: 24385661]
- Brownstein CA, Beggs AH, Homer N et al. An international effort towards developing standards for best practices in analysis, interpretation and reporting of clinical genome sequencing results in the CLARITY Challenge. Genome Biology. 2014;15(3):R53. [PMC free article: PMC4073084] [PubMed: 24667040]
- Buniello A, MacArthur JAL, Cerezo M et al. The NHGRI-EBI GWAS Catalog of published genome-wide association studies, targeted arrays and summary statistics 2019. Nucleic Acids Research. 2019;47(D1):D1005–12. [PMC free article: PMC6323933] [PubMed: 30445434]
- Cabitza F, Rasoini R, Gensini GF. Unintended consequences of machine learning in medicine. Journal of the American Medical Association. 2017;318(6):517–18. [PubMed: 28727867]
- Califf RM, Robb MA, Bindman AB et al. Transforming evidence generation to support health and health care decisions. New England Journal of Medicine. 2016;375(24):2395–2400. [PubMed: 27974039]
- Campbell AG, Campbell JH, Schwientek P et al. Multiple single-cell genomes provide insight into functions of uncultured Deltaproteobacteria in the human oral cavity. PLoS One. 2013;8(3):e59361. [PMC free article: PMC3608642] [PubMed: 23555659]
- Center for Connected Health Policy. State Telehealth Laws & Reimbursement Policies. Sacramento, CA: Center for Connected Health Policy. 2020. https://www
.cchpca.org /telehealth-policy /state-telehealth-laws-and-reimbursement-policies-report. Accessed June 25, 2021. - Cheifet B. Where is genomics going next? Genome Biology. 2019;20(1):17. [PMC free article: PMC6341733] [PubMed: 30670080]
- Chen K, Guo S, Sun L, Wang JL. Global partial likelihood for nonparametric proportional hazards models. Journal of the American Statistical Association. 2010;105(490):750–60. [PMC free article: PMC3404854] [PubMed: 22844168]
- Cheng L, Zhang K, Weir MD, Melo MA, Zhou X, Xu HH. Nanotechnology strategies for antibacterial and remineralizing composites and adhesives to tackle dental caries. Nanomedicine. 2015;10(4):627–41. [PMC free article: PMC4347904] [PubMed: 25723095]
- Cheng L, Zhang K, Zhang N et al. Developing a new generation of antimicrobial and bioactive dental resins. Journal of Dental Research. 2017;96(8):855–63. [PMC free article: PMC5502962] [PubMed: 28530844]
- Chi LY, Lin PY, Wang J, Chu YR, Chang YM. Can government-supported preventive fluoride varnish application service reduce pulp-involved primary molars? Journal of Public Health Dentistry. 2019;79(3):238–45. [PubMed: 31016749]
- Chinman M, Woodward EN, Curran GM, Hausmann LRM. Harnessing implementation science to increase the impact of health equity research. Medical Care. 2017;55(Suppl 9 2):S16–23. [PMC free article: PMC5639697] [PubMed: 28806362]
- Chinn CH, Levine J, Matos S, Findley S, Edelstein BL. An interprofessional collaborative approach in the development of a caries risk assessment mobile tablet application: My Smile Buddy. Journal of Health Care for the Poor and Underserved. 2013;24(3):1010–20. [PMC free article: PMC4523798] [PubMed: 23974376]
- Chiu CY, Miller SA. Clinical metagenomics. Nature Reviews Genetics. 2019;20(6):341–355. [PMC free article: PMC6858796] [PubMed: 30918369]
- Chmar JE, Weaver RG, Valachovic RW. Dental school vacant budgeted faculty positions: academic year 2004–05. Journal of Dental Education. 2006;70(2):188–98. [PubMed: 16478933]
- Choi JE, Lyons KM, Kieser JA, Waddell NJ. Diurnal variation of intraoral pH and temperature. BDJ Open. 2017;3:17015. [PMC free article: PMC5842828] [PubMed: 29607085]
- Chu TM, Halloran JW, Hollister SJ, Feinberg SE. Hydroxyapatite implants with designed internal architecture. Journal of Materials Science: Materials in Medicine. 2001;12(6):471–8. [PubMed: 15348260]
- Chung S, Fried D, Staninec M, Darling CL. Multispectral near-IR reflectance and transillumination imaging of teeth. Biomedical Optics Express. 2011;2(10):2804–14. [PMC free article: PMC3191447] [PubMed: 22025986]
- Clark DB, Ducharme L. Charting a course for implementation research in oral health. JDR Clinical & Translational Research. 2016;1(3):198–200. [PMC free article: PMC6728445] [PubMed: 30931737]
- Clarkson JE, Turner S, Grimshaw JM et al. Changing clinicians’ behavior: a randomized controlled trial of fees and education. Journal of Dental Research. 2008;87(7):640–44. [PubMed: 18573983]
- Cocco AR, Rosa WL, Silva AF, Lund RG, Piva E. A systematic review about antibacterial monomers used in dental adhesive systems: Current status and further prospects. Dental Materials. 2015;31(11):1345–62. [PubMed: 26345999]
- Cochran DL, Oh TJ, Mills MP et al. A randomized clinical trial evaluating rh-FGF-2/beta-TCP in periodontal defects. Journal of Dental Research. 2016;95(5):523–30. [PubMed: 26908630]
- Collins FS, Varmus H. A new initiative on precision medicine. New England Journal of Medicine. 2015;372(9):793–5. [PMC free article: PMC5101938] [PubMed: 25635347]
- Collins LM, Kugler KC, Gwadz MV. Optimization of multicomponent behavioral and biobehavioral interventions for the prevention and treatment of HIV/AIDS. AIDS and Behavior. 2016;20(Suppl 1):S197–214. [PMC free article: PMC4715714] [PubMed: 26238037]
- Contreras OA, Harrison S, Stewart D, Stewart J, Valachovic RW. Our Future Faculty—The Importance of Recruiting Students and Residents to Academic Dentistry. ADEA Policy Brief. 2018. https://www
.ADEA_PolicyBrief _AcademicCareers_web.pdf. Accessed December 15, 2020. - Cremers HR, Wager TD, Yarkoni T. The relation between statistical power and inference in fMRI. PLoS One. 2017;12(11):e0184923. [PMC free article: PMC5695788] [PubMed: 29155843]
- Cuív PO, Smith WJ, Pottenger S, Burman S, Shanahan ER, Morrison M. Isolation of genetically tractable most-wanted bacteria by metaparental mating. Scientific Reports. 2015;5:13282. [PMC free article: PMC4642544] [PubMed: 26293474]
- Cunningham-Glasspoole D et al. Periodontal bacteria induce epigenetic modifications in HPV expressing keratinocytes. International Center for Genetic Engineering and Biotechnology-DNS Tumorvirus Conference; 2015; Trieste, Italy.
- Curran GM, Bauer M, Mittman B, Pyne JM, Stetler C. Effectiveness-implementation hybrid designs: combining elements of clinical effectiveness and implementation research to enhance public health impact. Medical Care. 2012;50(3):217–26. [PMC free article: PMC3731143] [PubMed: 22310560]
- D’Silva NJ, Gutkind JS. Oral cancer: integration of studies for diagnostic and therapeutic precision. Advances in Dental Research. 2019;30(2):45–9. [PMC free article: PMC6806126] [PubMed: 31633388]
- D’Souza RN, Colombo JS. How research training will shape the future of dental, oral, and craniofacial research. Journal of Dental Education. 2017;81(9):eS73–82. [PubMed: 28864807]
- D’Souza RN, Colombo JS, Embree MC, Myers JM, DeRouen TA. Our essential and endangered dentist-scientist workforce. JDR Clinical & Translational Research. 2017;2(1):10–22. [PubMed: 30938647]
- Damschroder LJ, Aron DC, Keith RE, Kirsh SR, Alexander JA, Lowery JC. Fostering implementation of health services research findings into practice: a consolidated framework for advancing implementation science. Implementation Science. 2009;4:50. [PMC free article: PMC2736161] [PubMed: 19664226]
- Daniel H, Sulmasy LS, Health and Public Policy Committee of the American College of Physicians. Policy recommendations to guide the use of telemedicine in primary care settings: an American College of Physicians position paper. Annals of Internal Medicine. 2015;163(10):787–89. [PubMed: 26344925]
- Daniels RJ. A generation at risk: young investigators and the future of the biomedical workforce. Proceedings of the National Academy of Sciences. 2015;112(2):313–18. [PMC free article: PMC4299207] [PubMed: 25561560]
- Darveau RP. Periodontitis: a polymicrobial disruption of host homeostasis. Nature Review Microbiology. 2010;8(7):481–90. [PubMed: 20514045]
- Darveau RP, Hajishengallis G, Curtis MA. Porphyromonas gingivalis as a potential community activist for disease. Journal of Dental Research. 2012;91(9):816–20. [PMC free article: PMC3420389] [PubMed: 22772362]
- de Assunção IV, da Costa Gde F, Borges BC. Systematic review of noninvasive treatments to arrest dentin non-cavitated caries lesions. World Journal of Clinical Cases. 2014;2(5):137–41. [PMC free article: PMC4023307] [PubMed: 24868513]
- de Dumast P, Mirabel C, Cevidanes L et al. A web-based system for neural network-based classification in temporomandibular joint osteoarthritis. Computerized Medical Imaging and Graphics. 2018;67:45–54. [PMC free article: PMC5987251] [PubMed: 29753964]
- Dehurtevent M, Robberecht L, Hornez JC, Thuault A, Deveaux E, Behin P. Stereolithography: a new method for processing dental ceramics by additive computer-aided manufacturing. Dental Materials. 2017;33(5):477–85. [PubMed: 28318544]
- Demarco FF, Corrêa MB, Cenci MS, Moraes RR, Opdam NJ. Longevity of posterior composite restorations: not only a matter of materials. Dental Materials. 2012;28(1):87–101. [PubMed: 22192253]
- Demmitt BA, Corley RP, Huibregtse BM et al. Genetic influences on the human oral microbiome. BMC Genomics. 2017;18(1):659. [PMC free article: PMC5571580] [PubMed: 28836939]
- Denny JC, Collins FS. Precision medicine in 2030—seven ways to transform healthcare. Cell. 2021;184(6):1415–19. [PMC free article: PMC9616629] [PubMed: 33740447]
- Denry I, Kelly JR. Emerging ceramic-based materials for dentistry. Journal of Dental Research. 2014;93(12):1235–42. [PMC free article: PMC4237640] [PubMed: 25274751]
- Denry I, Kuhn LT. Design and characterization of calcium phosphate ceramic scaffolds for bone tissue engineering. Dental Materials. 2016;32(1):43–53. [PMC free article: PMC4696887] [PubMed: 26423007]
- DeSalvo KB, Galvez E. Connecting Health and Care for the Nation: A Shared Nationwide Interoperability Roadmap (Version 1.0). HealthITBuzz. 2015. https://www
.healthit .gov/buzz-blog/electronic-health-and-medical-records /interoperability-electronic-health-and-medical-records /connecting-health-care-nation-shared-nationwide-interoperability-roadmap-version-10. Accessed June 25, 2021. - Dewhirst FE, Izard J, Paster BJ et al. The human oral microbiome database. Journal of Bacteriology. 2010;192(19):5002–17. [PMC free article: PMC2944498] [PubMed: 20656903]
- Diaz PI, Hong BY, Frias-Lopez J et al. Transplantation-associated long-term immunosuppression promotes oral colonization by potentially opportunistic pathogens without impacting other members of the salivary bacteriome. Clinical and Vaccine Immunology. 2013;20(6):920–30. [PMC free article: PMC3675961] [PubMed: 23616410]
- Didilescu AC, Cristache CM, Andrei M, Voicu G, Perlea P. The effect of dental pulp-capping materials on hard-tissue barrier formation: A systematic review and meta-analysis. Journal of the American Dental Association. 2018;149(10):903–17. [PubMed: 30098704]
- Díez López C, Vidaki A, Ralf A et al. Novel taxonomy-independent deep learning microbiome approach allows for accurate classification of different forensically relevant human epithelial materials. Forensic Science International: Genetics. 2019;41:72–82. [PubMed: 31003081]
- Dinarello CA. IL-1: discoveries, controversies and future directions. European Journal of Immunology. 2010;40(3):599–606. [PubMed: 20201008]
- Divaris K, Monda KL, North KE et al. Exploring the genetic basis of chronic periodontitis: a genome-wide association study. Human Molecular Genetics. 2013;22(11):2312–24. [PMC free article: PMC3652417] [PubMed: 23459936]
- Dolley S. Big Data’s role in precision public health. Frontiers in Public Health. 2018;6:68. [PMC free article: PMC5859342] [PubMed: 29594091]
- Dominy SS, Lynch C, Ermini F et al. Porphyromonas gingivalis in Alzheimer’s disease brains: Evidence for disease causation and treatment with small-molecule inhibitors. Science Advances. 2019;5(1):eaau3333. [PMC free article: PMC6357742] [PubMed: 30746447]
- Donia MS, Cimermancic P, Schulze CJ et al. A systematic analysis of biosynthetic gene clusters in the human microbiome reveals a common family of antibiotics. Cell. 2014;158(6):1402–14. [PMC free article: PMC4164201] [PubMed: 25215495]
- Donovan T, Simonsen RJ, Guertin G, Tucker RV. Retrospective clinical evaluation of 1,314 cast gold restorations in service from 1 to 52 years. Journal of Esthetic and Restorative Dentistry. 2004;16(3):194–204. [PubMed: 15597641]
- Doshi J, Reneker DH. Electrospinning process and applications of electrospun fibers. Conference Record of the 1993 IEEE Industry Applications Conference 28th IAS Annual Meeting; 1993; Toronto, Ontario.
- Duran-Pinedo AE, Chen T, Teles R et al. Community-wide transcriptome of the oral microbiome in subjects with and without periodontitis. The ISME Journal. 2014;8(8):1659–72. [PMC free article: PMC4817619] [PubMed: 24599074]
- Dutzan N, Kajikawa T, Abusleme L et al. A dysbiotic microbiome triggers TH17 cells to mediate oral mucosal immunopathology in mice and humans. Science Translational Medicine. 2018;10(463). [PMC free article: PMC6330016] [PubMed: 30333238]
- Eccles MP, Mittman BS. Welcome to Implementation Science. Implementation Science. 2006;1(1):1.
- Edlund A, Garg N, Mohimani H et al. Metabolic fingerprints from the human oral microbiome reveal a vast knowledge gap of secreted small peptidic molecules. mSystems. 2017;2(4). [PMC free article: PMC5516222] [PubMed: 28761934]
- Eke PI, Page RC, Wei L, Thornton-Evans G, Genco RJ. Update of the case definitions for population-based surveillance of periodontitis. Journal of Periodontology. 2012;83(12):1449–54. [PMC free article: PMC6005373] [PubMed: 22420873]
- El Kholy K, Freire M, Chen T, Van Dyke TE. Resolvin E1 promotes bone preservation under inflammatory conditions. Frontiers in Immunology. 2018;9:1300. [PMC free article: PMC6005849] [PubMed: 29946319]
- Engebretson S, Kocher T. Evidence that periodontal treatment improves diabetes outcomes: a systematic review and meta-analysis. Journal of Clinical Periodontology. 2013;40:S153–63. [PubMed: 23627325]
- Escapa IF, Chen T, Huang Y, Gajare P, Dewhirst FE, Lemon KP. New insights into human nostril microbiome from the Expanded Human Oral Microbiome Database (eHOMD): a resource for the microbiome of the human aerodigestive tract. mSystems. 2018;3(6). [PMC free article: PMC6280432] [PubMed: 30534599]
- Esposito M, Grusovin MG, Papanikolaou N, Coulthard P, Worthington HV. Enamel matrix derivative (Emdogain) for periodontal tissue regeneration in intrabony defects. A Cochrane systematic review. European Journal of Oral Implantology. 2009;2(4):247–66. [PubMed: 20467602]
- Ethier JF, McGilchrist M, Barton A et al. The TRANSFoRm project: experience and lessons learned regarding functional and interoperability requirements to support primary care. Learning Health Systems. 2017;2(2):e10037. [PMC free article: PMC6508823] [PubMed: 31245579]
- Faiola A, Holden RJ. Consumer health informatics: empowering healthy-living-seekers through mHealth. Progress in Cardiovascular Diseases. 2017;59(5):479–86. [PubMed: 28038910]
- Fan X, Alekseyenko AV, Wu J et al. Human oral microbiome and prospective risk for pancreatic cancer: a population-based nested case-control study. Gut. 2018a;67(1):120–7. [PMC free article: PMC5607064] [PubMed: 27742762]
- Fan X, Peters BA, Jacobs EJ et al. Drinking alcohol is associated with variation in the human oral microbiome in a large study of American adults. Microbiome. 2018b;6(1):59. [PMC free article: PMC5914044] [PubMed: 29685174]
- Faraji F, Zaidi M, Fakhry C, Gaykalova DA. Molecular mechanisms of human papillomavirus-related carcinogenesis in head and neck cancer. Microbes and Infection. 2017;19(9–10):464–75. [PMC free article: PMC5603399] [PubMed: 28619685]
- Fatemifar G, Hoggart CJ, Paternoster L et al. Genome-wide association study of primary tooth eruption identifies pleiotropic loci associated with height and craniofacial distances. Human Molecular Genetics. 2013;22(18):3807–17. [PMC free article: PMC3749866] [PubMed: 23704328]
- Federico F. The Five Rights of Medication Administration. 2015. http://www
.ihi.org/resources /Pages/ImprovementStories /FiveRightsofMedicationAdministration.aspx. Accessed June 25, 2021. - Feinberg M, Garcia LT, Polverini PJ, Fox CH, Valachovic RW. The vital role of research funding in preserving the oral health of the public and the dental profession. Journal of the American Dental Association. 2015;146(6):355–6. [PubMed: 26025816]
- Ferland JN, Winstanley CA. Risk-preferring rats make worse decisions and show increased incubation of craving after cocaine self-administration. Addiction Biology. 2017;22(4):991–1001. [PubMed: 27002211]
- Ferracane JL. Is the wear of dental composites still a clinical concern? Is there still a need for in vitro wear simulating devices? Dental Materials. 2006;22(8):689–92. [PubMed: 16563492]
- Ferracane JL. Resin composite—state of the art. Dental Materials. 2011;27(1):29–38. [PubMed: 21093034]
- Fischbach MA. Microbiome: focus on causation and mechanism. Cell. 2018;174(4):785–90. [PMC free article: PMC6094951] [PubMed: 30096310]
- Fisher-Owens SA, Gansky SA, Platt LJ et al. Influences on children’s oral health: a conceptual model. Pediatrics. 2007;120(3):e510–20. [PubMed: 17766495]
- Fitzgerald RJ. Dental caries research in gnotobiotic animals. Caries Research. 1968;2(2):139–46. [PubMed: 5248531]
- Formicola AJ, Bailit HL, Weintraub JA, Fried JL, Polverini PJ. Advancing dental education in the 21st Century: Phase 2 report on strategic analysis and recommendations. Journal of Dental Education. 2018;82(10):eS1–32. [PubMed: 30275149]
- Forrester K, Sheridan R, Phoenix RD. Assessing the accuracy of casting and additive manufacturing techniques for fabrication of a complete palatal coverage metal framework. Journal of Prosthodontics. 2019;28(7):811–17. [PubMed: 31115125]
- Fouad AF, Khan AA, Silva RM, Kang MK. Genetic and epigenetic characterization of pulpal and periapical inflammation. Frontiers in Physiology. 2020;11:21. [PMC free article: PMC7010935] [PubMed: 32116745]
- Foxman B, Luo T, Srinivasan U et al. The effects of family, dentition, and dental caries on the salivary microbiome. Annals of Epidemiology. 2016;26(5):348–54. [PMC free article: PMC5015694] [PubMed: 27157862]
- Fried D, Xie J, Shafi S, Featherstone JD, Breunig TM, Le C. Imaging caries lesions and lesion progression with polarization sensitive optical coherence tomography. Journal of Biomedical Optics. 2002;7(4):618–27. [PubMed: 12421130]
- Fried WA, Fried D, Chan KH, Darling CL. High contrast reflectance imaging of simulated lesions on tooth occlusal surfaces at near-IR wavelengths. Lasers in Surgery and Medicine. 2013;45(8):533–41. [PMC free article: PMC4472445] [PubMed: 23857066]
- Friedman C, Rubin J. Realizing a learning health system: a vision for education to transform the future of health. 2015. https://www
.slideshare .net/learninghealthsciences /realizing-a-learning-health-system-a-vision-for-education-to-transform-the-future-of-health. Accessed July 14, 2021. - Friedman C, Rubin J, Brown J et al. Toward a science of learning systems: a research agenda for the high-functioning Learning Health System. Journal of the American Medical Informatics Association. 2015;22(1):43–50. [PMC free article: PMC4433378] [PubMed: 25342177]
- Friedman CP, Rubin JC, Sullivan KJ. Toward an information infrastructure for global health improvement. Yearbook of Medical Informatics. 2017;26(1):16–23. [PMC free article: PMC6239237] [PubMed: 28480469]
- Fugolin APP, Pfeifer CS. New resins for dental composites. Journal of Dental Research. 2017;96(10):1085–91. [PMC free article: PMC5582688] [PubMed: 28732183]
- Fukushima KA, Marques MM, Tedesco TK et al. Screening of hydrogel-based scaffolds for dental pulp regeneration – a systematic review. Archives of Oral Biology. 2019;98:182–94. [PubMed: 30500668]
- Furquim CP, Soares GM, Ribeiro LL et al. The salivary microbiome and oral cancer risk: a pilot study in Fanconi Anemia. Journal of Dental Research. 2017;96(3):292–9. [PMC free article: PMC5298391] [PubMed: 27827319]
- Galante R, Figueiredo-Pina CG, Serro AP. Additive manufacturing of ceramics for dental applications: a review. Dental Materials. 2019;35(6):825–46. [PubMed: 30948230]
- Ganesan SM, Joshi V, Fellows M et al. A tale of two risks: smoking, diabetes and the subgingival microbiome. The ISME Journal. 2017;11(9):2075–89. [PMC free article: PMC5563960] [PubMed: 28534880]
- Gao L, Xu T, Huang G, Jiang S, Gu Y, Chen F. Oral microbiomes: more and more importance in oral cavity and whole body. Protein Cell. 2018;9(5):488–500. [PMC free article: PMC5960472] [PubMed: 29736705]
- Garcia SS, Blackledge MS, Michalek S et al. Targeting of Streptococcus mutans biofilms by a novel small molecule prevents dental caries and preserves the oral microbiome. Journal of Dental Research. 2017;96(7):807–14. [PMC free article: PMC5480807] [PubMed: 28571487]
- Garrison GE, McAllister DE, Anderson EL, Valachovic RW. Dental school vacant budgeted faculty positions, academic years 2008–09 to 2010–11. Journal of Dental Education. 2014;78(4):638–47. [PubMed: 24843898]
- Gawel R. Tuition increases at dental schools. Dentistry Today. 2018. https://www
.dentistrytoday .com/news/todays-dental-news /item/3899-tuition-increases-at-dental-schools. Accessed June 25, 2021. - Geller F, Feenstra B, Zhang H et al. Genome-wide association study identifies four loci associated with eruption of permanent teeth. PLoS Genetics. 2011;7(9):e1002275. [PMC free article: PMC3169538] [PubMed: 21931568]
- Ghali S, MacQuillan A, Grobbelaar AO. Reanimation of the middle and lower face in facial paralysis: review of the literature and personal approach. Journal of Plastic, Reconstructive & Aesthetic Surgery. 2011;64(4):423–31. [PubMed: 20471341]
- Ghodsi S, Jafarian Z. A review on translucent zirconia. European Journal of Prosthodontics and Restorative Dentistry. 2018;26(2):62–74. [PubMed: 29797847]
- Giannobile WV. Our duty to promote global oral health. Journal of Dental Research. 2013;92(7):573–4. [PubMed: 23720571]
- Giannobile WV, Somerman MJ. Growth and amelogenin-like factors in periodontal wound healing. A systematic review. Annals of Periodontology. 2003;8(1):193–204. [PubMed: 14971254]
- Gilbert GH, Richman JS, Gordan VV et al. and the DPBRN Collaborative Group. Lessons learned during the conduct of clinical studies in the dental PBRN. Journal of Dental Education. 2011;75(4):453–65. [PMC free article: PMC3297081] [PubMed: 21460266]
- Gilbert GH, Williams OD, Korelitz JJ et al. Purpose, structure, and function of the United States National Dental Practice-Based Research Network. Journal of Dentistry. 2013;41(11):1051–9. [PMC free article: PMC3812393] [PubMed: 23597500]
- Gilman M, Stensland J. Telehealth and Medicare: payment policy, current use, and prospects for growth. Medicare & Medicaid Research Review. 2013;3(4). [PMC free article: PMC4011650] [PubMed: 24834368]
- Ginther DK, Schaffer WT, Schnell J et al. Race, ethnicity, and NIH research awards. Science. 2011;333(6045):1015–19. [PMC free article: PMC3412416] [PubMed: 21852498]
- Gironda MW, Bibb CA, Lefever K, Law C, Messadi D. A program to recruit and mentor future academic dentists: successes and challenges. Journal of Dental Education. 2013;77(3):292–9. [PubMed: 23486893]
- Glurich I, Acharya A, Shukla SK, Nycz GR, Brilliant MH. The oral-systemic personalized medicine model at Marshfield Clinic. Oral Diseases. 2013;19(1):1–17. [PMC free article: PMC3389173] [PubMed: 22458294]
- Glurich I, Nycz G, Acharya A. Status update on translation of integrated primary dental-medical care delivery for management of diabetic patients. Clinical Medicine & Research. 2017;15(1–2):21–32. [PMC free article: PMC5572842] [PubMed: 28373288]
- Glurich I, Schwei KM, Lindberg S, Shimpi N, Acharya A. Integrating medical-dental care for diabetic patients: qualitative assessment of provider perspectives. Health Promotion Practice. 2018;19(4):531–41. [PMC free article: PMC5878963] [PubMed: 29073800]
- Glurich I, Shimpi N, Acharya A. Interdisciplinary Care Model: Chronic Kidney Disease and Oral Health. In: Acharya A, Powell V, Torres-Urquidy M, Posteraro R, Thyvalikakath T, eds. Integration of Medical and Dental Care and Patient Data. 2nd ed. London: Springer International Publishing; 2019:87–107.
- Gold M, McLaughlin C. Assessing HITECH implementation and lessons: 5 years later. Milbank Quarterly. 2016;94(3):654–87. [PMC free article: PMC5020152] [PubMed: 27620687]
- Gomes ME, Rodrigues MT, Domingues RMA, Reis RL. Tissue engineering and regenerative medicine: new trends and directions – a year in review. Tissue Engineering Part B: Reviews. 2017;23(3):211–24. [PubMed: 28457175]
- Gomez A, Espinoza JL, Harkins DM et al. Host genetic control of the oral microbiome in health and disease. Cell Host & Microbe. 2017;22(3):269–78. [PMC free article: PMC5733791] [PubMed: 28910633]
- Gomez A, Nelson KE. The oral microbiome of children: development, disease, and implications beyond oral health. Microbial Ecology. 2017;73(2):492–503. [PMC free article: PMC5274568] [PubMed: 27628595]
- González Guzmán JF, Ohara AT. Evaluation of three-dimensional printed virtual setups. American Journal of Orthodontics and Dentofacial Orthopedics. 2019;155(2):288–95. [PubMed: 30712700]
- Gorres KL, Daigle D, Mohanram S, Miller G. Activation and repression of Epstein-Barr Virus and Kaposi’s sarcoma-associated herpesvirus lytic cycles by short- and medium-chain fatty acids. Journal of Virology. 2014;88(14):8028–44. [PMC free article: PMC4097796] [PubMed: 24807711]
- Göstemeyer G, Baker SR, Schwendicke F. Barriers and facilitators for provision of oral health care in dependent older people: a systematic review. Clinical Oral Investigations. 2019;23(3):979–993. [PubMed: 30707299]
- Graves DT, Correa JD, Silva TA. The oral microbiota is modified by systemic diseases. Journal of Dental Research. 2019;98(2):148–56. [PMC free article: PMC6761737] [PubMed: 30359170]
- Green LW. Public health asks of systems science: to advance our evidence-based practice, can you help us get more practice-based evidence? American Journal of Public Health. 2006;96(3):406–9. [PMC free article: PMC1470512] [PubMed: 16449580]
- Griffen AL, Beall CJ, Campbell JH et al. Distinct and complex bacterial profiles in human periodontitis and health revealed by 16S pyrosequencing. The ISME Journal. 2012;6(6):1176–85. [PMC free article: PMC3358035] [PubMed: 22170420]
- Griffen AL, Beall CJ, Firestone ND et al. CORE: a phylogenetically-curated 16S rDNA database of the core oral microbiome. PLoS One. 2011;6(4):e19051. [PMC free article: PMC3081323] [PubMed: 21544197]
- Gross EL, Beall CJ, Kutsch SR, Firestone ND, Leys EJ, Griffen AL. Beyond Streptococcus mutans: Dental caries onset linked to multiple species by 16S rRNA community analysis. PLoS One. 2012;7(10):e47722. [PMC free article: PMC3472979] [PubMed: 23091642]
- Guhr A, Kobold S, Seltmann S, Seiler Wulczyn AEM, Kurtz A, Loser P. Recent trends in research with human pluripotent stem cells: impact of research and use of cell lines in experimental research and clinical trials. Stem Cell Reports. 2018;11(2):485–496. [PMC free article: PMC6092712] [PubMed: 30033087]
- Gulshan V, Peng L, Coram M et al. Development and validation of a Deep Learning Algorithm for detection of diabetic retinopathy in retinal fundus photographs. Journal of the American Medical Association. 2016;316(22):2402–10. [PubMed: 27898976]
- Guo DC, Regalado ES, Pinard A et al. LTBP3 pathogenic variants predispose individuals to thoracic aortic aneurysms and dissections. American Journal of Human Genetics. 2018;102(4):706–12. [PMC free article: PMC5985335] [PubMed: 29625025]
- Haaland OA, Lie RT, Romanowska J, Gjerdevik M, Gjessing HK, Jugessur A. A Genome-wide search for gene-environment effects in isolated cleft lip with or without cleft palate triads points to an interaction between maternal periconceptional vitamin use and variants in ESRRG. Frontiers in Genetics. 2018;9:60. [PMC free article: PMC5834486] [PubMed: 29535761]
- Haden NK, Weaver RG, Valachovic RW. Meeting the demand for future dental school faculty: trends, challenges, and responses. Journal of Dental Education. 2002;66(9):1102–13. [PubMed: 12374272]
- Hafshejani TM, Zamanian A, Venugopal JR et al. Antibacterial glass-ionomer cement restorative materials: a critical review on the current status of extended release formulations. Journal of Controlled Release. 2017;262:317–28. [PubMed: 28774841]
- Hall KL, Vogel AL, Huang GC et al. The science of team science: a review of the empirical evidence and research gaps on collaboration in science. American Psychologist. 2018;73(4):532–48. [PubMed: 29792466]
- Hannig M, Hannig C. Nanotechnology and its role in caries therapy. Advances in Dental Research. 2012;24(2):53–7. [PubMed: 22899680]
- Harris A. The disciplines where no black people earn PhDs. The Atlantic. 2019 (April 19).
- Harun WSW, Manam MS, Kamariah MSIN et al. A review of powdered additive manufacturing techniques for Ti-6al-4v biomedical applications. Powder Technology. 2018;331:74–97.
- Hashim D, Cionca N. A comprehensive review of peri-implantitis risk factors. Current Oral Health Reports. 2020;7(3):262–73.
- Hasin Y, Seldin M, Lusis A. Multi-omics approaches to disease. Genome Biology. 2017;18(1):83. [PMC free article: PMC5418815] [PubMed: 28476144]
- Haworth S, Shungin D, van der Tas JT et al. Consortium-based genome-wide meta-analysis for childhood dental caries traits. Human Molecular Genetics. 2018;27(17):3113–27. [PMC free article: PMC6097157] [PubMed: 29931343]
- Hayes RB, Ahn J, Fan X et al. Association of oral microbiome with risk for incident head and neck squamous cell cancer. JAMA Oncology. 2018;4(3):358–65. [PMC free article: PMC5885828] [PubMed: 29327043]
- Heath BR, Michmerhuizen NL, Donnelly CR et al. Head and neck cancer immunotherapy beyond the Checkpoint blockade. Journal of Dental Research. 2019;98(10):1073–80. [PMC free article: PMC6704427] [PubMed: 31340724]
- Hegde HKR, Shimpi N, Glurich I, Panny A, Acharya A. Diabetes Mellitus prediction using machine learning in a dental setting. Paper presented at: 46th Annual Meeting of the American Association for Dental Research 2017; Ft. Lauderdale, FL.
- Hench LL. The future of bioactive ceramics. Journal of Materials Science: Materials in Medicine. 2015;26(2):86. [PubMed: 25644100]
- Hench LL, Polak JM. Third-generation biomedical materials. Science. 2002;295(5557):1014–17. [PubMed: 11834817]
- Herzog CR, Berzins DW, DenBesten P et al. Oral Sciences PhD program enrollment, graduates, and placement: 1994 to 2016. Journal of Dental Research. 2018;97(5):483–91. [PMC free article: PMC6728576] [PubMed: 29328868]
- Hilton TJ, Funkhouser E, Ferracane JL et al. Symptom changes and crack progression in untreated cracked teeth: One-year findings from the National Dental Practice-Based Research Network. Journal of Dentistry. 2020;93:103269. [PMC free article: PMC7255638] [PubMed: 31899264]
- Hirasuna K, Fried D, Darling CL. Near-infrared imaging of developmental defects in dental enamel. Journal of Biomedical Optics. 2008;13(4):044011. [PubMed: 19021339]
- Ho BV, Weijenberg RAF, van der Maarel-Wierink CD et al. Effectiveness of the implementation project ‘Don’t forget the mouth!’ of community dwelling older people with dementia: a prospective longitudinal single-blind multicentre study protocol (DFTM!). BMC Oral Health. 2019;19(1):91. [PMC free article: PMC6537198] [PubMed: 31138273]
- Honda K, Littman DR. The microbiota in adaptive immune homeostasis and disease. Nature. 2016;535(7610):75–84. [PubMed: 27383982]
- Hormia M, Willberg J, Ruokonen H, Syrjänen S. Marginal periodontium as a potential reservoir of human papillomavirus in oral mucosa. Journal of Periodontology. 2005;76(3):358–63. [PubMed: 15857068]
- Horst JA, Heima M. Prevention of dental caries by Silver Diamine Fluoride. Compendium of Continuing Education in Dentistry. 2019;40(3):158–63. [PubMed: 30829497]
- Horvath Z, Albani SE, Wankiiri-Hale C. Training future dentists for an academic career: a three-tiered model. Journal of Dental Education. 2016;80(5):502–16. [PubMed: 27139201]
- Hotamisligil GS. Inflammation and metabolic disorders. Nature. 2006;444(7121):860–7. [PubMed: 17167474]
- Howe MS, Keys W, Richards D. Long-term (10-year) dental implant survival: a systematic review and sensitivity meta-analysis. Journal of Dentistry. 2019;84:9–21. [PubMed: 30904559]
- Hrubešová K, Fousková M, Habartová L et al. Search for biomarkers of Alzheimer’s disease: recent insights, current challenges and future prospects. Clinical Biochemistry. 2019;72:39–51. [PubMed: 30953619]
- Huang GT, Gronthos S, Shi S. Mesenchymal stem cells derived from dental tissues vs. those from other sources: their biology and role in regenerative medicine. Journal of Dental Research. 2009;88(9):792–806. [PMC free article: PMC2830488] [PubMed: 19767575]
- Huang N, Pérez P, Kato T et al. SARS-CoV-2 infection of the oral cavity and saliva. Nature Medicine. 2021;27:892–903. [PMC free article: PMC8240394] [PubMed: 33767405]
- Human Microbiome Project Consortium. Structure, function and diversity of the healthy human microbiome. Nature. 2012a;486(7402):207–14. [PMC free article: PMC3564958] [PubMed: 22699609]
- Human Microbiome Project Consortium. A framework for human microbiome research. Nature. 2012b;486(7402):215–21. [PMC free article: PMC3377744] [PubMed: 22699610]
- Imabayashi Y, Moriyama M, Takeshita T et al. Molecular analysis of fungal populations in patients with oral candidiasis using next-generation sequencing. Scientific Reports. 2016;6:28110. [PMC free article: PMC4910111] [PubMed: 27305838]
- Imazato S. Bio-active restorative materials with antibacterial effects: new dimension of innovation in restorative dentistry. Dental Materials Journal. 2009;28(1):11–19. [PubMed: 19280964]
- Institute for Healthcare Improvement. The Breakthrough Series: IHI’s Collaborative Model for Achieving Breakthrough Improvement. IHI Innovation Series white paper. Boston, MA: Institute for Healthcare Improvement; 2003. http://www
.ihi.org/resources /Pages/IHIWhitePapers /TheBreakthroughSeriesIHIsCollaborativeModelforAchievingBreakthroughImprovement.aspx. Accessed June 25, 2021. - Institute of Medicine, Roundtable on Evidence-Based Medicine. Preface. In: Olsen LA AD, McGinnis JM, ed. The Learning Healthcare System: Workshop Summary. Washington, DC: The National Academies Press; 2007. [PubMed: 21452449]
- Intarak N, Theerapanon T, Thaweesapphithak S, Suphapeetiporn K, Porntaveetus T, Shotelersuk V. Genotype-phenotype correlation and expansion of orodental anomalies in LTBP3-related disorders. Molecular Genetics and Genomics. 2019;294(3):773–87. [PubMed: 30887145]
- Ioannidis JPA, Khoury MJ. Evidence-based medicine and big genomic data. Human Molecular Genetics. 2018;27(R1):R2–7. [PMC free article: PMC6247896] [PubMed: 29474574]
- Ioannidou E, Letra A, Shaddox LM et al. Empowering women researchers in the new century: IADR’s strategic direction. Advances in Dental Research. 2019;30(3):69–77. [PMC free article: PMC7364444] [PubMed: 31746653]
- Irish JM, Kotecha N, Nolan GP. Mapping normal and cancer cell signalling networks: towards single-cell proteomics. Nature Reviews Cancer. 2006;6(2):146–55. [PubMed: 16491074]
- Irving M, Stewart R, Spallek H, Blinkhorn A. Using teledentistry in clinical practice as an enabler to improve access to clinical care: a qualitative systematic review. Journal of Telemedicine and Telecare. 2018;24(3):129–46. [PubMed: 28092220]
- Isaza-Guzmán DM, Medina-Piedrahíta VM, Gutiérrez-Henao C, Tobón-Arroyave SI. Salivary levels of NLRP3 inflammasome-related proteins as potential biomarkers of periodontal clinical status. Journal of Periodontology. 2017;88(12):1329–38. [PubMed: 28691886]
- Ismail AI, Tellez M, Pitts NB et al. Caries management pathways preserve dental tissues and promote oral health. Community Dentistry and Oral Epidemiology. 2013;41(1):e12–40. [PubMed: 24916676]
- Istrate EC, Slapar FJ, Mallarapu M, Stewart DCL, West KP. Dentists of tomorrow 2020: an analysis of the results of the 2020 ADEA Survey of U.S. Dental School Seniors. Journal of Dental Education. 2021;85(3):427–40. [PubMed: 33638174]
- Ivanovski S, Vaquette C, Gronthos S, Hutmacher DW, Bartold PM. Multiphasic scaffolds for periodontal tissue engineering. Journal of Dental Research. 2014;93(12):1212–21. [PMC free article: PMC4462800] [PubMed: 25139362]
- Jedeon K, De la Dure-Molla M, Brookes SJ et al. Enamel defects reflect perinatal exposure to bisphenol A. American Journal of Pathology. 2013;183(1):108–18. [PMC free article: PMC3703547] [PubMed: 23764278]
- Jefferies SR. Bioactive and biomimetic restorative materials: a comprehensive review. Part I. Journal of Esthetic and Restorative Dentistry. 2014;26(1):14–26. [PubMed: 24341542]
- Jha AIA, Chaoui A, Defossex S, Bombaugh M, Miller Y. A Crisis in Health Care: A Call to Action on Physician Burnout. Waltham, MA: Massacusetts Medical Society; 2018.
- Jia S, Zhou J, Fanelli C et al. Small-molecule Wnt agonists correct cleft palates in Pax9 mutant mice in utero. Development. 2017a;144(20):3819–28. [PMC free article: PMC5675451] [PubMed: 28893947]
- Jia S, Zhou J, Wee Y, Mikkola ML, Schneider P, D’Souza RN. Anti-EDAR agonist antibody therapy resolves palate defects in Pax9-/- Mice. Journal of Dental Research. 2017b;96(11):1282–9. [PMC free article: PMC5613884] [PubMed: 28813171]
- Joachims ML, Leehan KM, Lawrence C et al. Single-cell analysis of glandular T cell receptors in Sjögren’s syndrome. JCI Insight. 2016;1(8). [PMC free article: PMC4922426] [PubMed: 27358913]
- Johnson L, Genco RJ, Damsky C et al. Genetics and its implications for clinical dental practice and education: report of panel 3 of the Macy study. Journal of Dental Education. 2008;72:86–94. [PubMed: 18250384]
- Johnston CD, Cotton SL, Rittling SR et al. Systematic evasion of the restriction-modification barrier in bacteria. Proceedings of the National Academy of Sciences. 2019;116(23):11454–9. [PMC free article: PMC6561282] [PubMed: 31097593]
- Jones HP, McGee R, Weber-Main AM et al. Enhancing research careers: an example of a U.S. national diversity-focused, grant-writing training and coaching experiment. BMC Proceedings. 2017;11:16. [PMC free article: PMC5773888] [PubMed: 29375659]
- Jones PA, Ohtani H, Chakravarthy A, De Carvalho DD. Epigenetic therapy in immune-oncology. Nature Reviews Cancer. 2019;19(3):151–61. [PubMed: 30723290]
- Joshipura K, Ritchie C, Douglass C. Strength of evidence linking oral conditions and systemic disease. Compendium of Continuing Education in Dentistry. 2000(30):12–23. [PubMed: 11908384]
- Jukam D, Limouse C, Smith OK, Risca VI, Bell JC, Straight AF. Chromatin-associated RNA sequencing (ChAR-seq). Current Protocols in Molecular Biology. 2019;126(1):e87. [PMC free article: PMC7670654] [PubMed: 30786161]
- Junker JP, van Oudenaarden A. Every cell is special: genome-wide studies add a new dimension to single-cell biology. Cell. 2014;157(1):8–11. [PubMed: 24679522]
- Kaiser J. Senate bill would give NIH $3 billion in 2020, or 7.7% boost. Science. Washington, DC: American Association for the Advancement of Science; 2019.
- Kang HW, Lee SJ, Ko IK, Kengla C, Yoo JJ, Atala A. A 3D bioprinting system to produce human-scale tissue constructs with structural integrity. Nature Biotechnology. 2016;34(3):312–19. [PubMed: 26878319]
- Karring T, Nyman S, Gottlow J, Laurell L. Development of the biological concept of guided tissue regeneration—animal and human studies. Periodontology 2000. 1993;1(1):26–35. [PubMed: 8401858]
- Kashyap PC, Marcobal A, Ursell LK et al. Complex interactions among diet, gastrointestinal transit, and gut microbiota in humanized mice. Gastroenterology. 2013;144(5):967–77. [PMC free article: PMC3890323] [PubMed: 23380084]
- Keehan SP, Cuckler GA, Poisal JA et al. National health expenditure projections, 2019–28: expected rebound in prices drives rising spending growth. Health Affairs. 2020;39(4):704–14. [PubMed: 32207998]
- Kennedy JE. Faculty status in a climate of change. Journal of Dental Education. 1990;54(5):268–72. [PubMed: 2335658]
- Kennedy JE. A fifteen year perspective on dental school faculty. Journal of Dental Education. 1995;59(5):578–83. [PubMed: 7636045]
- Khalafalla MG, Woods LT, Camden JM et al. P2X7 receptor antagonism prevents IL-1beta release from salivary epithelial cells and reduces inflammation in a mouse model of autoimmune exocrinopathy. Journal of Biological Chemistry. 2017;292(40):16626–37. [PMC free article: PMC5633125] [PubMed: 28798231]
- Kocak N, Cengiz-Yanardag E. Clinical performance of clinical-visual examination, digital bitewing radiography, laser fluorescence, and near-infrared light transillumination for detection of non-cavitated proximal enamel and dentin caries. Lasers in Medical Science. 2020;35(7):1621–8. [PubMed: 32333336]
- Konig K, Schneckenburger H, Hibst R. Time-gated in vivo autofluorescence imaging of dental caries. Cellular and Molecular Biology (Noisy-le-grand). 1999;45(2):233–9. [PubMed: 10230733]
- Koo H, Allan RN, Howlin RP, Stoodley P, Hall-Stoodley L. Targeting microbial biofilms: current and prospective therapeutic strategies. Nature Reviews Microbiology. 2017;15(12):740–55. [PMC free article: PMC5685531] [PubMed: 28944770]
- Koop R, Merheb J, Quirynen M. Periodontal regeneration with enamel matrix derivative in reconstructive periodontal therapy: a systematic review. Journal of Periodontology. 2012;83(6):707–20. [PubMed: 22050544]
- Kopperud SE, Tveit AB, Gaarden T, Sandvik L, Espelid I. Longevity of posterior dental restorations and reasons for failure. European Journal of Oral Sciences. 2012;120(6):539–48. [PubMed: 23167471]
- Kopycka-Kedzierawski DT, Bell CH, Billings RJ. Prevalence of dental caries in Early Head Start children as diagnosed using teledentistry. Pediatric Dentistry. 2008;30(4):329–33. [PubMed: 18767513]
- Kornman KS. Interleukin 1 genetics, inflammatory mechanisms, and nutrigenetic opportunities to modulate diseases of aging. American Journal of Clinical Nutrition. 2006;83(2):475–83S. [PubMed: 16470016]
- Koruyucu M, Seymen F, Gencay G et al. Nephrocalcinosis in amelogenesis imperfecta caused by the FAM20A mutation. Nephron. 2018;139(2):189–96. [PubMed: 29439260]
- Kostic AD, Chun E, Robertson L et al. Fusobacterium nucleatum potentiates intestinal tumorigenesis and modulates the tumor-immune microenvironment. Cell Host & Microbe. 2013;14(2):207–15. [PMC free article: PMC3772512] [PubMed: 23954159]
- Kostic AD, Gevers D, Pedamallu CS et al. Genomic analysis identifies association of Fusobacterium with colorectal carcinoma. Genome Research. 2012;22(2):292–8. [PMC free article: PMC3266036] [PubMed: 22009990]
- Kvedar J, Coye MJ, Everett W. Connected health: a review of technologies and strategies to improve patient care with telemedicine and telehealth. Health Affairs. 2014;33(2):194–9. [PubMed: 24493760]
- Lalla RV, Patton LL, Dongari-Bagtzoglou A. Oral candidiasis: pathogenesis, clinical presentation, diagnosis and treatment strategies. Journal of the California Dental Association. 2013;41(4):263–8. [PubMed: 23705242]
- Lamkanfi M, Dixit VM. In retrospect: the inflammasome turns 15. Nature. 2017;548(7669):534–5. [PubMed: 28858314]
- Lammi L, Arte S, Somer M et al. Mutations in AXIN2 cause familial tooth agenesis and predispose to colorectal cancer. American Journal of Human Genetics. 2004;74(5):1043–50. [PMC free article: PMC1181967] [PubMed: 15042511]
- Lamont RJ, Koo H, Hajishengallis G. The oral microbiota: dynamic communities and host interactions. Nature Reviews Microbiology. 2018;16(12):745–59. [PMC free article: PMC6278837] [PubMed: 30301974]
- Landes SJ, McBain SA, Curran GM. An introduction to effectiveness-implementation hybrid designs. Psychiatry Research. 2019;280:112513. [PMC free article: PMC6779135] [PubMed: 31434011]
- Larkin LM, Calve S, Kostrominova TY, Arruda EM. Structure and functional evaluation of tendon-skeletal muscle constructs engineered in vitro. Tissue Engineering. 2006;12(11):3149–58. [PMC free article: PMC2798802] [PubMed: 17518629]
- Larsson L, Castilho RM, Giannobile WV. Epigenetics and its role in periodontal diseases: a state-of-the-art review. Journal of Periodontology. 2015;86(4):556–8. [PubMed: 25415244]
- Larsson L, Decker AM, Nibali L, Pilipchuk SP, Berglundh T, Giannobile WV. Regenerative medicine for periodontal and peri-implant diseases. Journal of Dental Research. 2016;95(3):255–66. [PMC free article: PMC4766955] [PubMed: 26608580]
- Lee CH, Chen YW, Tu YK, Wu YC, Chang PC. The potential of salivary biomarkers for predicting the sensitivity and monitoring the response to nonsurgical periodontal therapy: a preliminary assessment. Journal of Periodontal Research. 2018;53(4):545–54. [PubMed: 29882262]
- Lee JY, Divaris K. The ethical imperative of addressing oral health disparities: a unifying framework. Journal of Dental Research. 2014;93(3):224–30. [PMC free article: PMC3929974] [PubMed: 24189268]
- Leslie EJ, Carlson JC, Shaffer JR et al. Genome-wide meta-analyses of nonsyndromic orofacial clefts identify novel associations between FOXE1 and all orofacial clefts, and TP63 and cleft lip with or without cleft palate. Human Genetics. 2017;136(3):275–86. [PMC free article: PMC5317097] [PubMed: 28054174]
- Leslie EJ, Carlson JC, Shaffer JR et al. A multi-ethnic genome-wide association study identifies novel loci for non-syndromic cleft lip with or without cleft palate on 2p24.2, 17q23 and 19q13. Human Molecular Genetics. 2016;25(13):2862–72. [PMC free article: PMC5181632] [PubMed: 27033726]
- Levy BD, Clish CB, Schmidt B, Gronert K, Serhan CN. Lipid mediator class switching during acute inflammation: signals in resolution. Nature Immunology. 2001;2(7):612–19. [PubMed: 11429545]
- Li SM, Zou J, Wang Z, Wright JT, Zhang Y. Quantitative assessment of enamel hypomineralization by KaVo DIAGNOdent at different sites on first permanent molars of children in China. Pediatric Dentistry. 2003;25(5):485–90. [PubMed: 14649613]
- Lif Holgerson P, Harnevik L, Hernell O, Tanner AC, Johansson I. Mode of birth delivery affects oral microbiota in infants. Journal of Dental Research. 2011;90(10):1183–8. [PMC free article: PMC3173012] [PubMed: 21828355]
- Ligon SC, Liska R, Stampfl J, Gurr M, Mulhaupt R. Polymers for 3D printing and customized additive manufacturing. Chemical Reviews. 2017;117(15):10212–90. [PMC free article: PMC5553103] [PubMed: 28756658]
- Liu CJ, Chen JH, Hsia SM et al. Salivary LDOC1 is a gender-difference biomarker of oral squamous cell carcinoma. PeerJ. 2019;7:e6732. [PMC free article: PMC6461027] [PubMed: 30993049]
- Liu YI, Rubin DL. The role of informatics in health care reform. Academic Radiology. 2012;19(9):1094–9. [PMC free article: PMC3416921] [PubMed: 22771052]
- Lo SJ, Yao DJ. Get to understand more from single-cells: current studies of microfluidic-based techniques for single-cell analysis. International Journal of Molecular Sciences. 2015;16(8):16763–77. [PMC free article: PMC4581168] [PubMed: 26213918]
- Louie T, Lee C, Hsu D et al. Clinical assessment of early tooth demineralization using polarization sensitive optical coherence tomography. Lasers in Surgery and Medicine. 2010;42(10):738–45. [PMC free article: PMC3058417] [PubMed: 21246578]
- Lu CY, Williams MS, Ginsburg GS, Toh S, Brown JS, Khoury MJ. A proposed approach to accelerate evidence generation for genomic-based technologies in the context of a learning health system. Genetic Medicine. 2018;20(4):390–6. [PubMed: 28796238]
- Lussi A, Hibst R, Paulus R. DIAGNOdent: an optical method for caries detection. Journal of Dental Research. 2004;83(Spec No C):C80–83. [PubMed: 15286128]
- Macaulay IC, Voet T. Single cell genomics: advances and future perspectives. PLoS Genetics. 2014;10(1):e1004126. [PMC free article: PMC3907301] [PubMed: 24497842]
- Majno G. Chronic inflammation: links with angiogenesis and wound healing. American Journal of Pathology. 1998;153(4):1035–9. [PMC free article: PMC1853039] [PubMed: 9777935]
- Majno G, Joris I. Cells, Tissues, and Disease: Principles of General Pathology. New York: Oxford University Press; 2004.
- Makvandi P, Jamaledin R, Jabbari M, Nikfarjam N, Borzacchiello A. Antibacterial quaternary ammonium compounds in dental materials: a systematic review. Dental Materials. 2018;34:851–67. [PubMed: 29678327]
- Mantero JC, Kishore N, Ziemek J et al. Randomised, double-blind, placebo-controlled trial of IL1-trap, rilonacept, in systemic sclerosis. A phase I/II biomarker trial. Clinical and Experimental Rheumatology. 2018;36(4):146–9. [PubMed: 30277862]
- Manukian G, Bar-Ad V, Lu B, Argiris A, Johnson JM. Combining radiation and immune checkpoint blockade in the treatment of head and neck squamous cell carcinoma. Frontiers in Oncology. 2019;9:122. [PMC free article: PMC6414812] [PubMed: 30895168]
- Mark Welch JL, Rossetti BJ, Rieken CW, Dewhirst FE, Borisy GG. Biogeography of a human oral microbiome at the micron scale. Proceedings of the National Academy of Science. 2016;113(6):E791–800. [PMC free article: PMC4760785] [PubMed: 26811460]
- Martin I, Galipeau J, Kessler C, Le Blanc K, Dazzi F. Challenges for mesenchymal stromal cell therapies. Science Translational Medicine. 2019;11(480). [PubMed: 30787168]
- Martinon F, Burns K, Tschopp J. The inflammasome: a molecular platform triggering activation of inflammatory caspases and processing of proIL-beta. Molecular Cell. 2002;10(2):417–26. [PubMed: 12191486]
- Mason MR, Chambers S, Dabdoub SM, Thikkurissy S, Kumar PS. Characterizing oral microbial communities across dentition states and colonization niches. Microbiome. 2018;6(1):67. [PMC free article: PMC5891995] [PubMed: 29631628]
- Mason MR, Nagaraja HN, Camerlengo T, Joshi V, Kumar PS. Deep sequencing identified ethnicity-specific bacterial signatures in the oral microbiome. PLoS One. 2013;8:e77287. [PMC free article: PMC3806732] [PubMed: 24194878]
- Mason MR, Preshaw PM, Nagaraja HN, Dabdoub SM, Rahman A, Kumar PS. The subgingival microbiome of clinically healthy current and never smokers. The ISME Journal. 2015;9(1):268–72. [PMC free article: PMC4274424] [PubMed: 25012901]
- McGrady MG, Ellwood RP, Taylor A et al. Evaluating the use of fluorescent imaging for the quantification of dental fluorosis. BMC Oral Health. 2012;12:47. [PMC free article: PMC3549943] [PubMed: 23116324]
- Mealey BL. Periodontal disease and diabetes. A two-way street. Journal of the American Dental Association. 2006;137(Suppl):26–31S. [PubMed: 17012733]
- Medema MH, Cimermancic P, Sali A, Takano E, Fischbach MA. A systematic computational analysis of biosynthetic gene cluster evolution: lessons for engineering biosynthesis. PLoS Computational Biology. 2014;10(12):e1004016. [PMC free article: PMC4256081] [PubMed: 25474254]
- Medzhitov R. Inflammation 2010: new adventures of an old flame. Cell. 2010;140(6):771–6. [PubMed: 20303867]
- Meereis CTW, Münchow EA, de Oliveira da Rosa WL, da Silva AF, Piva E. Polymerization shrinkage stress of resin-based dental materials: a systematic review and meta-analyses of composition strategies. Journal of the Mechanical Behavior of Biomedical Materials. 2018;82:268–81. [PubMed: 29627738]
- Melkers J, Hicks D, Isett KR et al. Preferences for peer-reviewed versus other publication sources: a survey of general dentists in the National Dental PBRN. Implementation Science. 2019;14(1):19. [PMC free article: PMC6397483] [PubMed: 30823931]
- Meng L, Hua F, Bian Z. Coronavirus disease 2019 (COVID-19): emerging and future challenges for dental and oral medicine. Journal of Dental Research. 2020;99(5):481–7. [PMC free article: PMC7140973] [PubMed: 32162995]
- Meuric V, Le Gall-David S, Boyer E et al. Signature of microbial dysbiosis in periodontitis. Applied and Environmental Microbiology. 2017;83(14). [PMC free article: PMC5494626] [PubMed: 28476771]
- Milshteyn A, Colosimo DA, Brady SF. Accessing bioactive natural products from the human microbiome. Cell Host & Microbe. 2018;23(6):725–36. [PMC free article: PMC7232905] [PubMed: 29902438]
- Monteiro N, Yelick PC. Advances and perspectives in tooth tissue engineering. Journal of Tissue Engineering and Regenerative Medicine. 2017;11(9):2443–61. [PMC free article: PMC6625321] [PubMed: 27151766]
- Morris TL, Arnold RR, Webster-Cyriaque J. Signaling cascades triggered by bacterial metabolic end products during reactivation of Kaposi’s sarcoma-associated herpesvirus. Journal of Virology. 2007;81(11):6032–42. [PMC free article: PMC1900322] [PubMed: 17376930]
- Moutsopoulos NM, Konkel J, Sarmadi M et al. Defective neutrophil recruitment in leukocyte adhesion deficiency type I disease causes local IL-17-driven inflammatory bone loss. Science Translational Medicine. 2014;6(229):229RA240. [PMC free article: PMC4090608] [PubMed: 24670684]
- Mukherjee C, Moyer CO, Steinkamp HM et al. Acquisition of oral microbiota is driven by environment, not host genetics. Microbiome. 2021;9(1):54. [PMC free article: PMC7903647] [PubMed: 33622378]
- Mungia R, Funkhouser E, Buchberg Trejo MK et al. Practitioner participation in national Dental Practice-based Research Network (PBRN) studies: 12-Year results. Journal of the American Board of Family Medicine. 2018;31(6):844–56. [PMC free article: PMC6936735] [PubMed: 30413541]
- Mureau MA, Hofer SO. Maximizing results in reconstruction of cheek defects. Clinics in Plastic Surgery. 2009;36(3):461–76. [PubMed: 19505614]
- Murphy SV, Atala A. 3D bioprinting of tissues and organs. Nature Biotechnology. 2014;32(8):773–85. [PubMed: 25093879]
- Nagarajan N, Dupret-Bories A, Karabulut E, Zorlutuna P, Vrana NE. Enabling personalized implant and controllable biosystem development through 3D printing. Biotechnology Advances. 2018;36(2):521–533. [PubMed: 29428560]
- Napoles AM, Stewart AL. Transcreation: an implementation science framework for community-engaged behavioral interventions to reduce health disparities. BMC Health Services Research. 2018;18(1):710. [PMC free article: PMC6134771] [PubMed: 30208873]
- National Academies of Sciences, Engineering, and Medicine. The Next Generation of Biomedical and Behavioral Sciences Researchers: Breaking Through. Washington, DC: The National Academies Press; 2018a. https://www
.nap.edu/catalog /25008/the-next-generation-of-biomedical-and-behavioral-sciences-researchers-breaking. Accessed June 25, 2021. [PubMed: 29664586] - National Academies of Sciences, Engineering, and Medicine. Sexual Harassment of Women: Climate, Culture, and Consequences in Academic Sciences, Engineering, and Medicine. 2018b. https://www
.nap.edu/catalog /24994/sexual-harassment-of-women-climate-culture-and-consequences-in-academic. Accessed July 13, 2021. [PubMed: 29894119] - National Academy of Engineering. Engineering a Learning Healthcare System: A Look at the Future: Workshop Summary. Washington, DC: The National Academies Press; 2011. [PubMed: 21977540]
- National Cancer Institute. Cancer Moonshot. 2020. https://www
.cancer.gov /research/key-initiatives /moonshot-cancer-initiative. Accessed June 25, 2021. - GenBank. National Institutes of Health; 2019. https://www
.ncbi.nlm.nih.gov/genbank/. Accessed June 25, 2021. - National Center for Complementary and Integrative Health. Complementary, Alternative, or Integrative Health: What’s In a Name? 2021. https://www
.nccih.nih .gov/health/complementary-alternative-or-integrative-health-whats-in-a-name. Accessed June 25, 2021. - National Center for Health Statistics, Centers for Disease Control and Prevention. NCHS Data Linkage Activities. 2020. https://www
.cdc.gov/nchs /data-linkage/index.htm. Accessed June 25, 2021. - National Institute of General Medical Sciences. Enhancing the Diversity of the NIH-Funded Workforce. 2021a. https://www
.nigms.nih.gov/training/dpc/. Accessed June 15, 2021. - National Institute of General Medical Sciences. Building Infrastructure Leading to Diversity (BUILD) Initiative. 2021b. https://www
.nigms.nih .gov/training/dpc/pages/build.aspx. Accessed June 25, 2021. - National Institute on Minority Health and Health Disparities. NIMHD Research Framework. 2018. https://www
.nimhd.nih .gov/docs/research_framework /research-framework-slide.pdf. Accessed June 25, 2021. - National Institutes of Health. Physician-Scientist Workforce Working Group Report. 2014. https://acd
.od.nih.gov /documents/reports /PSW_Report_ACD_06042014.pdf. Accessed June 25, 2021. - National Institutes of Health. PhenX Social Determinants of Health Assessments Collection. 2020a. https://www
.nimhd.nih .gov/programs/collab/phenx/index.html. Accessed June 25, 2021. - National Institutes of Health. 21st Century Cures Act. 2020b. https://www
.nih.gov/research-training /medical-research-initiatives/cures. Accessed June 25, 2021. - National Institutes of Health. About the NIH Common Fund. 2021a. https://commonfund
.nih.gov/ Accessed June 25, 2021. - National Institutes of Health. All of Us Research Program Overview. 2021b. https://allofus
.nih.gov /about/all-us-research-program-overview. Accessed June 25, 2021. - National Library of Medicine, National Institutes of Health. The Genome Reference Consortium. 2021a. https://www
.ncbi.nlm.nih.gov/grc. Accessed June 25, 2021. - National Library of Medicine, National Institutes of Health. GenBank. 2021b. https://www
.ncbi.nlm.nih.gov/genbank/. Accessed June 25, 2021. - National Library of Medicine, National Institutes of Health. RefSeq: NCBI Reference Sequence Database. 2021c. https://www
.nchi.nlm.nih.gov/refseq/. Accessed September 16, 2021. - National Library of Medicine, Unified Medical Language System (UMLS). 2021d. https://www
.nlm.nih.gov /research/umls/index.html. Accessed October 28, 2021. - National Library of Medicine, National Institutes of Health. ClinicalTrials
.gov. De-Implementing Opioids for Dental Extractions (DIODE). 2018 (July 12). https://clinicaltrials.gov/ct2/show/NCT03584789. Accessed June 25, 2021. - National Library of Medicine, National Institutes of Health. ClinicalTrials
.gov. Organizational Change in Dental Care. 2017 (December 8). https://clinicaltrials.gov/ct2/show/NCT03367416. Accessed June 25, 2021. - National Science Foundation. Women, Minorities, and Persons with Disabilities in Science and Engineering. Alexandria, VA: NSF; 2019. https://ncses
.nsf.gov /pubs/nsf19304/digest. Accessed June 25, 2021. - Neben CL, Roberts RR, Dipple KM, Merrill AE, Klein OD. Modeling craniofacial and skeletal congenital birth defects to advance therapies. Human Molecular Genetics. 2016;25(R2):R86–93. [PMC free article: PMC5036869] [PubMed: 27346519]
- Nevins M, Kao RT, McGuire MK et al. Platelet-derived growth factor promotes periodontal regeneration in localized osseous defects: 36-month extension results from a randomized, controlled, double-masked clinical trial. Journal of Periodontology. 2013;84(4):456–64. [PMC free article: PMC5715458] [PubMed: 22612364]
- Nichols K. Teledentistry overview: United States of America. Journal of the International Society for Telemedicine and eHealth. 2019;7(May):e9(1–6).
- Niller HH, Minarovits J. Patho-epigenetics of infectious diseases caused by intracellular bacteria. In: Niller HH, Minarovits J, eds. Patho-Epigenics of Infectious Disease. Vol 879. New York: Springer International Publishing; 2016:107–30. [PubMed: 26659266]
- Northridge ME, Metcalf SS, Yi S, Zhang Q, Gu X, Trinh-Shevrin C. A protocol for a feasibility and acceptability study of a participatory, multi-level, dynamic intervention in urban outreach centers to improve the oral health of low-income Chinese Americans. Frontiers in Public Health. 2018;6:29. [PMC free article: PMC5817910] [PubMed: 29492400]
- Norton WE, Lungeanu A, Chambers DA, Contractor N. Mapping the growing discipline of dissemination and implementation science in health. Scientometrics. 2017;112(3):1367–90. [PMC free article: PMC5729761] [PubMed: 29249842]
- Nycz G, Shimpi N, Glurich I, Ryan M, Sova G, Weiner S, Nichols L, Acharya A. Positioning operations in the dental safety net to enhance value-based care delivery in an integrated health-care setting. Journal of Public Health Dentistry. 2020 Sep;80 Suppl 2:S71–76. [PubMed: 32885424]
- Obermeyer Z, Powers B, Vogeli C, Mullainathan S. Dissecting racial bias in an algorithm used to manage the health of populations. Science. 2019;366(6464):447–53. [PubMed: 31649194]
- Oberoi G, Nitsch S, Edelmayer M, Janjic K, Muller AS, Agis H. 3D printing - encompassing the facets of dentistry. Frontiers in Bioengineering and Biotechnology. 2018;6:172. [PMC free article: PMC6262086] [PubMed: 30525032]
- Offenbacher S, Beck JD, Jared HL et al. Effects of periodontal therapy on rate of preterm delivery: a randomized controlled trial. Obstetrics & Gynecology. 2009;114(3):551–9. [PMC free article: PMC2917914] [PubMed: 19701034]
- Offenbacher S, Divaris K, Barros SP et al. Genome-wide association study of biologically informed periodontal complex traits offers novel insights into the genetic basis of periodontal disease. Human Molecular Genetics. 2016;25(10):2113–29. [PMC free article: PMC5062586] [PubMed: 26962152]
- Offenbacher S, Jiao Y, Kim SJ et al. GWAS for Interleukin-1beta levels in gingival crevicular fluid identifies IL37 variants in periodontal inflammation. Nature Communications. 2018;9(1):3686. [PMC free article: PMC6134146] [PubMed: 30206230]
- Office of Data Science Strategy, National Institutes of Health. About the NIH Cloud Platform Interoperability (NCPI) Effort. 2021. https://datascience
.nih .gov/nih-cloud-platform-interoperability-effort. Accessed September 20, 2021. - Ohno-Machado L, Kim J, Gabriel RA, Kuo GM, Hogarth MA. Genomics and electronic health record systems. Human Molecular Genetics. 2018;27(R1):R48–55. [PMC free article: PMC5946823] [PubMed: 29741693]
- Okahana H, Zhou E. Graduate enrollment and degrees: 2008 to 2018. Washington, DC: Council of Graduate Schools; 2019.
- Olsen I, Potempa J. Strategies for the inhibition of gingipains for the potential treatment of periodontitis and associated systemic diseases. Journal of Oral Microbiology. 6:1,24800. doi:10.3402/jom.v6.24800. [PMC free article: PMC4138498] [PubMed: 25206939] [CrossRef]
- Opdam NJ, van de Sande FH, Bronkhorst E et al. Longevity of posterior composite restorations: a systematic review and meta-analysis. Journal of Dental Research. 2014;93(10):943–9. [PMC free article: PMC4293707] [PubMed: 25048250]
- Ovretveit J, Nelson E, James B. Building a learning health system using clinical registers: a non-technical introduction. Journal of Health Organization and Management. 2016;30(7):1105–18. [PubMed: 27700477]
- Ozbolat IT, Hospodiuk M. Current advances and future perspectives in extrusion-based bioprinting. Biomaterials. 2016;76:321–43. [PubMed: 26561931]
- Palatta AM. From pipeline to mainstream: increasing the number of dental students and residents pursuing academic careers. Journal of Dental Education. 2016;80(5):499–501. [PubMed: 27139200]
- Pallos D, Hart PS, Cortelli JR et al. Novel COL1A1 mutation (G559C) [correction of G599C] associated with mild osteogenesis imperfecta and dentinogenesis imperfecta. Archives of Oral Biology. 2001;46(5):459–70. [PubMed: 11286811]
- Palmer S. Data Science for the C-Suite. New York: Digital Living Press, 2015.
- Passipieri JA, Christ GJ. The potential of combination therapeutics for more complete repair of volumetric muscle loss injuries: the role of exogenous growth factors and/or progenitor cells in implantable skeletal muscle tissue engineering technologies. Cells Tissues Organs. 2016;202(3–4):202–13. [PubMed: 27825153]
- Patel E, Pradeep P, Kumar P, Choonara YE, Pillay V. Oroactive dental biomaterials and their use in endodontic therapy. Journal of Biomedical Materials Research Part B: Applied Biomaterials. 2019;108(1):101–12. [PubMed: 30957440]
- Patil S, Rao RS, Majumdar B. Single gene disorders with craniofacial and oral manifestations. Journal of Contemporary Dental Practice. 2014;15(5):659–71. [PubMed: 25707843]
- Patrick DL, Burke LB, Powers JH et al. Patient-reported outcomes to support medical product labeling claims: FDA perspective. Value Health. 2007;10(Suppl 2):S125–37. [PubMed: 17995471]
- Paula AB, Laranjo M, Marto CM et al. Direct pulp capping: what is the most effective therapy? Systematic review and meta-analysis. Journal of Evidence-Based Dental Practice. 2018;18(4):298–14. [PubMed: 30514444]
- Peck J, Sedgley CM, Schwarz E, Replogle KJ. The impact of the Affordable Care Act on provision of endodontic services within a dental school setting in Oregon. Journal of Public Health Dentistry. 2019;79(2):175–80. [PubMed: 31020668]
- Pendergrass SA, Brown-Gentry K, Dudek SM et al. The use of phenome-wide association studies (PheWAS) for exploration of novel genotype-phenotype relationships and pleiotropy discovery. Genetic Epidemiology. 2011;35(5):410–22. [PMC free article: PMC3116446] [PubMed: 21594894]
- Peppas NA, Langer R. New challenges in biomaterials. Science. 1994;263(5154):1715–20. [PubMed: 8134835]
- Peumans M, De Munck J, Van Landuyt KL, Poitevin A, Lambrechts P, Van Meerbeek B. Eight-year clinical evaluation of a 2-step self-etch adhesive with and without selective enamel etching. Dental Materials. 2010;26(12):1176–84. [PubMed: 20947155]
- Pfeifer CS. Polymer-based direct filling materials. Dental Clinics of North America. 2017;61(4):733–50. [PMC free article: PMC5679066] [PubMed: 28886766]
- Pinu FR, Beale DJ, Paten AM et al. Systems biology and multi-omics integration: viewpoints from the metabolomics research community. Metabolites. 2019;9(4):76. [PMC free article: PMC6523452] [PubMed: 31003499]
- Platt J, Spector-Bagdady K, Platt T et al. Ethical, legal, and social implications of learning health systems. Learning Healthcare Systems. 2018;2(1):e10051. [PMC free article: PMC6508801] [PubMed: 31245577]
- Pol CWP, Raghoebar GM, Kerdijk W, Boven GC, Cune MS, Meijer HJA. A systematic review and meta-analysis of 3-unit fixed dental prostheses: Are the results of 2 abutment implants comparable to the results of 2 abutment teeth? Journal of Oral Rehabilitation. 2018;45(2):147–60. [PubMed: 28940725]
- Polak D, Shapira L. An update on the evidence for pathogenic mechanisms that may link periodontitis and diabetes. Journal of Clinical Periodontology. 2018;45(2):150–66. [PubMed: 29280184]
- Polverini PJ, Krebsbach PH. Research and discovery science and the future of dental education and practice. Journal of Dental Education. 2017;81(9):eS97–eS107. [PubMed: 28864810]
- Polverini PJ, Lingen MW. Expanding the research capacity of dental schools: are we there yet? Journal of Dental Research. 2017;96(1):8–9. [PubMed: 28033062]
- Polymeri A, Giannobile WV, Kaigler D. Bone marrow stromal stem cells in tissue engineering and regenerative medicine. Hormone and Metabolic Research. 2016;48(11):700–13. [PMC free article: PMC9284410] [PubMed: 27871114]
- Popejoy AB, Fullerton SM. Genomics is failing on diversity. Nature. 2016;538(7624):161–4. [PMC free article: PMC5089703] [PubMed: 27734877]
- Potempa J, Mydel P, Koziel J. The case for periodontitis in the pathogenesis of rheumatoid arthritis. Nature Reviews Rheumatology. 2017;13(10):606–20. [PubMed: 28835673]
- Powell RE, Henstenburg JM, Cooper G, Hollander JE, Rising KL. Patient perceptions of telehealth primary care video visits. Annals of Family Medicine. 2017;15(3):225–9. [PMC free article: PMC5422083] [PubMed: 28483887]
- Prakadan SM, Shalek AK, Weitz DA. Scaling by shrinking: empowering single-cell ‘omics’ with microfluidic devices. Nature Reviews Genetics. 2017;18(6):345–61. [PMC free article: PMC5495114] [PubMed: 28392571]
- Prentice DA. Adult stem cells: successful standard for regenerative medicine. Circulation Research. 2019;124(6):837–9. [PubMed: 30870122]
- Pretty IA, McGrady M, Zakian C et al. Quantitative light fluorescence (QLF) and polarized white light (PWL) assessments of dental fluorosis in an epidemiological setting. BMC Public Health. 2012;12:366. [PMC free article: PMC3490889] [PubMed: 22607363]
- Proctor C, Thiennimitr P, Chattipakorn N, Chattipakorn SC. Diet, gut microbiota and cognition. Metabolic Brain Disease. 2017;32(1):1–17. [PubMed: 27709426]
- Pronovost PJ, Mathews SC, Chute CG, Rosen A. Creating a purpose-driven learning and improving health system: The Johns Hopkins Medicine quality and safety experience. Learning Health Systems. 2017;1(1):e10018. [PMC free article: PMC6516722] [PubMed: 31245554]
- Qi Z, Barrett T, Parikh AS, Tirosh I, Puram SV. Single-cell sequencing and its applications in head and neck cancer. Oral Oncology. 2019;99:104441. [PMC free article: PMC6981283] [PubMed: 31689639]
- Raaijmakers RHL, Ripken L, Ausems CRM, Wansink DG. CRISPR/Cas applications in myotonic dystrophy: expanding opportunities. International Journal of Molecular Sciences. 2019;20(15). [PMC free article: PMC6696057] [PubMed: 31357652]
- Radiological Society of North America. Imaging Research Tools. 2021. https://www
.rsna.org /research/imaging-research-tools. Accessed August 12, 2021. - Rakic M, Galindo-Moreno P, Monje A et al. How frequent does peri-implantitis occur? A systematic review and meta-analysis. Clinical Oral Investigations. 2018;22(4):1805–16. [PubMed: 29218422]
- Randall CL, Wright CD, Chernus JM et al. A preliminary Genome-Wide Association Study of pain-related fear: implications for orofacial pain. Pain Research and Management. 2017;2017:7375468. [PMC free article: PMC5494109] [PubMed: 28701861]
- Rasines Alcaraz MG, Veitz-Keenan A, Sahrmann P, Schmidlin PR, Davis D, Iheozor-Ejiofor Z. Direct composite resin fillings versus amalgam fillings for permanent or adult posterior teeth. Cochrane Database Systematic Reviews. 2014(3):CD005620. [PubMed: 24683067]
- Regier DS, Hart TC. Genetics: the future is now with interprofessional collaboration. Dental Clinics of North America. 2016;60(4):943–9. [PubMed: 27671963]
- Remiszewski DP, Bidra AS. Implementation of a surgical safety checklist for dental implant surgeries in a prosthodontics residency program. Journal of Prosthetic Dentistry. 2019;122(4):371–5. [PubMed: 30982614]
- Resnik DB. How should engineered nanomaterials be regulated for public and environmental health? AMA Journal of Ethics. 2019;21(4):E363–9. [PubMed: 31012424]
- Reynolds JC, McKernan SC, Damiano PC, Kuthy RA. A tale of two public dental benefit programs: Iowa dentist participation in traditional Medicaid versus a Medicaid expansion program. BMC Oral Health. 2019;19(1):89. [PMC free article: PMC6534867] [PubMed: 31126338]
- Rho YJ, Namgung C, Jin BH, Lim BS, Cho BH. Longevity of direct restorations in stress-bearing posterior cavities: a retrospective study. Operative Dentistry. 2013;38(6):572–82. [PubMed: 23550914]
- Ricketts D, Kidd E, Weerheijm K, de Soet H. Hidden caries: what is it? Does it exist? Does it matter? International Dental Journal. 1997;47(5):259–65. [PubMed: 9448806]
- Rickinson AB. Co-infections, inflammation and oncogenesis: future directions for EBV research. Seminars in Cancer Biology. 2014;26:99–115. [PubMed: 24751797]
- Rigassio Radler D. Complementary and Alternative Medicine Practices and Oral and Nutritional Health. In: Touger-Decker R, Mobley C., Epstein J., eds. Nutrition and Oral Medicine, 2nd ed. New York: Humana Press; 2014:153–70.
- Rindal DB, Asche SE, Gryczynski J et al. De-implementing opioid use and implementing optimal pain management following dental extractions (DIODE): protocol for a cluster randomized trial. JMIR Research Protocols. 2021;10(4):e24342. [PMC free article: PMC8076983] [PubMed: 33843594]
- Rodriguez-Ramirez C, Nör JE. p53 and cell fate: sensitizing head and neck cancer stem cells to chemotherapy. Critical Reviews in Oncogenesis. 2018;23(3–4):173–87. [PMC free article: PMC6396309] [PubMed: 30311573]
- Roger JM. The Academic Dental Careers Fellowship Program: a pilot program to introduce dental students to academia. Journal of Dental Education. 2008;72(4):438–47. [PubMed: 18381850]
- Ronda C, Chen SP, Cabral V, Yaung SJ, Wang HH. Metagenomic engineering of the mammalian gut microbiome in situ. Nature Methods. 2019;16(2):167–70. [PMC free article: PMC6467691] [PubMed: 30643213]
- Rose EH. Aesthetic restoration of the severely disfigured face in burn victims: a comprehensive strategy. Plastic and Reconstructive Surgery. 1995;96(7):1573–85. [PubMed: 7480277]
- Rupp JK, Jones DL, Seale NS. Dental students’ knowledge about careers in academic dentistry. Journal of Dental Education. 2006;70(10):1051–60. [PubMed: 17021284]
- Saadaoui M, Singh P, Al Khodor S. Oral microbiome and pregnancy: a bidirectional relationship. Journal of Reproductive Immunology. 2021;145:103293. [PubMed: 33676065]
- Salvi A, Giacopuzzi E, Bardellini E et al. Mutation analysis by direct and whole exome sequencing in familial and sporadic tooth agenesis. International Journal of Molecular Medicine. 2016;38(5):1338–48. [PMC free article: PMC5065298] [PubMed: 27665865]
- Samaranayake LP, Peiris M. Severe acute respiratory syndrome and dentistry: a retrospective view. Journal of the American Dental Association. 2004;135(9):1292–1302. [PMC free article: PMC7093872] [PubMed: 15493394]
- Sánchez-Sanhueza G, Bello-Toledo H, González-Rocha G, Gonçalves AT, Valenzuela V, Gallardo-Escárate C. Metagenomic study of bacterial microbiota in persistent endodontic infections using Next-generation sequencing. International Endodontic Journal. 2018;51(12):1336–48. [PubMed: 29786880]
- Saranjam H, Chopra SS, Levy H et al. A germline or de novo mutation in two families with Gaucher disease: implications for recessive disorders. European Journal of Human Genetics. 2013;21(1):115–17. [PMC free article: PMC3522207] [PubMed: 22713811]
- Scheinost D, Noble S, Horien C et al. Ten simple rules for predictive modeling of individual differences in neuroimaging. Neuroimage. 2019;193:35–45. [PMC free article: PMC6521850] [PubMed: 30831310]
- Schenkein HA, Best AM. Factors considered by new faculty in their decision to choose careers in academic dentistry. Journal of Dental Education. 2001;65(9):832–40. [PubMed: 11569598]
- Scheper A. AMA policies and Code of Medical Ethics’ opinions related to human genome editing. AMA Journal of Ethics. 2019;21(12):E1056–8. [PubMed: 31876469]
- Schincaglia GP, Hong BY, Rosania A et al. Clinical, immune, and microbiome traits of gingivitis and peri-implant mucositis. Journal of Dental Research. 2017;96(1):47–55. [PubMed: 28033066]
- Schully SD, Lam TK, Dotson WD et al. Evidence synthesis and guideline development in genomic medicine: current status and future prospects. Genetics in Medicine. 2015;17(1):63–7. [PMC free article: PMC4272332] [PubMed: 24946156]
- Schwendicke F, Samek W, Krois J. Artificial Intelligence in dentistry: chances and challenges. Journal of Dental Research. 2020;99(7):769–74. [PMC free article: PMC7309354] [PubMed: 32315260]
- Secretary’s Advisory Committee for Healthy People 2030. Recommendations for Building a Data Partnership Infrastructure for Healthy People 2030. Secretary’s Advisory Committee Report #9. Washington, DC: USDHHS; 2019. https://www
.healthypeople .gov/sites/default /files/Report%209_Data %20Partnerships_2019.12.2_508.pdf. Accessed June 25, 2021. - Selby JV, Krumholz HM, Kuntz RE, Collins FS. Network news: powering clinical research. Science Translational Medicine. 2013;5(182):182fs113. [PMC free article: PMC5101932] [PubMed: 23612587]
- Serhan CN, Clish CB, Brannon J, Colgan SP, Chiang N, Gronert K. Novel functional sets of lipid-derived mediators with antiinflammatory actions generated from omega-3 fatty acids via cyclooxygenase 2-nonsteroidal antiinflammatory drugs and transcellular processing. Journal of Experimental Medicine. 2000;192(8):1197–1204. [PMC free article: PMC2195872] [PubMed: 11034610]
- Shah N, Bansal N, Logani A. Recent advances in imaging technologies in dentistry. World Journal of Radiology. 2014;6(10):794–807. [PMC free article: PMC4209425] [PubMed: 25349663]
- Shan T, Tay FR, Gu L. Application of Artificial Intelligence in dentistry. Journal of Dental Research. 2021;100(3):232–44. [PubMed: 33118431]
- Shapiro E, Biezuner T, Linnarsson S. Single-cell sequencing-based technologies will revolutionize whole-organism science. Nature Reviews Genetics. 2013;14(9):618–30. [PubMed: 23897237]
- Shaw L, Ribeiro ALR, Levine AP et al. The human salivary microbiome is shaped by shared environment rather than genetics: evidence from a large family of closely related individuals. MBio. 2017;8(5). [PMC free article: PMC5596345] [PubMed: 28900019]
- Shi W, Heber D. Nutrition and Dental Health. In: Berdanier CD, Dwyer JT, Heber D, eds. Handbook of Nutrition and Food. Boca Raton: CRC Press; 2013.
- Shi XQ, Welander U, Angmar-Mansson B. Occlusal caries detection with KaVo DIAGNOdent and radiography: an in vitro comparison. Caries Research. 2000;34(2):151–8. [PubMed: 10773633]
- Shi Y, Wang Y, Li Q et al. Immunoregulatory mechanisms of mesenchymal stem and stromal cells in inflammatory diseases. Nature Reviews Nephrology. 2018;14(8):493–507. [PubMed: 29895977]
- Shimpi N, Ashton JL, Sorenson CA et al. Interdisciplinary Care Model: Diabetes and Oral Health. In: Acharya A, Powell V, Torres-Urquidy MH, Posteraro RH, Thyvalikakath TP, eds. Integration of Medical and Dental Care and Patient Data. 2nd ed. Switzerland: Springer International Publishing; 2019d:47–61.
- Shimpi N, Dart R, Umukoro P, Acharya A. Interdisciplinary Care Model: Cardiovascular Diseases and Oral Health. In: Acharya A, Powell V, Torres-Urquidy MH, Posteraro RH, Thyvalikakath TP, eds. Integration of Medical and Dental Care and Patient Data. 2nd ed. Switzerland: Springer International Publishing; 2019b:71–85.
- Shimpi N, Glurich I, Acharya A. Integrated Care Case Study: Marshfield Clinic Health System. In: Acharya A, Powell V, Torres-Urquidy MH, Posteraro RH, Thyvalikakath TP, eds. Integration of Medical and Dental Care and Patient Data. 2nd ed. Switzerland: Springer International Publishing; 2019a:315–26.
- Shimpi N, Glurich I, Schroeder D, Katrak C, Chyou PH, Acharya A. Patient awareness of association of diabetes and periodontal disease. Health Promotion Practice. 2018:1524839918801909. [PubMed: 30238811]
- Shimpi N, Pathak R, Acharya A. Interdisciplinary Care Model: Metabolic Syndrome and Oral Health. In: Acharya A, Powell V, Torres-Urquidy M, Posteraro R, Thyvalikakath T, eds. Integration of Medical and Dental Care and Patient Data. 2nd ed. Switzerland: Springer International Publishing; 2019c:141–54.
- Shimpi N, Schroeder D, Kilsdonk J et al. Medical providers’ oral health knowledgeability, attitudes, and practice behaviors: an opportunity for interprofessional collaboration. Journal of Evidence-Based Dental Practice. 2016;16(1):19–29. [PubMed: 27132552]
- Siemionow M. The decade of face transplant outcomes. Journal of Materials Science: Materials in Medicine. 2017;28(5):64. [PubMed: 28303433]
- Sigmund O. Materials with prescribed constitutive parameters: an inverse homogenization problem. International Journal of Solids and Structures. 1994;31(17):2313–29.
- Simon JC, Lucas SA, Staninec M et al. Near-IR transillumination and reflectance imaging at 1,300 nm and 1,500-1,700 nm for in vivo caries detection. Lasers in Surgery and Medicine. 2016;48(9):828–36. [PMC free article: PMC5495016] [PubMed: 27389018]
- Slavkin HC. The impact of research on the future of dental education: how research and innovation shape dental education and the dental profession. Journal of Dental Education. 2017;81(9):eS108–27. [PubMed: 28864811]
- Slavkin HC. From high definition precision healthcare to precision public oral health: opportunities and challenges. Journal of Public Health Dentistry. 2018;80:S23–30. [PubMed: 30516837]
- Slots J. Periodontal herpesviruses: prevalence, pathogenicity, systemic risk. Periodontology 2000. 2015;69(1):28–45. [PubMed: 26252400]
- Smith CEL, Poulter JA, Brookes SJ et al. Phenotype and variant spectrum in the LAMB3 form of Amelogenesis Imperfecta. Journal of Dental Research. 2019;98(6):698–704. [PMC free article: PMC6535922] [PubMed: 30905256]
- Smits SA, Marcobal A, Higginbottom S, Sonnenburg JL, Kashyap PC. Individualized responses of gut microbiota to dietary intervention modeled in humanized mice. mSystems. 2016;1(5). [PMC free article: PMC5069738] [PubMed: 27822551]
- Snyder J, Rin Son A, Hamid Q, Wang C, Lui Y, Sun W. Mesenchymal stem cell printing and process regulated cell properties. Biofabrication. 2015;7(4):044106. [PubMed: 26696405]
- Song M, Liu K, Abromitis R, Schleyer TL. Reusing electronic patient data for dental clinical research: a review of current status. Journal of Dentistry. 2013;41(12):1148–63. [PMC free article: PMC4141471] [PubMed: 23603087]
- Song M, Spallek H, Polk D, Schleyer T, Wali T. How information systems should support the information needs of general dentists in clinical settings: suggestions from a qualitative study. BMC Medical Informatics and Decision Making. 2010;10(1):7–15. [PMC free article: PMC2843644] [PubMed: 20122272]
- Song PH, McAlearney AS, Robbins J, McCullough JS. Exploring the business case for ambulatory electronic health record system adoption. Journal of Healthcare Management. 2011;56(3):169–82. [PubMed: 21714372]
- Speicher DJ, Ramirez-Amador V, Dittmer DP, Webster-Cyriaque J, Goodman MT, Moscicki AB. Viral infections associated with oral cancers and diseases in the context of HIV: a workshop report. Oral Diseases. 2016;22:181–92. [PMC free article: PMC5590239] [PubMed: 27109286]
- Stacy A, McNally L, Darch SE, Brown SP, Whiteley M. The biogeography of polymicrobial infection. Nature Reviews Microbiology. 2016;14(2):93–105. [PMC free article: PMC5116812] [PubMed: 26714431]
- Stark Z, Dolman L, Manolio TA et al. Integrating genomics into healthcare: a global responsibility. American Journal of Human Genetics. 2019;104(1):13–20. [PMC free article: PMC6323624] [PubMed: 30609404]
- Starr JR, Huang Y, Lee KH et al. Oral microbiota in youth with perinatally acquired HIV infection. Microbiome. 2018;6(1):100. [PMC free article: PMC5984365] [PubMed: 29855347]
- Stewart CA, Finer Y. Biostable, antidegradative and antimicrobial restorative systems based on host-biomaterials and microbial interactions. Dental Materials. 2019;35(1):36–52. [PMC free article: PMC6312743] [PubMed: 30301624]
- Stewart MP, Sharei A, Ding X, Sahay G, Langer R, Jensen KF. In vitro and ex vivo strategies for intracellular delivery. Nature. 2016;538(7624):183–92. [PubMed: 27734871]
- Stirman SW, Miller CJ, Toder K, Calloway A. Development of a framework and coding system for modifications and adaptations of evidence-based interventions. Implementation Science. 2013;8:65. [PMC free article: PMC3686699] [PubMed: 23758995]
- Stone VN, Xu P. Targeted antimicrobial therapy in the microbiome era. Molecular Oral Microbiology. 2017;32(6):446–54. [PMC free article: PMC5697594] [PubMed: 28609586]
- Stookey GK. Quantitative light fluorescence: a technology for early monitoring of the caries process. Dental Clinics of North America. 2005;49(4):753–70. [PubMed: 16150315]
- Stucky A, Sedghizadeh PP, Mahabady S et al. Single-cell genomic analysis of head and neck squamous cell carcinoma. Oncotarget. 2017;8(42):73208–18. [PMC free article: PMC5641207] [PubMed: 29069864]
- Stulberg E, Fravel D, Proctor LM et al. An assessment of U.S. microbiome research. Nature Microbiology. 2016;1:15015. [PubMed: 27571759]
- Susarla SM, Swanson E, Gordon CR. Craniomaxillofacial reconstruction using allotransplantation and tissue engineering: challenges, opportunities, and potential synergy. Annals of Plastic Surgery. 2011;67(6):655–61. [PubMed: 21825966]
- Swanson KV, Deng M, Ting JP. The NLRP3 inflammasome: molecular activation and regulation to therapeutics. Nature Reviews Immunology. 2019;19(8):477–89. [PMC free article: PMC7807242] [PubMed: 31036962]
- Tachjian A, Maria V, Jahangir A. Use of herbal products and potential interactions in patients with cardiovascular diseases. Journal of the American College of Cardiology. 2010;55(6):515–25. [PMC free article: PMC2831618] [PubMed: 20152556]
- Taha AA, Patel MP, Hill RG, Fleming PS. The effect of bioactive glasses on enamel remineralization: a systematic review. Journal of Dentistry. 2017;67:9–17. [PubMed: 28939485]
- Takahashi N. Oral microbiome metabolism: from “Who Are They?” to “What Are They Doing?” Journal of Dental Research. 2015;94(12):1628–37. [PubMed: 26377570]
- Tappa K, Jammalamadaka U. Novel biomaterials used in medical 3D printing techniques. Journal of Functional Biomaterials. 2018;9(1):17. [PMC free article: PMC5872103] [PubMed: 29414913]
- Tate JR, Tollefson TT. Advances in facial reanimation. Current Opinion in Otolaryngology & Head & Neck Surgery. 2006;14(4):242–8. [PubMed: 16832180]
- Tavelli L, McGuire MK, Zucchelli G et al. Extracellular matrix-based scaffolding technologies for periodontal and peri-implant soft tissue regeneration. Journal of Periodontology. 2020;91(1):17–25. [PubMed: 31475361]
- Teijido O, Cacabelos R. Pharmacoepigenomic interventions as novel potential treatments for Alzheimer’s and Parkinson’s diseases. International Journal of Molecular Sciences. 2018;19(10):3199. [PMC free article: PMC6213964] [PubMed: 30332838]
- Ten Bosch JJ, Angmar-Mansson B. A review of quantitative methods for studies of mineral content of intra-oral caries lesions. Journal of Dental Research. 1991;70(1):2–14. [PubMed: 1991857]
- The Learning Healthcare Project. What is a Learning Health System? 2021. https:
//learninghealthcareproject .org/introduction-and-rationale /what-is-a-learning-health-system/. Accessed June 25, 2021. - To KK-W, Tsang OT-Y, Leung W-S et al. Temporal profiles of viral load in posterior oropharyngeal saliva samples and serum antibody responses during infection by SARS-CoV-2: an observational cohort study. The Lancet Infectious Diseases. 2020;20(5):565–74. [PMC free article: PMC7158907] [PubMed: 32213337]
- Tonetti MS, Sanz M. Implementation of the new classification of periodontal diseases: Decision-making algorithms for clinical practice and education. Journal of Clinical Periodontology. 2019;46(4):398–405. [PubMed: 30883878]
- Tyler-Smith C, Yang H, Landweber LF et al. Where next for genetics and genomics? PLoS Biology. 2015;13(7):e1002216. [PMC free article: PMC4520474] [PubMed: 26225775]
- U.S. Food and Drug Administration. FDA Issues Recommendations for Certain High-Risk Groups Regarding Mercury-Containing Dental Amalgam. 2020a (September 24). https://www
.fda.gov/news-events /press-announcements /fda-issues-recommendations-certain-high-risk-groups-regarding-mercury-containing-dental-amalgam. Accessed June 25, 2021. - U.S. Food and Drug Administration. Consumer Alert on Regenerative Medicine Products Including Stem Cells and Exosomes. 2020b. https://www
.fda.gov/vaccines-blood-biologics /consumers-biologics /consumer-alert-regenerative-medicine-products-including-stem-cells-and-exosomes. Accessed June 25, 2021. - U.S. Food and Drug Administration. 21st Century Cures Act. 2020c. https://www
.fda.gov/regulatory-information /selected-amendments-fdc-act /21st-century-cures-act. Accessed June 25, 2021. - van de Sande FH, Opdam NJ, Rodolpho PA, Correa MB, Demarco FF, Cenci MS. Patient risk factors’ influence on survival of posterior composites. Journal of Dental Research. 2013;92(7 Suppl):78–83S. [PubMed: 23690354]
- van Dijken JW. A prospective 8-year evaluation of a mild two-step self-etching adhesive and a heavily filled two-step etch-and-rinse system in non-carious cervical lesions. Dental Materials. 2010;26(9):940–6. [PubMed: 20646753]
- Van Dyke TE, Kornman KS. Inflammation and factors that may regulate inflammatory response. Journal of Periodontology. 2008;79(8 Suppl):1503–7. [PubMed: 18673003]
- van Noort R. The future of dental devices is digital. Dental Materials. 2012;28(1):3–12. [PubMed: 22119539]
- Vaquette C, Pilipchuk SP, Bartold PM, Hutmacher DW, Giannobile WV, Ivanovski S. Tissue engineered constructs for periodontal regeneration: current status and future perspectives. Advanced Healthcare Materials. 2018;7(21):e1800457. [PubMed: 30146758]
- Vartoukian SR. Cultivation strategies for growth of uncultivated bacteria. Journal of Oral Biosciences. 2016;58(4):142–9. [PMC free article: PMC5382963] [PubMed: 28392745]
- Veloso SRM, Lemos CAA, de Moraes SLD, do Egito Vasconcelos BC, Pellizzer EP, de Melo Monteiro GQ. Clinical performance of bulk-fill and conventional resin composite restorations in posterior teeth: a systematic review and meta-analysis. Clinical Oral Investigations. 2019;23(1):221–33. [PubMed: 29594349]
- Verma A, Bang L, Miller JE et al. Human-disease phenotype map derived from PheWAS across 38,682 individuals. American Journal of Human Genetics. 2019;104(1):55–64. [PMC free article: PMC6323551] [PubMed: 30598166]
- Verma D, Garg PK, Dubey AK. Insights into the human oral microbiome. Archives of Microbiology. 2018;200(4):525–40. [PubMed: 29572583]
- Voigt A, Bohn K, Sukumaran S, Stewart CM, Bhattacharya I, Nguyen CQ. Unique glandular ex-vivo Th1 and Th17 receptor motifs in Sjögren’s syndrome patients using single-cell analysis. Clinical Immunology. 2018;192:58–67. [PMC free article: PMC6941595] [PubMed: 29679709]
- Wade VA, Taylor AD, Kidd MR, Carati C. Transitioning a home telehealth project into a sustainable, large-scale service: a qualitative study. BMC Health Services Research. 2016;16:183. [PMC free article: PMC4869378] [PubMed: 27185041]
- Wade WG. The oral microbiome in health and disease. Pharmacological Research. 2013;69(1):137–43. [PubMed: 23201354]
- Wanchek T, Cook B, Slapar F, Valachovic R. Dental schools vacant budgeted faculty positions, academic year 2014-15. Journal of Dental Education. 2016;80(8):1012–22.
- Wanchek T, Cook BJ, Anderson EL, Duranleau L, Valachovic RW. Dental school vacant budgeted faculty positions, academic years 2011–12 through 2013–14. Journal of Dental Education. 2015;79(10):1230–42. [PubMed: 26702464]
- Wang D, Bodovitz S. Single cell analysis: the new frontier in ‘omics’. Trends in Biotechnology. 2010;28(6):281–90. [PMC free article: PMC2876223] [PubMed: 20434785]
- Wang K, Huang Y, Zhang Z et al. A preliminary study of microbiota diversity in saliva and bronchoalveolar lavage fluid from patients with primary bronchogenic carcinoma. Medical Science Monitor. 2019;25:2819–34. [PMC free article: PMC6482867] [PubMed: 30994108]
- Wang P, Aguirre A. New strategies and in vivo monitoring methods for stem cell-based anticancer therapies. Stem Cells International. 2018;2018:7315218. [PMC free article: PMC6276456] [PubMed: 30581474]
- Wang X, Shaffer JR, Zeng Z et al. Genome-wide association scan of dental caries in the permanent dentition. BMC Oral Health. 2012;12:57. [PMC free article: PMC3574042] [PubMed: 23259602]
- Wang Y, Navin NE. Advances and applications of single-cell sequencing technologies. Molecular Cell. 2015;58(4):598–609. [PMC free article: PMC4441954] [PubMed: 26000845]
- Webster TJ. Safety of Nanoparticles: From Manufacturing to Medical Applications. New York: Springer; 2009.
- Wei F, Strom CM, Cheng J et al. Electric field-induced release and measurement liquid biopsy for noninvasive early lung cancer assessment. Journal of Molecular Diagnostics. 2018;20(6):738–42. [PMC free article: PMC6198245] [PubMed: 30309763]
- Weintraub JA, Birken SA, Burgette JM, Lewis TA, White BA. Use of the consolidated framework for implementation research to assess determinants of silver diamine fluoride implementation in safety net dental clinics. Journal of Public Health Dentistry. 2019;78(4):298–306. [PubMed: 31144330]
- Weintraub JA, Quinonez RB, Smith AJT et al. Responding to a pandemic: development of the Carolina Dentistry Virtual Oral Health Care Helpline. Journal of the American Dental Association. 2020;151(11):825–34. [PubMed: 33121604]
- Weitzel KW, Alexander M, Bernhardt BA et al. The IGNITE network: a model for genomic medicine implementation and research. BMC Medical Genomics. 2016;9:1. [PMC free article: PMC4700677] [PubMed: 26729011]
- Whelan R, Garavan H. When optimism hurts: inflated predictions in psychiatric neuroimaging. Biological Psychiatry. 2014;75(9):746–8. [PubMed: 23778288]
- White JD, Indencleef K, Naqvi S et al. Insights into the genetic architecture of the human face. Nature Genetics. 2021;53(1):45–53. [PMC free article: PMC7796995] [PubMed: 33288918]
- White JM, Kalenderian E, Stark PC, Ramoni RL, Vaderhobli R, Walji MF. Evaluating a dental diagnostic terminology in an electronic health record. Journal of Dental Education. 2011;75(5):605–15. [PMC free article: PMC3119719] [PubMed: 21546594]
- Williams RC, Paquette DW, Offenbacher S et al. Treatment of periodontitis by local administration of minocycline microspheres: a controlled trial. Journal of Periodontology. 2001;72(11):1535–1544. [PubMed: 11759865]
- Working Group on Diversity in the Biomedical Research Workforce. Draft Report of the Advisory Committee to the Director Working Group on Diversity in the Biomedical Research Workforce. Bethesda, MD: National Institutes of Health; 2012.
- World Health Organization. Human Genomics in Global Health: Genes and Human Diseases. 2020. https://www
.who.int/genomics /public/geneticdiseases /en/index2.html. Accessed June 25, 2021. - Wu CH, Wang CC, Tsai MT, Huang WT, Kennedy J. Trend and pattern of herb and supplement use in the United States: results from the 2002, 2007, and 2012 national health interview surveys. Evidence-Based Complementary and Alternative Medicine. 2014;2014:872320. [PMC free article: PMC4276694] [PubMed: 25574184]
- Wu Z, Wang F, Fan Z et al. Whole tooth regeneration by allogeneic cell reassociation in pig jawbone. Tissue Engineering Part A. 2019;25(17–18):1202–12. [PubMed: 30648470]
- Wunsch K, Wurst R, von Dawans B, Strahler J, Kasten N, Fuchs R. Habitual and acute exercise effects on salivary biomarkers in response to psychosocial stress. Psychoneuroendocrinology. 2019;106:216–25. [PubMed: 31003138]
- Xiao J, Huang X, Alkhers N et al. Candida albicans and early childhood caries: a systematic review and meta-analysis. Caries Research. 2018;52(1–2):102–12. [PMC free article: PMC5828948] [PubMed: 29262404]
- Yan X, Yang M, Liu J et al. Discovery and validation of potential bacterial biomarkers for lung cancer. American Journal of Cancer Research. 2015;5(10):3111–22. [PMC free article: PMC4656734] [PubMed: 26693063]
- Yang VB, Curtis DA, Fried D. Cross-polarization reflectance imaging of root caries and dental calculus on extracted teeth at wavelengths from 400 to 2350 nm. Journal of Biophotonics. 2018;11(11):e201800113. [PubMed: 29952066]
- Yarkoni T, Westfall J. Choosing prediction over explanation in psychology: lessons from machine learning. Perspectives on Psychological Science. 2017;12(6):1100–22. [PMC free article: PMC6603289] [PubMed: 28841086]
- Yelick PC, Vacanti JP. Bioengineered teeth from tooth bud cells. Dental Clinics of North America. 2006;50(2):191–203. [PubMed: 16530057]
- Yim N, Ryu SW, Choi K et al. Exosome engineering for efficient intracellular delivery of soluble proteins using optically reversible protein-protein interaction module. Nature Communications. 2016;7:12277. [PMC free article: PMC4961865] [PubMed: 27447450]
- Yong R, Ranjitkar S, Townsend GC et al. Dental phenomics: advancing genotype to phenotype correlations in craniofacial research. Australian Dental Journal. 2014;59:34–47. [PubMed: 24611797]
- Yost S, Duran-Pinedo AE, Teles R, Krishnan K, Frias-Lopez J. Functional signatures of oral dysbiosis during periodontitis progression revealed by microbial metatranscriptome analysis. Genome Medicine. 2015;7(1):27. [PMC free article: PMC4410737] [PubMed: 25918553]
- Yu N, Yang J, Mishina Y, Giannobile WV. Genome editing: a new horizon for oral and craniofacial research. Journal of Dental Research. 2019;98(1):36–45. [PMC free article: PMC6728561] [PubMed: 30354846]
- Zhang K, Zhang N, Weir MD, Reynolds MA, Bai Y, Xu HHK. Bioactive dental composites and bonding agents having remineralizing and antibacterial characteristics. Dental Clinics of North America. 2017;61(4):669–87. [PMC free article: PMC5803788] [PubMed: 28886763]
- Zhang S, Divaris K, Moss K al. The Novel ASIC2 Locus is associated with severe gingival inflammation. JDR Clinical & Translational Research. 2016;1(2):163–70. [PMC free article: PMC5409514] [PubMed: 28459102]
- Zhang Y, Kelly JR. Dental ceramics for restoration and metal veneering. Dental Clinics of North America. 2017;61(4):797–819. [PMC free article: PMC5657342] [PubMed: 28886769]
- Zhang Y, Lawn BR. Novel zirconia materials in dentistry. Journal of Dental Research. 2018;97(2):140–7. [PMC free article: PMC5784474] [PubMed: 29035694]
- Zhao H, Chu M, Huang Z et al. Variations in oral microbiota associated with oral cancer. Scientific Reports. 2017;7(1):11773. [PMC free article: PMC5603520] [PubMed: 28924229]
- Zhu F, Zhang H, Wu H. Glycosyltransferase-mediated sweet modification in oral Streptococci. Journal of Dental Research. 2015;94(5):659–65. [PMC free article: PMC4502785] [PubMed: 25755271]
- Zhu Y, Close K, Zeldin L, Quinonez RB, White BA, Rozier RG. A clinical vignette-based study of physicians’ adherence to guidelines for dental referrals of young children. Academic Pediatrics. 2019a;19(2):195–202. [PubMed: 30361125]
- Zhu Y, Close K, Zeldin LP, White BA, Rozier RG. Implementation of oral health screening and referral guidelines in primary health care. JDR Clinical & Translational Research. 2019b;4(2):167–77. [PubMed: 30931710]
- Zozus MN, Hammond E, Green B, Kahn M, Richesson R, Rusincovitch S, Simon G, Smerek M. Assessing Data Quality for Healthcare Systems Data Used in Clinical Research. NIH Collaboratory. Bethesda, MD; 2014.
- Emerging Science and Promising Technologies to Transform Oral Health - Oral Heal...Emerging Science and Promising Technologies to Transform Oral Health - Oral Health in America
Your browsing activity is empty.
Activity recording is turned off.
See more...