NCBI Bookshelf. A service of the National Library of Medicine, National Institutes of Health.
Nussey S, Whitehead S. Endocrinology: An Integrated Approach. Oxford: BIOS Scientific Publishers; 2001.
Chapter objectives
Knowledge of
- 1.
Importance of iodine in thyroid metabolism
- 2.
Mechanisms of iodination of tyrosine and their regulation
- 3.
Physiological roles of thyroxine and tri-iodothyronine, their transport and metabolism
- 4.
Physiological control of thyroid growth and hormone secretion
- 5.
Causes and clinical effects of insufficient and excessive thyroid hormone secretion
- 6.
Investigation and treatment of thyroid disease
‘See, see! what showers arise,
Blown with the windy tempest of my heart.’
King Henry VI Part III, William Shakespeare.
Thyroid hormones are extremely important and have diverse actions. They act on virtually every cell in the body to alter gene transcription: under- or over-production of these hormones has potent effects. Disorders associated with altered thyroid hormone secretion are common and affect about 5% women and 0.5% men.
Like the catecholamines epinephrine and norepinephrine, thyroid hormones are synthesized from the amino acid tyrosine. The synthesis of thyroid hormones requires the iodination of tyrosine molecules and the combination of two iodinated tyrosine residues (Box 3.1). Whilst tyrosine is relatively easily iodinated, iodine is rare, ranking 61st in the list of most common elements and forming just 0.000006% of the Earth's mantle. The thyroid gland has evolved not only to trap this element avidly from dietary sources but also to maintain a large store of the iodinated tyrosines to maintain the secretion of thyroid hormones during periods of relative iodine deficiency. Clinical Case 3.1 illustrates the importance of iodine in thyroid function and, whilst it is relatively unusual, it demonstrates the problems of excess iodine intake and a consequent increase in thyroid hormone synthesis and release.

Box 3.1
Structures of hormones derived from tyrosine.
Clinical Case 3.1
A 25-year-old city high-flyer was in an interview for a position with a merchant bank. During the interview she suddenly found herself unable to speak and left the room unsteadily and in tears under the gaze of her disbelieving interviewers. The sympathetic secretary in the outer office ordered her a taxi to the hospital. She was found to have an irregular pulse rate of 180 beats per minute and remained unable to find her words (termed an expressive dysphasia). By writing, she was able to inform the medical team that she was taking the drug amiodarone for a heart condition that runs in her father's family. She had attributed recent anxiety, poor sleeping patterns, intolerance of summer weather and a 3 kg weight loss to the worry over the job interview. An electrocardiogram confirmed the irregular tachycardia and a diagnosis of a left cerebral hemisphere stroke (causing the expressive dysphasia), secondary to atrial fibrillation, was made. The atrial fibrillation was treated. Clinical examination revealed a diffusely enlarged thyroid gland (Box 3.4) and blood tests later confirmed that she was hyperthyroid.

Box 3.4
Gross anatomy and histology of a normal thyroid gland. WHO classification of a goitre. Gross anatomy Histological appearance of normal thyroid follicles
The important clue to Clinical Case 3.1, which led to her diagnosis, was the drug she had been prescribed to treat her inherited cardiac condition. Amiodarone (Box 3.2) contains 30% by weight of iodine and, at the dose prescribed (one 200 mg tablet daily), she was ingesting approximately 70 mg iodine per day.

Box 3.2
Structure of amiodarone.
Iodine intake
The normal European daily dietary intake of iodine is about 150 μg, of which approximately 125 μg is taken up by the thyroid gland and used for hormone synthesis. The patient in Clinical Case 3.1 was, thus, taking over 500 times the normal intake (released upon the breakdown of amiodarone) resulting in an excess production and release of thyroid hormones and symptoms of hyperthyroidism. In contrast, lack of iodine in the diet leads to hypothyroidism.
Iodine (as the iodide, I-) is relatively abundant in seawater and seafood is a rich dietary source. Fruit and vegetables also contain significant concentrations of iodine, although the amount depends on the soil and growing region. Areas of iodine deficiency tend to be inland, at high altitude and isolated and daily iodine intake may be as low as 25 μg. The term cretinism, used to define the severe impairment of physical and neurological development resulting from iodine deficiency during fetal and post-natal development, derived from ‘cretein’, a term first used in the Swiss Alps. The word cretin entered the vernacular as a term of abuse indicating severe mental retardation. In 1994, nearly 30% of the world population was at risk of iodine deficiency. The commitment from the World Health Organization in 1990 to eliminate iodine deficiency disorders by the year 2000 (with a policy of iodide supplementation of salt) had reduced the population at risk to less than 15% by 1997.
Increased iodine intake or a deficiency of dietary iodine can both induce goiter formation (Box 3.4). The former results from increased thyroid hormone synthesis and storage as seen in Clinical Case 3.1. Goiter formation in regions of dietary deficiency (the most common cause of goiter), is graphically illustrated in Box 3.3. It is due to reduced thyroid hormone synthesis and the compensatory increased secretion of the pituitary hormone thyrotrophin as a result of the loss of negative feedback (see below).

Box 3.3
Multinodular goiter due to a dietary deficiency of iodine in Kashmir. In the mountains of Kashmir patients such as this are said to find it easy going up mountains, but much more difficult negotiating an accurate descent.
Anatomical features of the thyroid gland
The thyroid gland consists of two lobes lying on either side of the ventral aspect of the trachea. Each lobe is about 4 cm in length and 2 cm thickness connected together by a thin band of connective tissue called the isthmus (Box 3.4). Weighing approximately 20 g, it is one of the largest classical endocrine glands in the body and receives a high blood flow from the superior thyroid arteries (arising from the external carotids) and the inferior thyroid arteries (arising from the subclavian arteries). The gland is so important that it takes more blood per unit weight than the kidney and sometimes, when there is a goiter, blood flow in the gland may be heard with a stethoscope. The sound is termed a bruit.
The functional unit of the thyroid gland is the follicle, a roughly spherical group of cells arranged around a protein-rich storage material called colloid. The follicular cells are orientated with their bases near the capillary blood supply and the apices abutting the colloid.
Iodine trapping and thyroid function
The active uptake of iodide (I-) by the follicular cells involves an energy-requiring (ATPase-dependent) transport mechanism which allows I- to be taken up from capillary blood against both a concentration and an electrical gradient in exchange for Na+ (Box 3.5). This enables the thyroid gland to concentrate iodide 30–50 times that of the circulating concentration and allows radioactive isotopes to be used to investigate patients with thyroid disease (Box 3.6). Other ions such as bromide, chlorate or pertechnetate (though not fluoride) may also compete with I- for this uptake process and this has important clinical uses (see Box 3.14). On a normal daily iodide intake the thyroid gland clears approximately 20 ml of plasma iodide per minute.

Box 3.5
Uptake and organification of iodine by the thyroid gland. Active uptake of iodide (I-) in exchange for Na+.
Iodide may be discharged from the follicular cell by administration of competing ions such as perchlorate, bromide or chlorate.

Box 3.6
Radioisotopic scanning of the thyroid gland. Uptake of a radioactive isotope by the thyroid gland is used to determine: localization of the gland

Box 3.14
Effect of pregnancy on thyroid gland physiology. Increased renal I- clearance (increasing dietary requirements in deficient areas). Increased serum TBG increasing total T4 and T3 concentrations (see Box 3.28).
The active uptake of iodide appears to be the main control point for hormone synthesis and is stimulated by the pituitary hormone thyrotrophin (thyroid stimulating hormone, TSH). Iodide itself, however, plays an important role in regulating the activity of the thyroid gland (termed autoregulation). Excess iodine given to a person with normal thyroid gland activity leads to an initial reduction in organification and hormone synthesis and secretion, the Wolff-Chaikoff effect. ‘Escape’ from this inhibition (as was seen in Clinical Case 3.1) generally occurs after several days. The exact mechanism(s) of such autoregulation is unknown but the Wolff-Chaikoff effect is useful clinically because pharmacological doses of iodine may be used to reduce acutely the activity of the thyroid gland (see page 19). When dietary intake of iodine is insufficient, overactivity of the thyroid gland is a more common initial response to excess iodine.
Synthesis of thyroid hormones
The synthesis and storage of thyroid hormones occurs between the follicular cells and the colloid (Box 3.5). Different follicles may be in different states of activity. Less active follicles contain cells with a more cuboidal appearance, whilst the active follicles contain columnar cells.
The process of thyroid hormone synthesis is complex. Once inside the follicular cell, iodide is oxidized to active iodine by hydrogen peroxide. This reaction is catalyzed by the heme-containing enzyme thyroid peroxidase (TPO). Iodine is then actively transported across the apical surface of the follicular cell by the same active process that occurs at the basal surface.
At the apical-colloid interface, iodine is immediately incorporated into the tyrosine residues of the large glycoprotein thyroglobulin molecules. Thyroglobulin is synthesized in the follicular cells and has a molecular weight of around 650 000 with about 140 tyrosine residues, depending on the form of thyroglobulin. Approximately one quarter of these residues can be iodinated. Once iodinated, thyroglobulin is taken up into the colloid of the follicle where, still incorporated in the protein, a coupling reaction between pairs of iodinated tyrosine molecules occurs. The coupling of two tyrosine residues each iodinated at two positions (di-iodotyrosine, DIT) produces tetra-iodothyronine or thyroxine (T4) whilst the combination of DIT with mono-iodotyrosine (MIT) produces tri-iodothyronine (T3). Such coupling can occur within a single molecule of thyroglobulin or between dimerized molecules of the protein. This coupling is catalyzed by TPO.
Thyroid hormones are stored in this state and are only released when the thyroglobulin molecule is taken back up into the follicular cells (Box 3.7). Stimulated by TSH, thyroglobulin droplets are captured by the follicular cells by a process of pinocytosis. Fusion of the droplets with lysosomes results in hydrolysis of the thyroglobulin molecules and release of T4 and T3. About 10% of T4 undergoes mono-deiodination to T3 before it is secreted and the released iodine is recycled.
Approximately 100 μg of thyroid hormones are secreted from the gland each day, mostly in the form of T4 with about 10% as T3. Eighty percent of the T4 undergoes peripheral conversion to the more active T3 in the liver and kidney (T3 is ten times more active than T4) or to reverse T3 (rT3) that has little or no biological activity (Box 3.1). Very small quantities of other iodinated molecules, such as MIT and DIT as well as thyroglobulin, are also measurable in the circulation. As this thyroglobulin originates from the normal secretory process, its measurement in the serum is used, for example, to detect endogenous thyroid secretion when patients are taking oral T4 replacement (an important clinical use).
Actions of thyroid hormones
The effects of thyroid hormones on virtually every cell in the body is manifest in the widespread clinical effects of their lack or excess (Box 3.8). They are very important in growth and development and their role in these processes will be discussed in relation to Clinical Case 3.3 (see page 94).

Box 3.8
Clinical manifestations of thyroid hormone deficiency and excess.
Many of the actions of thyroid hormones are mediated by their binding to nuclear receptors (Box 3.9) that have a preferential affinity for T3. T3 receptors are, like all the steroid hormone receptors, members of a family of nuclear transcription factors that, in combination with other transcription factors, regulate gene expression in target cells. Unlike some steroid receptors (i.e. those for sex steroids and glucocorticoids), thyroid hormone receptors exist in the nucleus, not the cytoplasm, and may remain bound to DNA in the absence of hormone binding.
Thyroid hormones are lipid soluble and readily cross cell membranes. Once inside the nucleus, T3 binds to its receptor. This dimerizes with another T3 receptor (to form a homodimer) or with a different receptor, notably the retinoic acid receptor, to form a heterodimer (Box 3.9). In this form, the dimers interact with DNA. This occurs between recognition sites in the ‘zinc fingers’ of the DNA-binding domains of the receptors and particular base sequences in the DNA helix known as hormone response elements (HRE). The location of HREs determines which genes are regulated by T3.
There is also evidence that thyroid hormones can have rapid, non-genomic effects on membrane receptors independent of protein synthesis. These include stimulation of sugar transport, Ca2+ATPase activity and increased Na+ transport in muscle. The receptors for these effects have not been identified.
In most tissues (exceptions include brain, spleen and testis), thyroid hormones stimulate the metabolic rate by increasing the number and size of mitochondria, stimulating the synthesis of enzymes in the respiratory chain and increasing membrane Na+-K+ ATPase concentration and membrane Na+ and K+ permeability. Since as much as 15–40% of a cell's resting energy expenditure is used to maintain its electrochemical gradient (pumping Na+ out in exchange for K+), increasing the Na+-K+ ATPase activity, therefore, increases the resting metabolic rate (RMR). RMR may increase by up to 100% in the presence of excess hormones or decrease by as much as 50% in a deficiency.
The clinical signs of intolerance to heat, weight loss and fatigue, as seen in Clinical Case 3.1, are typical symptoms of patients presenting with hyperthyroidism (Box 3.10) and provide evidence of the metabolic action of thyroid hormones on their target tissues. In contrast, the hypothyroid patient is intolerant to cold, is lethargic, gains weight and has a cool, dry skin.

Box 3.10
Clinical features of excess thyroid hormone.
Whilst these metabolic changes associated with hyperthyroidism increase cardiac output, the increase is disproportionate to the increase in metabolic rate. The reason for this is that thyroid hormones have positive inotropic and chronotropic effects on the heart. The chronotropic effects of thyroid hormone (increases in sinoatrial node firing rate, decreases in atrial excitation threshold, decreased refractory period of conduction tissues) may be due to increases in sarcolemmal Na+ transport and Ca2+ influx. The inotropic effects (increase in contractile force) may be mediated by increases in Ca2+-ATPase activity in sarcoplasmic reticulum and increases in the expression of the α-myosin heavy chain.
Thus, it is reasonable to assume that it was these effects of excess thyroid hormones on the cardiovascular system that led to the onset of atrial fibrillation in Clinical Case 3.1. This led to the disastrous formation of atrial thrombus with subsequent embolization to the left cerebral hemisphere producing a stroke and the consequent temporary loss of speech.
Control of thyroid hormone synthesis and secretion
Like the adrenal cortex and the gonads, the thyroid gland is controlled by hormone secretions from the hypothalamo-pituitary axis. The synthesis and secretion of TSH from the thyrotrophs is stimulated by the tripeptide, thyrotrophin-releasing hormone (TRH). This small peptide, cleaved from a larger pro-hormone, is released from neurosecretory cells in the hypothalamus into the hypothalamo-hypophyseal portal capillaries where it is transported to the pituitary thyrotrophs (Box 3.11). TSH secretion is inhibited by other hormones (including somatostatin and dopamine) and also cytokines, particularly IL-1β, IL-6 and TNF-α.

Box 3.11
Control of the thyroid gland activity.
TSH is a complex glycoprotein hormone, containing approximately 16% carbohydrate. It contains 211 amino acids in two sub-units and has a molecular weight of about 28 000–30 000. The α unit is identical to that of two other glycoprotein hormones secreted by the human anterior pituitary gland, luteinizing hormone (LH) and follicle stimulating hormone (FSH, see Box 6.13). The β unit is unique to TSH and confers biological specificity. The structural homology between TSH, LH and FSH includes ‘knots’ of three disulfide bonds in both α and β sub-units. The glycosylation of TSH is heterogeneous and this affects both its bioactivity and clearance. TSH has a t1/2 in the circulation of about 1 h.
The cell surface receptor for TSH is a typical G-protein linked receptor with 7 helical transmembrane domains, 3 external (E) loops and three internal (I) loops (Box 3.12). The hormone binds to the long extracellular amino terminus whilst the carboxyl terminus is intracellular. There are approximately 1 000 TSH receptors on the basal surface of each follicular cell.
The binding of TSH to its receptor activates the G proteins associated with the I loops. This involves the replacement of GDP bound to the αβγ G-protein heterotrimer with GTP. The α sub-unit then dissociates from the complex and activates intracellular enzymes. The G proteins coupled to the TSH receptor, Gs and Gq, stimulate adenylate cyclase and phospholipase C, respectively. Adenylate cyclase activates protein kinase A whilst phospholipase C stimulates the hydrolysis of phosphoinositides to inositol trisphosphate (IP3) and diacylglycerol. IP3 transiently increases the concentration of intracellular Ca2+ whilst diacylglycerol activates protein kinase C (Box 3.12). These kinases activate further signal transduction mechanisms that eventually stimulate several processes involved in thyroid hormone synthesis and release and thyroid growth. These include iodine uptake, production of hydrogen peroxide and TPO and uptake of colloid droplets.
Inactivating mutations in the long extracellular amino-terminal of this receptor have been described and lead to hypothyroidism. In contrast, activating mutations have been described in E1 and E2 and I3 and the 2nd, 3rd, 6th and 7th transmembrane domains, though most are around the 6th and 7th domains. Activating mutations have been reported most frequently in autonomous ‘hot’ nodules (areas of increased growth and activity) of the gland. If present in the germ line and thus heritable (which is extremely rare), such activating mutations cause familial hyperthyroidism. Mutations of Gs (termed gsp) that result in constitutive activation of the G protein have also been described in ‘hot’ nodules.
The concentration of thyroid hormones in the circulation is regulated by an homeostatic feedback loop involving the hypothalamo-pituitary axis (Box 3.11). The main effect of thyroid hormones is to reduce the response of the pituitary thyrotrophs to TRH rather than altering the secretion rate of TRH from the hypothalamus. The sensitivity of the thyrotrophs to TRH depends on their intracellular concentration of T3, 80% of which is derived from the intrapituitary conversion of T4 to T3 (see Box 3.29). When circulating concentrations of T4 are low, there is an increase in the number of TRH receptors and in TSH synthesis resulting in an increased TSH response to TRH. The reverse is true in the presence of high circulating concentrations of thyroid hormones. The TSH response to a bolus injection of TRH has been used to diagnose the exact cause of hypo- and hyper-thyroidism, but measurement of thyroid hormones and TSH, using sensitive modern assays, is usually sufficient for diagnosis. Thus, in Clinical Case 3.1 concentrations of thyroid hormones in peripheral blood were high and the negative feedback resulted in unmeasurably low serum concentration of TSH.
This regulatory loop is affected by internal and external factors that alter the rate at which TSH is secreted. It is secreted in a pulsatile fashion with a diurnal variation, peaking around midnight. Environmental temperature may stimulate or inhibit the release of TSH by adjusting TRH secretion. Thus, after 24 h exposure to a cold environment, the plasma concentrations of thyroid hormones increase with a consequent rise in basal metabolic rate and an increase in the endogenous production of body heat. This effect is more marked in rats than humans. Pharmacological doses of glucocorticoids, as prescribed in anti-inflammatory therapy, or seen in Cushing's syndrome inhibit thyroid hormone secretions by reducing the TSH secretory response to TRH. In contrast, estrogens have the opposite effect, increasing TSH secretion and, hence, increasing the activity of the thyroid gland.
Hyperthyroidism — Graves' disease
The importance of the TSH receptor in regulating the activity of the thyroid gland is exemplified by Clinical Case 3.2. This patient has Graves' disease, an autoimmune disease resulting in the production of autoantibodies that stimulate the TSH receptor. This highly unusual action of an antibody (most block receptor activation) not only stimulates thyroid hormone synthesis and secretion but also thyroid growth. Autoantibodies to thyroglobulin and TPO may also be present.
Clinical Case 3.2
A 35-year-old woman came to the outpatient clinic with her 5-month-old child. She had noted increasing tenseness and irritability with her baby, poor sleep, weight loss and palpitations. Her husband was concerned that she had post-natal depression and had taken time off work to look after the family. She had an unremarkable past medical history and had sailed through pregnancy without any problems; she denied depression but felt exhausted all the time. She recalled that her late mother had an operation on her neck in her twenties and that her younger sister had regular vitamin injections for anemia. She ruefully admitted that the recent strain had led her to restart smoking ten cigarettes daily. On examination, she had a moderate diffuse goiter with an audible bruit, a tremor and a resting tachycardia of 100/min. Her eyes were prominent and puffy (see Box 3.16).
Like Clinical Case 3.1, this patient with hyperthyroidism is also a young woman with cardiac problems and a diffuse goiter indicating that the pathology was affecting all parts of the gland. The differences between these two cases are that in the second case there was a suggestive family history of thyroid disease, a recent pregnancy, evidence of eye problems and no evidence of an excess intake of iodine.
Incidence and diagnosis of Graves' disease
Graves' disease is the most common cause of hyperthyroidism and other causes are uncommon or, indeed, rare (Box 3.13). For reasons that remain unknown, it is much commoner in women, particularly those aged 30–50. It affects approximately 3% women and 0.3% men. The family history indicates a genetic predisposition to autoimmune disease. In Clinical Case 3.2, the patient's mother had had Graves' disease and her sister pernicious anemia (also considered to be autoimmune in nature). The exact nature of this genetic predilection remains uncertain but experimental studies have suggested linkage with a number of other genes. These include a linkage with certain histocompatibility complex genes (on chromosome 6) and associations with other diseases that are characterized by markers of autoimmunity, suggesting a primary genetic defect in immune function. The familial associations include pernicious anemia, Sjogren's syndrome, Addison's disease, type 1 diabetes mellitus and primary biliary cirrhosis.

Box 3.13
Causes of hyperthyroidism. Graves' disease (~80%) — autoimmune with stimulating antibodies to the TSH receptor. Toxic multinodular goitre (~ 15%).
The relationship of thyroid disease to a recent pregnancy is noteworthy for several reasons. First, pregnancy alters thyroid physiology and, overall, increases circulating concentrations of thyroid hormones (Box 3.14). Second, pregnancy is associated with an amelioration of symptoms associated with autoimmune diseases (i.e. immunosuppression) although the causes of this are not understood. Third, the immunological changes that occur post-partum induce a recrudescence of (or, indeed, first appearance of) autoimmune hyperthyroidism or hypothyroidism; it is likely that this caused the hyperthyroidism seen in Clinical Case 3.2. It is estimated the 2–15% women become hyperthyroid (often transiently) post-partum.
Graves' disease may be distinguished from other causes of hyperthyroidism because it is associated with abnormalities of the eyes and integument. The diagnosis may be confirmed by the detection of autoantibodies to the TSH receptor (Box 3.15). However, the presence of such antibodies is not essential to the diagnosis; indeed, the absence of autoantibodies is usually attributed to the assay.

Box 3.15
Assay of antibodies to the human TSH receptor — competitive binding assay. Principle: Patient's serum containing antibodies (Abs) is incubated with TSH receptors.
Ophthalmopathy and dermopathy of Graves' disease
The patient in Clinical Case 3.2 presented with prominent and puffy eyes and, indeed, eye disease is the most common associated feature of Graves' disease. Since the ophthalmopathy is linked to Graves' disease rather than other autoimmune diseases of the thyroid (such as Hashimoto’s disease, see below), it is generally considered that the eye disease results from autoimmune interactions within the orbit involving the TSH receptor. However, attempts to identify the expression of the TSH receptor protein or receptor fragments within the eye have been problematical. In addition, the eye disease may arise before, during or after raised thyroid hormone secretions. Thus, its etiology appears to be independent of thyroid function.
The features of Graves' ophthalmopathy are given in Box 3.16. There are three phases to the eye disease; an active period of inflammation is followed by regression and a third inactive or ‘burnt out’ period. Those elements of the disease that are expressed within the orbit are far more serious than those expressed extraorbitally because they can endanger vision. Within the orbit, antibodies stimulate the production of glycosaminoglycans by the fibroblasts. These attract large amounts of water and, thus, increase retro-orbital pressure causing bulging of the eyes (proptosis). The increased pressure may jeopardize the vascular supply to the eye. Inflammatory activity within the orbit (involving T lymphocytes, macrophages and local production of cytokines) may lead to muscle fibrosis and cause diplopia (double vision). In contrast, the extra-orbital lid retraction and chemosis do not threaten vision though they may be awkward for the patient.
Treatment of the severe symptoms of eye disease (Box 3.17) are problematic although minor symptoms such as grittiness or sensitivity can be treated with synthetic tears or darkened and wind protective glasses. An interesting point about Clinical Case 3.2 is that she was a smoker. Smoking increases the risk of thyroid eye disease some 7-fold, although the relationship to the number (or duration) of cigarettes smoked is poorly defined and the cause unknown.

Box 3.17
Treatment of thyroid eye disease.
The effects of Graves' disease on the integument are shown in Box 3.18. Graves' dermopathy is the least frequent of all the associated symptoms and even less is known about its etiology. Like the ophthalmopathy it is associated with an increased production of glycosaminoglycans (predominantly hyaluronic acid and chondroitin sulfate) that causes edema, as in the orbit.
Treatment of hyperthyroidism
There are three methods of treating hyperthyroidism — drugs, surgery and radioisotopes. None is ideal; each has its own advantages and disadvantages (Box 3.19); none is exclusive and, not infrequently, a combination of therapies is used.

Box 3.19
Treatments of hyperthyroidism.
Antithyroid drugs
There are three types of drugs used to inhibit thyroid hormone synthesis and release.
Thiocarbamides
These are the most widely used and were developed after the discovery that feeding certain plants (particularly those of the genus Brassica) to animals caused goiter formation. Thiocarbamides reduce thyroid hormone synthesis by inhibiting TPO. The synthetic thiocarbamides now used are carbimazole (or its main metabolite methimazole in the US) and propylthiouacil (abbreviated to PTU) (Box 3.19). They have short half-lives, particularly so in the case of PTU, and for this reason carbimazole is more widely used. However, PTU tends to be prescribed to pregnant and lactating women because it binds to plasma proteins and less crosses the placenta or enters the breast milk. It also has the added advantage of reducing the hepatic conversion of the less active T4 to T3 (Box 3.29).
High doses of anti-thyroid drugs are initially prescribed to patients with Graves' disease and the dose gradually reduced whilst aiming to keep the patient euthyroid. Alternatively some clinicians use the ‘block and replace’ regimen in which patients are maintained on high doses of anti-thyroid drugs with replacement thyroxine as appropriate.
Anti-thyroid treatment is continued for a somewhat arbitrary length of time (about 18 months) with the hope that the patients will be ‘cured’ (in remission) after a single course. Unfortunately, many patients relapse after stopping the drug.
Iodine
The fastest acting anti-thyroid agent is iodine itself, reducing thyroid hormone synthesis within three days through a presumed autoregulatory mechanism. It may be given as drops of ‘Lugol's solution of iodine’ or a saturated solution of potassium iodide for 10 days or so. This form of treatment is no longer widely used but is particularly useful in the short term in hyperthyroid ‘storm’ where the degree of thyrotoxicosis becomes life threatening (with, for example, cardiac failure). It has also been used prior to thyroid surgery to reduce the high blood supply to the gland.
Perchlorate
Perchlorate reduces thyroid hormone synthesis by competing with iodine for uptake into the thyroid gland and discharging iodine from the trapped pool. It is no longer used routinely because it is relatively toxic with adverse effects on bone marrow. However, it is used in cases such as that of Clinical Case 3.1 in which there has been an acute excess of iodine.
The patient in Clinical Case 3.1 was treated with a combination of perchlorate and carbimazole and her anti-arrhythmic drug was changed to one that did not contain iodine, a β-adrenergic receptor blocker that has additional benefits in hyperthyroidism, particularly on cardiac symptoms.
Destruction of thyroid tissue is the alternative to drug therapy. This can be done using radioactive isotopes of iodine or surgically.
Radioiodine
Radioactive isotopes of iodine have been in use for over 50 years and today 131I, with a radioactive half-life of 8 days is the most commonly used for therapy. Since it has proved almost impossible to calculate a dose of the isotope that would result in euthyroidism in individual patients and 131I treatment is remarkably safe, many clinicians now use a fixed dose of isotope (for example 15mCi or 550MBq) and aim to produce hypothyroidism. Once hypothyroidism is induced, such patients can be treated with oral thyroxine that is cheap, effective and easy to monitor. 131I is also used to treat euthyroid goiters (so as to avoid surgery) and such treatment usually results in a reduction of about 40% in the volume of the thyroid gland.
Thyroid surgery
The ancient Egyptians considered that a goiter added grace and beauty to a female neck. Whilst most women in the 20th century might not agree with this view, they are often reluctant to exchange a goiter for a 7 cm scar on the neck. Thus, unless the goiter is malignant, surgery is usually restricted to large and unsightly goiters that are not treatable medically or with radioiodine. It is important to note that, prior to surgery, the patient must be rendered euthyroid with drugs because of the increased risks of anesthesia and surgery in the hyperthyroid patient.
Surgical anatomy and embryology of the thyroid gland
The surgical anatomy of the thyroid gland is complex and important because of its proximity to the common carotid artery and internal jugular vein, the laryngeal and vagus nerves and its close association with the parathyroid glands (Box 3.20). The thyroid gland is the first endocrine gland to develop in the embryo and begins to form about 24 days after fertilization. Like Rathke's pouch, that develops into the anterior pituitary gland (see Box 7.2), the thyroid gland also develops from an outgrowth of the pharyngeal endoderm. As the embryo grows the thyroid gland descends into the neck and for a short time the gland is connected to the developing tongue by a narrow tube, the thyroglossal duct. At around 7 weeks, the gland has assumed its definitive shape and has reached its final destination in the neck. By this time the thyroglossal duct has normally disappeared although its remnants persist as a small pit, the foramen cecum (Box 3.21C).

Box 3.20
Schematic views of the surgical anatomy of the thyroid gland. Anterior of the blood supply and relationship to other important vessels
Left lateral view of the relationship to important nerves
The parathyroid glands that are closely associated with the thyroid gland also develop from the pharyngeal endoderm, in this case the 3rd and 4th pharyngeal pouches (see Box 3.21B). The inferior parathyroid glands (sitting at the lower poles of the two lobes of the thyroid gland) migrate with the thymus gland, and the superior parathyroids from the 4th pharyngeal pouch migrate laterally.
The embryology of these glands results in three important clinical sequelae (Box 3.21D). First, the thyroglossal duct may not disappear completely and this may result in mid-line thyroglossal cysts. The connection with the tongue means that such cysts move upwards when the tongue is protruded. Second, ectopic thyroid and parathyroid tissue may be found in a number of positions from the tongue to the chest. Third, surgery to the thyroid gland may result in the removal of parathyroid tissue and subsequent hypoparathyroidism.
Primary hypothyroidism — Hashimoto's disease and myxedema
The most common causes of hypothyroidism are autoimmune in etiology (Box 3.22). Like Graves' disease they may have a number of autoimmune markers, including the presence of autoantibodies. However, unlike Graves' disease, T-cell mediated actions result in thyroid gland destruction rather than stimulation. The symptoms of hypothyroidism are vividly demonstrated in the next case.

Box 3.22
Causes of hypothyroidism. Common (~ 95%) Hashimoto's disease — autoimmune thyroid destruction
Clinical Case 3.3
A 19-year-old young man presented to the Emergency Room with pain in the left hip and knee. X-rays showed that he had a slipped femoral epiphysis A . At the age of 19.7 years, he was 1.544 m tall and weighed 57 kg. His mother, who had type 1 diabetes mellitus and hypothyroidism, reported him to be a ‘lazy sod … difficult to get out of bed in the morning’. His schoolwork had been poor and he had worn sweaters even during the summer. He looked much younger than his age C and, indeed, his bone age (an index of skeletal development) was markedly delayed at 13.4 years. His pubertal development was also delayed by several years (Tanner stage 3) and his serum testosterone concentration was low at 4 nmol/l (NR 9–25 nmol/l). A moderate size goiter was palpable.
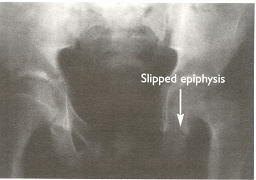
X-ray appearances of the hips at presentation. Comparison of the epiphyses of the right and left femurs shows that the left has ‘slipped’ sideways at the junction with the diaphysis (arrowed).
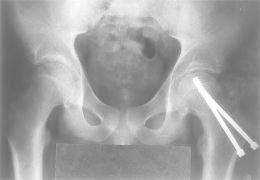
X-ray appearances of the hips after surgical correction.

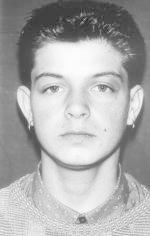
Facial appearance at presentation (left) and after 7 months treatment of T4 (right).
Hashimoto's disease is similar to Graves' disease in that it occurs with about the same incidence and with the same sex bias, peak age of presentation and family history of thyroid disease or related autoimmune diseases. In this regard, Clinical Case 3.3 was atypical in being male and younger, but serves to illustrate the catastrophic effects of the lack of thyroid hormones.
Etiology and clinical features of Hashimoto's disease
The cause of the autoimmunity is unknown and since the autoimmune processes in Hashimoto's disease induce destruction of the thyroid gland, it may be surprising to note that it is marked by goiter formation. The goiter is the result of diffuse lymphocytic infiltration (which gives it a number of alternative names such as chronic lymphocytic thyroiditis), together with TSH-stimulated hyperplasia of surviving thyroid tissue due to loss of feedback inhibition from the thyroid hormones. The goiter is usually diffuse with a characteristic — at least to the experienced clinician — ‘rubbery’ feel to palpation. It is rare for it to be painful or problematic by its size and surgery is infrequently required. Other features of this disease are antibodies against TPO and thyroglobulin (usually in much higher titers than in Graves'). Some patients are affected by a period of hyperthyroidism, in which case it is termed ‘Hashitoxicosis’, but the degree of hyperthyroidism is usually milder than in Graves' disease.
Hypothyroidism is also associated with primary thyroid atrophy and termed primary myxedema. The relationship between Hashimoto's disease and myxedema has been much discussed but it seems highly likely that non-goitrous myxedema is simply an end-stage of autoimmune destruction leaving only fibrous remnants. A role for antibodies to the TSH receptor that block the actions of TSH (rather than stimulating the receptor as in Graves' disease) has also been proposed.
The symptoms of hypothyroidism are extremely diverse — as might be predicted (Box 3.23). Clinical Case 3.3 showed a number of classic features including those affecting mental processes, gut motility, metabolic rate and the skeleton. He also presented with pubertal delay, though it is to be noted that hypothyroidism can occasionally present in the teenage years with precocious puberty.

Box 3.23
Clinical features of hypothyroidism.
Diagnosis of hypothyroidism
The diagnosis of Hashimoto's disease is usually easy, based on the presence of goiter, circulating thyroid autoantibodies and low circulating concentrations of thyroid hormones with high TSH concentrations. Biochemical investigation in Clinical Case 3.3 was performed to investigate the cause of his poor growth and pubertal delay that culminated in his presentation to orthopedic surgeons with a slipped femoral epiphysis. These revealed total serum T4 to be unmeasurable at <10 nmol/l (NR 70–150 nmol/l) with a very high serum TSH (>110 mU/l), NR 0.5–4 mU/l. Anti-TPO antibodies were positive in high concentration. Surgical procedures in hypothyroidism are associated with increased risk of morbidity and mortality. He was started on oral thyroxine therapy and subsequently underwent surgery. After some 7 months, he was seen in the out-patient department complaining that his friends did not recognize him (Clinical Case 3.3, C). His growth and pubertal development matched the change in his facial appearance.
Treatment of primary hypothyroidism
Use of the prohormone thyroxine in the treatment of primary hypothyroidism is cheap and easy to monitor. The conversion of T4 to T3 is physiologically regulated and the dose can be altered according to serum TSH concentrations. No attempt is made to treat the underlying immune disorder. Severe hypothyroidism resulting in ‘myxedema coma’ is not often seen nowadays but may require parenteral T4 or T3 in addition to general supportive measures and hydrocortisone.
It is to be noted that some physiological conditions such as pregnancy may increase T4 requirements whilst gastrointestinal diseases or drugs such as sucralfate may decrease its absorption. Requirements may also be increased by drugs increasing its clearance such as rifampicin whilst some such as amiodarone may decrease T4 to T3 conversion. Diseases such as cirrhosis of the liver or the natural processes of ageing may decrease requirements.
The terms ‘compensated hypothyroidism’ or ‘decreased thyroid reserve’ have been used to indicate the situation in which circulating T4 or T3 concentrations are low-normal but serum TSH concentrations are elevated. There has been discussion over when such patients should be treated with T4. In this controversial area, some have argued that patients with serological evidence of anti-thyroid antibodies are likely to develop clinical hypothyroidism and should be treated.
Other forms of thyroiditis
The term thyroiditis is a term applied to a number of conditions that arise as a result of inflammation of the thyroid gland and, as has been seen, Hashimoto's chronic lymphocytic thyroiditis is the most common. Others are thought to result from infections and give rise to very different clinical features.
Bacterial or fungal infections may precipitate acute thyroiditis whilst subacute thyroiditis, such as De Quervain's, has been attributed to a viral illness. De Quervain's is usually self-limiting, but with a tendency to recur and the diagnosis is made on clinical features (general malaise and a painful thyroid gland). If fine-needle aspiration cytological examination (FNA, see Box 3.33) is performed it may reveal the presence of inflammatory cells. The disease process is associated with evidence of an acute phase reaction (a non-specific reaction leading to the high erythrocyte sedimentation rate) and the release of thyroid hormones from damaged cells. It is not due to hyperfunctioning thyroid tissue and 99mTc scans show reduced or absent iodine uptake (Box 3.6) and thiocarbamides play no part in treatment.
Some affected patients (approximately 50%) may have some symptoms of hyperthyroidism and, in a later phase of the illness, a temporary period of hypothyroidism may be experienced. During the acute phase, anti-inflammatory oral glucocorticoids (e.g. prednisolone) bring about rapid symptomatic relief.
Riedel's thyroiditis is not associated with features of inflammation but has additional features of fibrotic reaction elsewhere (e.g. retroperitoneal fibrosis in the abdomen). Like Hashimoto's disease, it results in progressive destruction of the thyroid gland that may necessitate T4 replacement therapy.
Secondary hypothyroidism
Less often, hypothyroidism may result from a loss of trophic stimulation due to damage or disease of the hypothalamic-pituitary axis. Thus, in the absence of the TRH and/or TSH drive, thyroid function is reduced. Clinical Case 3.4 illustrates some of the clinical features of hypothyroidism and indicates how secondary hypothyroidism can be distinguished from primary hypothyroidism.
Clinical Case 3.4
A 28-year-old man was seen in the outpatient clinic with his wife. He had noted gradually worsening tiredness and fatigability, making his job difficult to maintain. He had gained weight (approximately 3 kg) and his wife had noted that he had worn an extra sweater during the winter months. His feet had been cold in bed and his hair and skin dry. His wife has had to take time off from work to bring her husband to the clinic (because her husband's car was being repaired following damage to both the near- and offside wings) and was naturally keen to see things sorted out quickly. The blood tests showed that the serum T4 was low and the TSH was normal. Thyroid autoantibodies were negative.
This patient had clinical features typical of hypothyroidism. Whilst autoimmunity is the commonest cause of both primary hyper- and hypothyroidism, two noteworthy features indicate that this was not the cause of the hypothyroidism seen in this case. First, the patient was a young male and second, the serum TSH concentration was normal in the face of a low T4 concentration. Thus, in the absence of raised concentrations of TSH with low concentrations of thyroid hormones (as seen in primary hypothyroidism) the cause lay at the level of the hypothalamus or pituitary gland. The fact that the patient had damaged both front wings of his car was another clinical indicator. He has a visual field defect (see Box 7.1) caused by a large benign pituitary tumor that extended above the pituitary fossa and pressed on the optic chiasm. The patient had secondary hypothyroidism due to hypopituitarism.
Treatment of secondary hypothyroidism
Clinical Case 3.4 raised the clinical problem of how much T4 should be given to replace a deficit. Over the last 20–30 years (and as assays for TSH have improved), the daily dose of T4 has been reduced from 300–400 μg to about 125–175 μg (approximately 1.5 μg/kg ideal weight in adults). In a patient with primary hypothyroidism, there is general agreement that the amount of thyroxine given should be titrated against measurements of the serum TSH, aiming to keep this parameter within the normal range. This, of course, assumes normal function of the enzyme that converts T4 to T3 and is important in mediating the feedback effects of thyroid hormones on the pituitary gland. However, in a patient without an intact feedback loop and in the absence of a cheap, reliable measure of T3 action in peripheral tissues, the replacement dose is generally guessed in the range 100–200 μg. The question of combined T3 and T4 replacement (mimicking the natural secretion of the thyroid gland) is under active discussion.
Hypothyroidism in infancy and childhood
Clinical Case 3.3 illustrated the dramatic effects of hypothyroidism during pubertal development. However the effects are worse (and permanent) when hypothyroidism occurs in fetal, neonatal and early childhood. An athyroidal or hypothyroidal neonate occurs in approximately 1:4000 live births and, since thyroid hormones are essential for normal development of the nervous and skeletal systems, neonatal screening programmes are used (Box 3.24). These involve measuring serum concentrations of TSH by a sensitive immunoradiometric assay (Box 3.25) and such tests ensure that all affected neonates receive T4 replacement as early as possible after birth.
The most severely affected infants will, however, be left with a permanent decrease in psycho-neurological function, probably reflecting intrauterine events. If there is any doubt about thyroid status (for example, with borderline results), it is better to replace thyroxine up to the age of, say, 2 years when treatment can be stopped under supervision and reassessment performed. The usual dose given is up to ten times the adult mean dose calculated on surface area. In childhood, acquired hypothyroidism (usually autoimmune) characteristically presents, as has been seen, with poor growth, epiphyseal dysplasia or poor school attainment. All features respond very well to T4 replacement.
Goitrous hypothyroidism in childhood may be due to defects in the synthesis of thyroid hormones. The best recognised of these is Pendred's syndrome that is associated with congenital deafness and a defect in the synthesis of a protein (pendrin) thought to be involved in sulfate transport.
Thyroid hormone resistance
The inability of target cells to respond to a hormone is known as hormone resistance and this is a common cause of endocrine disorders. Resistance can result from a reduction or loss of hormone receptors, or an aberrant receptor or signalling molecule.
Thyroid hormone resistance does exist but, compared to the millions of people exhibiting insulin resistance, the number of people showing resistance to thyroid hormones is minute. Complete resistance to thyroid hormone has never been recorded (it may be incompatible with life) and mutations causing resistance have only been found in the TR-β receptor (Box 3.9).
The key clinical features of thyroid hormone resistance are attention deficit hyperactivity disorder, developmental delay, learning disability, deafness and impaired growth in children with goiters. Patients with a generalized resistance and with signs and symptoms of hypothyroidism are treated with doses of thyroxine that facilitate normal growth and development. Paradoxically, some patients with thyroid hormone resistance exhibit symptoms and signs of hyperthyroidism. This may result from relatively greater resistance at the pituitary gland than in peripheral target tissues resulting in TSH secretion inappropriately high for these tissues. In such patients, beneficial effects have been seen with TRIAC (see Box 3.29) or with the D isomer of T4 that have a higher affinity for the thyroid hormone receptor than T3.
Non-thyroid illness (‘sick euthyroid’ syndrome)
Measurements of serum thyroid hormone and/or TSH concentrations are often abnormal in patients hospitalized for acute illness (Box 3.26). These are usually reversible disturbances detected by biochemical assays but without associated symptoms of hypo- or hyperthyroidism. Such a condition has been termed ‘sick euthyroid’ syndrome, although a better term is ‘non-thyroid illness’. It is considered to result from the effects of acute illness and/or the drugs treating the illness (Box 3.27) on the synthesis, transport and metabolism of hormones. To understand these it is necessary to discuss the transport and metabolism of thyroid hormones and the concept of ‘total’ and ‘free’ circulating hormone concentrations.

Box 3.27
Drugs and the thyroid gland. Drugs can alter thyroid hormone status by affecting thyroid hormone synthesis, transport or metabolism. They act at a number of sites to: Block I- uptake, e.g. lithium.
Transport and metabolism of thyroid hormones
The iodothyronines are virtually insoluble in water and, once released from thyroglobulin, they are very rapidly bound to the plasma proteins, transthyretin (previously called thyroxine-binding prealbumin), thyroxine-binding globulin (TBG) and albumin. These vary in their capacity and affinity for T3 and T4 (Box 3.28); about 70% of circulating thyroid hormones are bound to TBG. Only a tiny fraction (<0.5%) of released thyroid hormones exist in a free form in the circulation and this is in equilibrium with the bound forms of thyroid hormones. The free-hormone hypothesis states that it is only the free thyroid hormones that act on target cells. The bound forms are considered to act as a circulating reservoir. There remains controversy as to whether the ratio of bound to free hormone changes as blood passes through the capillary bed of an organ.
Assays for thyroid hormones (see below) may measure the ‘total’ T4 or T3 or the respective ‘free’ fractions. Any reduction of serum TBG concentration (e.g. reduced synthesis in liver disease or increased loss in kidney disease) reduces the concentrations of total T4 and total T3. The feedback loop ‘senses’ changes in free hormone concentration and TSH secretion is modulated to maintain this. Similar compensations occur when TBG concentrations increase, most commonly as a result of pregnancy or estrogen administration.
Eighty per cent of the total thyroid hormones secreted each day is T4 but this is relatively inactive at nuclear receptors and, thus, considered to be a prohormone. Approximately 70–80% of released T4 is converted by deiodinases to the biologically active T3, the remainder to reverse-T3 (rT3) which has no significant biological activity. Deiodinases are unusual selenium-containing enzymes that are present in a number of tissues and are responsible for the metabolism of thyroid hormones (Box 3.29).
Removal of an iodine atom from the 5th carbon atom (5′) of the outer tyrosine ring of T4 by Type 1 and Type 2 deiodinases produces T3 whilst deiodination of the inner (5) tyrosine ring by Type 1 and Type 3 deiodinases produces rT3. Further deiodinations at the 3rd and 5th carbon atoms of both outer and inner tyrosine rings produce increasingly inactive diiodo- and monoiodo-thyronines and at the same time conserving iodine. Iodothyronines are excreted in the urine although some T3 and T4 is conjugated with glucuronide and excreted via the bile in the feces.
The decreased circulating concentrations of T3 in the severely ill may be due to a reduction in 5′ deiodinase activity as a result of the low calorie intake and the raised glucocorticoid secretions in response to stress. This reduces the conversion of T4 to T3 and, concomitantly, the conversion of T3 to T2.
The term ‘sick euthyroid syndrome’ presupposes that the results of all the biochemical changes are neutral with regard to thyroid hormone function. There is, in fact, little evidence to support this. However, it can be said that generally thyroid hormone treatment for the severely ill does not bring any clinical benefits.
Biochemical measurements of thyroid hormone status
Assays of circulating thyroid hormone concentrations are usually referred to as ‘thyroid function tests’, often abbreviated to ‘TFTs’. It is clear that they do not measure thyroid ‘function’ since this implies a measure of the effectiveness of thyroid hormone on peripheral tissues, but they are used as surrogate measures. Total (bound plus free) thyroid hormones can be measured by a competitive binding assay after chemically removing the binding proteins. As discussed above, this measurement may not reflect the physiological state since changes in the concentration of binding proteins alter the total hormone concentration. It is possible to measure the concentration of free thyroid hormones in serum samples by an indirect assay method (Box 3.30).
It is to be emphasized that, in the presence of an intact feedback loop, serum TSH concentration reflects the effects of thyroid hormones on the pituitary gland. For this reason serum TSH concentration has been recommended as first-line assay of ‘thyroid function’.
The results of the biochemical tests obtained in the clinical cases may now be interpreted. In Clinical Case 3.1 (iodide-induced hyperthyroidism), the concentrations of circulating thyroid hormones were high (total T4 310 nmol/l, NR 55–150 nmol/l) and, as a result of negative feedback, the peripheral concentration of TSH was low (<0.05 mU/l, NR 0.5–4.0 mU/l). There were no detectable anti-thyroid autoantibodies. In Clinical Case 3.2 (Graves' disease), the concentrations of thyroid hormones were again high (total-T4 320 nmol/l, NR 70–150 nmol/l) and the serum TSH concentration suppressed (<0.05 mU/l, NR 0.5–4.0 mU/l). The autoantibody titers (measured by the binding assay — see Box 3.15) were high, not only those against the TSH receptor but also to thyroid peroxidase and thyroglobulin. In Clinical Case 3.3 (primary hypothyroidism), the operative hypothalamo-pituitary feedback loop led to high circulating TSH concentrations (>110 mU/l, NR 0.5–4.0 mU/l) in response to the unmeasurable total-T4 (<5 nmol/l, NR 70–150 nmol/l). In contrast, Clinical Case 3.4 (secondary hypothyroidism) the loss of the feedback loop resulted in a normal circulating concentration of TSH, 2.5 mU/l (NR 0.5–4.0 mU/l), despite low circulating concentrations of thyroid hormones (free-T4 4 pmol/l, NR 11–23 pmol/l).
Thyroid growth
It has been seen that activation of the TSH receptor (whether by the hormone itself or by autoantibodies) is a potent stimulator to thyroid growth; since the receptors are present on every follicular cell, such growth tends to be diffuse.
Experimental studies, however, have shown that numerous cytokines and growth factors can affect thyroid function and these may have clinical implications. For example, they may exert overall inhibitory effects on the hypothalamic-pituitary-thyroid axis and could account for some of the effects seen in non-thyroidal illness. Cytokines may also be involved in the etiology of autoimmune responses and it is noteworthy that the thyroid gland produces more cytokines than any other endocrine gland. Like cytokines, growth factors are present at all levels of the axis and there is evidence that insulin-like growth factor potentiates TSH action on thyrotrophs. This could account for the growth of some nodules.
Nodular thyroid disease
In contrast to diffuse goiters, sometimes focal abnormalities arise that affect only certain cells in the thyroid gland and give rise to nodules. These may be ‘hot’ (i.e. take up radioactive iodine and show increased thyroid hormone synthesis) or ‘cold’ (i.e. non-functional) (see Box 3.6).
Clinical Case 3.5
A 53-year-old woman came to the outpatient clinic. She had no symptoms but gave a history of a lump in her neck being noticed by her primary care physician during a routine ‘well-woman’ check. There was no family history of thyroid disease and she had a blameless past medical history. She was a nonsmoker and was on no medication apart from estrogen replacement therapy for menopausal hot flushes. She had not noticed any change in her voice, or difficulty swallowing or breathing. Examination was entirely normal except for a 3 × 2 cm single nodule in the left lower thyroid pole. Blood tests showed that her total T4 was 196 nmol/l (NR 70–150nmo/l), free T3 7.8 pmol/l (NR 4.0–8.1pmol/l), TSH 2 mU/l (NR 0.5–4.0 mU/l) and thyroid autoantibodies were not present in serum.
In this patient, the serum total T4 was raised whilst free T3 and TSH were normal, indicating that she was euthyroid. The high concentration of total T4 was due to an estrogen-induced increase in TBG. Estrogen increases glycosylation of this protein and, thus, reduces its metabolism. This resulted in an increase in bound T4 and a consequent increase in T4 secretion to maintain normal concentrations of ‘free’ T4. The 99mTc scan showed normal uptake of the radioactivity but with an area of decreased uptake in the region of the nodule (Box 3.6). This indicated that the nodule lacked the iodide symporter and, thus, it was a non-functional or ‘cold’ nodule.
Prevalence and etiology of benign nodular disease
Studies of populations (such as Whickham in Northern England or Framingham in the US) have indicated that multinodular thyroids occur in around 5% of the population with a marked female preponderance (10:1). Autopsy studies have indicated a much higher incidence of nodular thyroid disease, indeed up to 50%, with multinodular disease outnumbering single nodules by about 4 to 1. The incidence increases markedly in people over 50 years of age. It is much higher in areas of iodine deficiency indicating the importance of iodine in the etiology of nodularity.
The etiology of benign nodular disease is not well understood although in some cases it is due to activating mutations of the TSH receptor or G-protein signalling system (Box 3.12). When such activating mutations of the TSH receptor are in the germ line (which is very rare) these will be passed on to succeeding generations appearing as familial hyperthyroidism.
The clinical index of a nodule, which makes no assumption of the underlying pathology, is the ability to detect one by palpation. Generally, (fat necks notwithstanding) this needs a lump 1 cm in diameter before it can be felt. However, if ultrasound scanning is used, it is more often than not that nodules some 2–3 mm in diameter are detected. The clinical relevance of such micronodularity detected on an ultrasound scan is very doubtful. However, the detection of a thyroid nodule(s) by palpation raises the important clinical question; is the lump malignant?
Thyroid cancer
Tumors of the thyroid gland may be primary (arising from cells within the thyroid gland) or secondary due to malignant cells which have spread from other tissues (Box 3.31). Those arising from parafollicular cells which secrete calcitonin (giving rise to medullary cell carcinomas) are discussed. Lymphomas may arise from infiltrating lymphocytes and, though they are much more common in Hashimoto's disease, they are still very rare. Other tumors such as sarcomas from smooth muscle cells within the gland are also exceedingly rare.

Box 3.31
Classification of thyroid epithelial cell tumors. These are classified according to histological features Adenomata
The majority of primary tumors arise from epithelial cells of the thyroid gland and are, therefore, termed adenomas if benign and carcinomas if malignant. The epithelial cell tumors are sub-classified as either papillary or follicular according to their histological appearance. These tumors are found more often in women (2 to 4 fold more often than men), aged 45–50 years. In areas with adequate iodine intake, the commonest tumor is papillary, accounting for some 80% of all tumors. Where iodine intake is low there is a relative increase in follicular and anaplastic carcinomata, though no overall increase in frequency. A number of factors, both genetic and environmental has been implicated in the etiology of epithelial tumors (Box 3.32).
Thyroid cancer conundra
There are three conundra. The first is that, whilst nodular thyroid disease is common in the general population, only about 30 people in a million are diagnosed clinically with thyroid cancer annually and 6 people in a million die each year of the disease. This is a minute fraction of all cancer deaths and the risk of thyroid cancer causing death is extremely low.
The second conundrum arises because it is difficult to differentiate benign from malignant primary epithelial tumors by their histological appearances. The commonly used criteria of histological malignancy are invasion of blood vessels or lymphatics and breach of the capsule containing the nodule cells. When thyroid glands are examined at autopsy the histological features of papillary cancer are seen in 5 to 25% of thyroid glands examined. Thus, thyroid nodules and histological appearances of thyroid cancer are extremely common yet clinical disease is rare.
Clinical suspicion of malignancy may be raised by the speed of growth of a nodule or the involvement of the esophagus, trachea or recurrent laryngeal nerve (giving a hoarse voice and difficulty in breathing or swallowing). However, the only certain clinical benchmark is the behavior of the tumor; if it spreads (or metastasizes) it is malignant.
Differentiating the few malignant tumors from a large number of benign nodules has exercised clinicians for many years and, though various imaging techniques have been used, most centers now use fine needle aspiration cytological examination (Box 3.33). Typically, FNA cytology is used to separate diagnoses into five categories.
The third conundrum arises because death rates from differentiated thyroid cancer are extremely low and distinguishing malignant from benign nodules is problematic; how should individual patients be treated? Prognosis is the most important consideration and whilst various staging systems have been used to predict this, none seems better than the long established Tumor (T), Node (N), Metastasis (M) or TNM classification (Box 3.31). In general, the bigger the tumor, and the less well differentiated it is the worse the prognosis. Age is the other very important factor and patients under about 45 years have a better prognosis.
There is no general agreement on the degree of surgery and post-operative radioiodine or radiotherapy but, given the risks of thyroid surgery (Box 3.19), it has been argued that low risk patients should have less aggressive surgery (e.g. lobectomy rather than total thyroidectomy). Although the experimental data were obtained from studies on rodents, there is general agreement that patients with differentiated thyroid cancer are treated with T4 to suppress TSH secretion (via the negative feedback effect). Measurements of serum thyroglobulin (an index of TSH stimulation on the thyroid gland) may be used to monitor the effects of exogenous T4.
The patient in Clinical Case 3.5 underwent FNA. Cyst fluid was removed with resolution of symptoms. Cytological examination showed no evidence of malignancy.
Clinical case questions
Clinical Case Study Q3.1
A 43-year-old old female nurse was referred to the endocrine clinic. She weighed 110 kg and was 1.67 m tall. Her primary care physician had taken her blood for routine tests and had found the total T4 to be 257 nmol/l (NR 70–150 nmol/l) with a free T3 of 12.7 pmol/l (NR 4.0–8.1 pmol/l) and a TSH of <0.05 mU/l (NR 0.5–4.0 mU/l). There was no family history of any endocrine disease and her serum thyroid autoantibodies were normal. The primary care physician had treated the patient for several months with carbimazole 30 mg daily with no change in her biochemical tests of thyroid function. He therefore stopped the carbimazole and referred the patient for further investigation. A 99mTc scan of the thyroid gland was performed 4 weeks after cessation of the carbimazole (see Box Q3.1a).

Box Q3.1a
This 99Tc scan of Clinical Case Study Q3.1 taken at a time when the patient denied taking any medications for several months. (Compare the image with a normal scan (shown in Box 3.6).
Question 1: Using your knowledge of the hypothalamo-pituitary-thyroid axis feedback loop, how may the serum assays be interpreted and what are the possible diagnoses?
Question 2: How would you distinguish between these possibilities?
Clinical Case Study Q3.2
A 59-year-old old Pakistani woman was admitted via the Emergency Room with a 4-week history of fever (with profuse night sweats), malaise, nausea, but no vomiting. Her symptoms had not responded to two courses of antibiotics prescribed by the primary care physician. Over the same time, she had noted a sore throat with pain radiating to the ears. There was a strong family history of type 2 diabetes mellitus and no family or contact history of TB. On examination, she had a temperature of 38.5°C, a pulse rate of 120 beats/min and a tender diffuse goiter (Box Q3.2a). She was noted to be mildly anemic (Hb 10.9g/dl) with an elevated peripheral blood white cell count (14.3 × 109/l). A number of investigations were performed to find a source of infection to no avail. Her free T4 was 55 pmol/l and the serum TSH was unmeasurable (<0.05 mU/l). (Box Q3.2a) 99mTc scan of the thyroid was performed (Box Q3.2a).

Box Q3.2a
The small diffusely enlarged thyroid gland. The 99mTc scan of the patient performed during her admission.
Question 1: Given the clinical features and the serum assay results, what is the most likely diagnosis?
Question 2: In light of your answer to question 1, how should the 99mTc scan of the thyroid be interpreted?
Question 3: How should she be treated?
Clinical Case Study Q3.3
A 34-year-old patient on the post-natal ward requested that her midwives send urgently for the duty pediatrician because of concerns for her 5-h-old baby. He was feeding poorly and his respiratory rate was rapid. Five years previously she had been treated for Graves' disease with courses of carbimazole and later radioactive iodine. The pediatrician found her son (Box Q3.3) to have a characteristic facial appearance with staring eyes, a marked tachycardia and fever.

Box Q3.3
Photograph of the child in Clinical case study Q3.3 taken by his mother a few weeks after birth
Question 1: What is the diagnosis and how is it related to the mothers previous medical history?
Question 2: How can it be confirmed and treated?
Question 3: Could it have been predicted and what is the likely prognosis?
- Iodine intake
- Anatomical features of the thyroid gland
- Iodine trapping and thyroid function
- Synthesis of thyroid hormones
- Actions of thyroid hormones
- Control of thyroid hormone synthesis and secretion
- Hyperthyroidism — Graves' disease
- Surgical anatomy and embryology of the thyroid gland
- Primary hypothyroidism — Hashimoto's disease and myxedema
- Secondary hypothyroidism
- Hypothyroidism in infancy and childhood
- Thyroid hormone resistance
- Non-thyroid illness (‘sick euthyroid’ syndrome)
- Transport and metabolism of thyroid hormones
- Biochemical measurements of thyroid hormone status
- Thyroid growth
- Nodular thyroid disease
- Thyroid cancer
- Clinical case questions
- The thyroid gland - EndocrinologyThe thyroid gland - Endocrinology
- The parathyroid glands and vitamin D - EndocrinologyThe parathyroid glands and vitamin D - Endocrinology
Your browsing activity is empty.
Activity recording is turned off.
See more...