NCBI Bookshelf. A service of the National Library of Medicine, National Institutes of Health.
Benzie IFF, Wachtel-Galor S, editors. Herbal Medicine: Biomolecular and Clinical Aspects. 2nd edition. Boca Raton (FL): CRC Press/Taylor & Francis; 2011.
8.1. INTRODUCTION
Ginseng is a medicinal plant widely used for the treatment of various conditions. The pharmacological effects of ginseng have been demonstrated in cancer, diabetes, cardiovascular diseases and have been used for promoting immune function, central nervous system (CNS) function, relieving stress, and for its antioxidant activities (Jung and Jin 1996). The root of Panax ginseng C. A. Meyer, which is known as Korean or Asian ginseng, is a valuable and an important folk medicine in East Asian countries, including China, Korea, and Japan, for more than 2000 years. Panax is derived from the word “panacea,” which means a cure for all diseases and a source of longevity as well as physical strength and resistance. As the use of traditional Chinese herbs for medicinal and dietary purposes becomes increasingly popular in Western countries, sales of P. ginseng are increasing in North America and Europe as well as in other parts of the world.
The major bioactive components of P. ginseng are the ginsenosides, a group of saponins with dammarane triterpenoid structure (Huang 1999). Almost 50 ginsenosides have been isolated from P. ginseng root (white and red ginsengs), and novel structures continue to be identified, particularly from Panax quinquefolius (American ginseng) and Panax japonica (Japanese ginseng) as well as their berries (Gillis 1997; Yoshikawa et al. 1998; Attele et al. 2002; Christensen 2009). In this chapter, we review the structural and pharmacological properties of ginseng, and its active constituents, including ginsenosides, polysaccharides, and polyacetylenic alcohols. The pharmacological and clinical usages of ginseng, particularly ginsenosides, are discussed in relation to its anticancer, antidiabetes, immunomodulatory functions, and improving CNS functions including learning, memory, and neurodegenerative diseases.
8.2. STRUCTURAL PROPERTIES OF GINSENG
Ginsenosides, known as ginseng saponins, are the major components of ginseng and are classified into two major groups by the type of their aglycones, namely protopanaxadiol (PPD) and protopanaxatriol (PPT). PPD and PPT have dammarane triterpenoidal skeletons with sugar moieties binding at C-3, C-6, and C-20 positions (Huang 1999). The genuine structures of the PPD and PPT ginseng sapogenins are dammar-24-ene-3β,12β,20(S)-triol(PPD) and dammar-24-ene-3β,6α,12β,20(S)- tetrol(PPT), respectively (Shibata et al. 1995). Ginsenosides, which are named as ginsenoside Rx (x = o, a1, a2, b1, b2, b3, c, d, e, f, g1, g2, h1, and h2), differ from one another by the type of aglycone, sugar moieties, number of sugars, and their site of attachment (Figure 8.1). Exceptionally, ginsenoside Ro is an oleanane-type saponin, which is common in plants (Figure 8.1c). Another oleanane-type of ginsenoside is polyacetylene ginsenoside Ro, which contains a polyacetylenyl ester at the C-6′ position of glucosyl moiety (Zhang et al. 2002). These ginsenosides are usually extracted through water/n-butanol partitioning, following the extraction of ginseng root with aqueous alcohol, resulting in n-butanol extract as a saponin fraction.
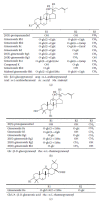
FIGURE 8.1
(a) Protopanaxadiol-type ginsenoside. (b) Protopanaxatriol-type ginsenoside. (c) Oleanane-type ginsenoside.
The structural diversity of ginsenosides may contribute to the multiple pharmacological effects of ginseng on cancer, diabetes, inflammation, stress, immune, cardiovascular system, and CNS. Furthermore, it is of interest that the coexistence of PPD- and PPT-type ginsenosides in ginseng may be associated with its dual effects that can both stimulate and sedate the CNS. Ginsenoside Rb1 has been observed to exhibit depressant activity on the CNS, whereas ginsenoside Rg1 showed stimulant activity (Takagi, Saito, and Nabata 1972). Microinjection of Rb1 into the hypothalamic ventromedial nucleus decreased food intake, indicating CNS-suppressive action (Etou et al. 1988). In contrast, the sustained central administration of ginsenoside Rg1 attenuated anorexia, increased water intake, and decreased ambulation produced by an increase in environmental temperature (Fujimoto et al. 1989).
8.2.1. Structural Conversion of Ginsenosides
Ginsenosides undergo structural conversion under high temperature conditions, such as the decoction and steaming of ginseng, and in the acidic conditions in the stomach or due to metabolism by the intestinal bacteria. Some partially deglycosylated saponins, such as ginsenoside Rh1, Rg2, and Rg3, are obtained as byproducts produced during steaming of red ginseng (Figure 8.1a, b). Ginsenoside Rh1 is generated from the ginsenoside Rg1 via deglycosylation at the C-20 position, whereas ginsenoside Rg2 is generated from ginsenoside Re. Ginsenoside Rg3 is further converted to ginsenoside Rh2 via deglycosylation of one terminal glucose at the C-3 position. Elimination of the sugar moiety and subsequent epimerization of the hydroxyl group at the C-20 position yields 20(R)-ginsenosides Rh1, 20(R)-ginsenosides Rg2, and 20(R)-ginsenosides Rg3 as epimers (Figure 8.1a, b).
Stereoisomers often differ considerably in pharmacological activity, potency, and pharmacokinetic profile, exerting different effects in biological systems. For example, 20(S)-Rg3 enhanced glucose-stimulated insulin secretion, whereas 20(R)-Rg3 did not show any effect (Park, Ha, and Chung 2008). Malonyl groups at the 6″-position of glucosyl moiety of ginsenoside Rb1, Rb2, Rc, and Rd are released during steaming, resulting in their corresponding ginsenosides (Kitagawa et al. 1983; Figure 8.1a). In red ginseng, the acetyl group remains at the 6″-position of glucosyl moiety of some saponins such as ginsenosides Rs1 and Rs2; thus, it appears that steaming inactivates the deacetylating enzyme (Kasai et al. 1983; Figure 8.1a).
The chemical structure of the side chain at the C-20 position can be modified by hydration or dehydration during steaming or decoction of the ginseng root. Recently, new dammarane glycosides with modified side chains, which are named ginsenoside Rh4, Rg5, Rg6, 20(E)-ginsenoside F4, and Rf2, have been isolated from Korean red ginseng (KRG; Park, Rhee, and Lee 2005). Further information may be found in a review by Christensen (2009). The structural conversion of ginsenosides also takes place in the gastrointestinal tract by gastric juice and digestive and bacterial enzymes after ingestion. The sugars attached to the C-3 or C-20 hydroxyl group of the aglycone are cleaved off separately from the end by intestinal flora (Hasegawa et al. 1996). PPD-type saponins are metabolized to compound K (C-K), whereas PPT-type saponins are hydrolyzed to 20(S)-PPT (Figure 8.1a, b). Pharmacological activities of C-K such as anticancer, antidiabetes, and anti-inflammation effects will be discussed in Sections 8.3 and 8.4.
8.2.2. Other Constituents in Ginseng
Ginseng contains several valuable nonsaponin components, including essential oils, antioxidants, polyacetylenic alcohols, peptides, amino acids, polysaccharides, and vitamins. Ginseng polysaccharides have also been a target of chemical and biological research, because plant polysaccharides generally show antitumor effects through modulation of innate immunity. Two acidic polysaccharides, which are named ginsenan S-IA and ginsenan S-IIA, were isolated from P. ginseng (Tomoda et al. 1993). Ginsenan S-IIA was shown to increase phagocytosis. Many immunological studies have been performed with crude polysaccharide fractions, which are usually prepared by ethanol precipitation after the extracting ginseng root with hot water. Their immunological activities will be described in Section 8.3.
Enormous progress has been made in understanding the chemistry of ginsenosides in transformed or metabolized forms as well as intact ones, contributing to the understanding of ginseng pharmacological properties. However, further studies on nonsaponin constituents, especially immunomodulating polysaccharides, and on the interaction and/or harmonization of constituents still remain to be explored.
8.3. IMMUNOMODULATORY FUNCTION OF GINSENG
There have been many reports describing the immunomodulating effects of ginseng, although results are somewhat controversial and inconsistent, since the chemical composition of purified fractions of ginseng employed in studies is different. In Sections 8.3.1 through 8.3.3, we describe the immunomodulating effects of aqueous extracts, saponin fractions, and polysaccharide fractions of ginseng.
8.3.1. Immunomodulating Effects of Aqueous Extracts of Ginseng
Aqueous extracts of ginseng contain amino acids, minerals, saponins, and various water-soluble low- and high-molecular weight compounds. It was reported that a ginseng extract modulated the cytokine production in a mouse model with Pseudomonas aeruginosa lung infection. The lung cells from the ginseng extract-treated group produced more interferon γ (IFN-γ) and tumor necrosis factor α (TNF-α), but less interleukin 4 (IL-4), with a higher ratio of IFN-γ/IL-4. Results indicated that a ginseng extract treatment induced a Th1-like immune response (cellular immune response) in the mice with Panax aeruginosa lung infection (Song et al. 2003).
Long-term oral administration of the ginseng extract appears to potentiate humoral immune response but suppresses spleen cell functions in male BALB/c mice. Mice treated with ginseng extract and immunized with ovalbumin (OVA) resulted in an eightfold increase in titers of anti-OVA immunoglobulin G (IgG) in serum, but IgG production was not affected in spleen cells (Liou, Huang, and Tseng 2005). Intranasal coadministration with inactivated influenza virus A (PR8) and ginseng extract increased the levels of influenza virus-specific antibodies and neutralizing activities and provided protective immunity compared to immunization with PR8 alone. Ginseng extract coadministration also significantly induced high levels of IL-4 and IL-5 cytokines, producing cells after PR8 infection, implying that ginseng extract plays a role as a mucosal adjuvant against influenza virus as well as an immunomodulator during influenza virus infection (Quan et al. 2007).
8.3.2. Immunomodulating Effects of Saponin Fraction
Dendritic cells (DCs) play a pivotal role in the initiation of T-cell-mediated immune responses, making them an attractive cellular adjuvant for use in cancer vaccines. Researchers investigated whether M4, end products of steroidal ginseng saponins metabolized in digestive tracts, can drive DCs maturation from human monocytes in vitro. Results showed that mature DCs differentiated with M4 induced the differentiation of naive T cells toward a helper T-cell type 1 (Th1) response and augmented cytotoxicity toward tumor cells. Takei et al. (2004) suggested that M4 might be used on DC-based vaccines for cancer immunotherapy.
In the case of ginsenoside Rg1, it was reported that Rg1 enhanced CD4(+) T-cell activities and modulated Th1/Th2 differentiation in murine splenocytes. Rg1 had no mitogenic effects on unstimulated CD4(+) T cells but augmented CD4(+) T-cell proliferation on activation with anti-CD3/anti-CD28 antibodies in a dose-dependent manner. Rg1 also enhanced the expression of cell surface protein CD69 on CD4(+) T cells. In Th0 condition, Rg1 increased the expression of IL-2 mRNA and enhanced the expression of IL-4 mRNA on CD4(+) T cells, suggesting that Rg1 prefers to induce Th2 lineage development (Lee et al. 2004). In addition, ginsenoside Rg1 induced Th1 type differentiation of CD4(+) T cells and helped mice resist disseminated candidiasis. Antimouse IFN-γ antibody treatment of Rg1-treated mice abolished the protection against disseminated candidiasis (Lee and Han 2006).
PPD saponins (Rg3, Rd, Rc, Rb1, and Rb2) and PPT saponins (Rg1, Re, and Rg2) were evaluated for their adjuvant effects on the immune responses to OVA in BALB/c mice. OVA-specific antibody responses were significantly higher in mice immunized with OVA coadministered with Rg1, Re, Rg2, Rg3, and Rb1, but not with Rd, Rc, and Rb2. Therefore, it is suggested that Rg1, Re, Rg2, Rg3, and Rb1 have more potent adjuvant effects than the others (Sun, Hu, and Song 2007). Recently, it has been reported that ginsenoside-based nanoparticles (ginsomes) played a role as a novel adjuvant and upregulated Th1 and Th2 immune response in imprinting control region (ICR) mice. The ginsomes were spherical with diameters ranging from 70 to 107 nm and contained ginsenosides Rb2, Rc, Rb1, and Rd. The ginsomes promoted significantly higher IgG responses, increased the levels of specific IgG1, IgG2a, IgG2b, and IgG3, as well as T and B lymphocyte proliferation in response to concanavalin A, LPS, and OVA. The enhanced IgG titer and subclass levels paralleled the increased production of IFN-γ (Th1 cytokine) and IL-5 (Th2 cytokine). Therefore, ginsomes as an adjuvant are assumed to upregulate both Th1 and Th2 immune responses (Song, Zang, and Hu 2009).
8.3.3. Immunomodulating Effects of Polysaccharide Fractions
Polysaccharide fractions of ginseng are high-molecular weight compounds obtained from the water-soluble and ethanol-insoluble fractions of ginseng. The in vitro immunostimulating activities of polysaccharides from ginseng were investigated. Four polysaccharides, which were found to be homogeneous by gel-filtration chromatography, were prepared and designated PF3111, PF3112, PBGA11, and PBGA12. Component sugar analysis revealed that they were heteroglycans with molecular weights ranging from 37 to 760 kD, composed of glucose, galactose, arabinose, mannose, and xylose in different molar ratios. Fraction PBGA12 had the most anticomplementary activity, which is mediated through both alternative and classical pathways. All the polysaccharides except PBGA11 induced the production of IFN-γ in the presence of concanavalin A. They induced the production of significant amount of TNF-α in cell cultures (Gao et al. 1996).
Incubation of murine macrophages (RAW 264.7 cells) with increasing amounts of polysaccharide fraction of ginseng showed a dose-dependent stimulation of inducible nitric oxide (NO) synthesis. This was associated with an incline in inducible nitric oxide synthase (NOS) mRNA levels as determined by semiquantitative polymerase chain reaction, and electromobility shift assay studies indicated enhanced nuclear factor κB (NF-κB) DNA binding activity. Friedl et al. (2001) suggested that polysaccharide treatment could modulate several aspects of host defense mechanisms due to stimulation of the inducible NOS. It was also reported that a polysaccharide fraction of ginseng stimulated murine normal splenocytes by inducing the mRNA expressions of Th1- and Th2-type cytokines and also restored the mRNA expression of IFN-γ, Th1 cytokine, after its inhibition by whole-body γ irradiation. Therefore, the polysaccharide fraction of ginseng was found to restore the T lymphocytes function that had been suppressed by γ irradiation in allogeneic mixed lymphocyte reactions (Han et al. 2005).
More recently, reports on the acidic polysaccharide of P. ginseng (APG) were described. Acidic polysaccharide fractions altered the phenotype of bone marrow cells (BMCs) and increased the viability and alloreactivity of BMCs after γ irradiation both in vitro and in vivo. A pretreatment with APG significantly increased the viability of BMCs against γ irradiation. APG-treated BMCs had a significantly higher amount of IL-12, which is a major cytokine for immune responses, compared with the medium-treated BMCs. The expression of major histocompatibility complex (MHC) class II molecules of APG-treated BMCs was also increased, and APG-treated BMCs showed significantly higher levels of allogeneic CD4(+) T lymphocyte proliferation. Furthermore, APG-treated mice had a larger number of BMCs after γ irradiation than the control mice, and the BMCs of APG-treated mice were successfully cultured into DCs, which are the representative antigen-presenting cells (Kim, Kim et al. 2007).
Various aspects of immunomodulatory effects of ginseng have been investigated for their tonic effects. Modulation of cytokine production, potentiation of humoral immune response, enhancement of CD4(+) T-cell activities, upregulation of adjuvant effects, restoration of T lymphocytes function, and BMCs viability after suppression by γ irradiation were especially remarkable.
8.4. ANTICARCINOGENIC FUNCTION OF GINSENG
The main weapons in the war against cancer have been early detection and surgical removal of the tumor, radiotherapy, and chemotherapy. There are also attempts to develop gene therapy. However, the results have been less than ideal, and strategy is now changing from therapeutic approaches to prevention of cancer by identifying effective natural products as chemopreventive agents. One of the promising candidates for cancer prevention is ginseng. People who consume ginseng preparations are at lower risk of cancers in the stomach, lung, liver, pancreas, ovaries, colon, and oral cavity (Yun 2003). P. ginseng, P. quinquefolius, and other related plants including Panax japonicus are frequently used for medicinal purposes. Although a complex mixture of compounds is present in these plants, the ginsenosides are mostly responsible for the pharmacological effects of these ginsengs, and Rg3 and Rh2 are recognized as major active anticancer saponins (Helms 2004). In Sections 8.4.1–8.4.5 describe the anticarcinogenic effects of ginseng based on its diverse mechanisms, including cell cytotoxicity and differentiation, antitumor promotion related to inflammation, antimetastasis and inhibition of angiogenesis, synergistic effect on chemical therapeutic agents, and decreasing multidrug resistance (MDR).
8.4.1. Effect on Tumor Cell Cytotoxicity and Differentiation
Saponin and nonsaponin compounds have been reported to show cytotoxic activities against various kinds of cancer cell lines in culture. The major active components are ginsenoside Rh2, a peculiar component of KRG, polyacetylenes, panaxydol, panaxynol, and panaxytriol. Jia et al. (2004) demonstrated that ginsenoside Rh2 inhibited proliferation, induced apoptosis in cancer cell lines, and sensitized drug-resistance breast cancer cells to paclitaxel. Recently, it was shown that KRG extract induced apoptosis and decreased telomerase activity in human leukemia cells (Park et al. 2009). The main active ingredients in KRG are four representative ginsenosides, Rg1, Rg3, Rh2, and Rk1. Ginsenosides Rg3 and Rh2 inhibited proliferation of prostate cancer cells by detachment of the cells and by modulating mitogen-activated protein (MAP) kinases (Kim, Lee et al. 2004). Ginsenoside Rh2 treatment significantly inhibited the viability of MCF-7 and MDA-MB-231 breast cancer cells with G0/G1 phase cell-cycle arrest, which was caused by p15Ink4B and p27Kip1-dependent inhibition of cyclin-dependent kinases (Choi, Kim, and Singh 2009). In addition, Rh2 markedly increased albumin secretion and alkaline phosphatase activity, whereas it strikingly decreased α-fetoprotein secretion and γ-glutamyl transpeptidase in SMMC-7721 hepatocarcinoma (Zeng and Tu 2003). Furthermore, Rh2 almost completely inhibited telomerase activity with the parallel induction of the cell differentiation. After steam or heat treatment of American ginseng and notoginseng, the content of Rg3 was found to increase remarkably, with increased antiproliferation of colorectal cancer cells (Wang and Yuan in press). In addition, acetylpanaxydol and panaxydolchlorohydrin, showing cytotoxicity against lymphoid leukemia L1210, have been isolated from Korean ginseng root (Ahn, Kim, and Lee 1989). Panaxydol, a polyacetylene compound isolated from Panax notoginseng, and P. ginseng inhibited proliferation and induced differentiation of the human hepatocarcinoma cell line HepG2 by increasing the expression of p21 and pRb, while reducing that of inhibitor of differentiation 1 and 2 (Guo et al. 2009). These studies suggest that ginseng compounds, such as ginsenoside Rh2 and panaxydol, block cancer cell proliferation and induce cell differentiation toward more mature forms of normal cells.
8.4.2. Antitumor Promotion Related to Inflammation
Considerable effort has been made to develop chemopreventive agents that could inhibit, retard, or reverse multistage carcinogenesis (Weinstein 1991). Tumor promotion is closely related to inflammation (DiGiovanni 1992), and compounds with strong anti-inflammatory activity possess antitumor promoter activity. Treatment with KRG extract of human leukemia cells decreased the expression levels of cyclo-oxygenase-2 (COX-2) and inducible NOS (Park et al. 2009), which are indicators of inflammation related to tumor promotion. In addition, treatment with KRG extract induced apoptosis of leukemia cells mediated by an inhibition of Bcl-2 and Bcl-XL, and it progressively downregulated the expression of human telomerase reverse transcriptase by inhibiting the expression of c-Myc. Ginsenosides Rb1, Rc, Re, Rg1, and Rg3 from P. ginseng were tested for anti-inflammatory activity (Surh et al. 2002). Rg3 was found to be the most effective in terms of inhibiting 12-0-tetradecanoylphorbol-13-acetate (TPA)-induced ear edema, COX-2 expression, and NF-κB activation. One ginsenoside metabolite, 20-0-β-D-glucopyranosyl-20(S)-PPD, which is known as C-K, given to ICR mice suppressed COX-2 expression and ornithine decarboxylase activity induced by TPA (Lee et al. 2005).
The eukaryotic transcriptional factor NF-κB is involved in intracellular signaling pathways associated with inflammation and carcinogenesis. C-K pretreatment inhibited TPA-induced epidermal NF-κB activity in mouse skin. Antitumor promotional effects of C-K were shown by markedly decreased numbers of papillomas in mouse skin induced by 12-dimethylbenz[a]anthracene (DMBA). These findings suggest that C-K exerts anti-inflammatory effects by inhibiting TPA-induced COX-2 expression, which may contribute to its antitumor-promoting effects on mouse skin carcinogenesis. Ginsenoside Rb1 inhibited histamine release and IL-4 production induced by substance P, an allergic enhancer, via the extracellular receptor kinase (ERK) pathway (Liao et al. 2006). It was also shown that C-K as a functional ligand of the glucocorticoid receptor regulated distinct Toll-like receptor 4-mediated inflammatory responses, which suggests a novel therapy for gram-negative septic shock (Yang et al. 2008).
8.4.3. Antimetastatic Effecr and Inhibition of Angiogenesis
Ginsenoside Rg3 inhibited tumor invasion and metastasis of F16 melanoma cells without impairing cell growth and proliferation of tumor cells (Mochizuki, Yoo, and Matsuzawa 1995). Rg3 inhibited the metastasis of ovarian cancer; the inhibitory effect is partially due to inhibition of tumor-induced angiogenesis and the decreased invasive ability and MMP-9 expression of SKOV-3 cells (Xu et al. 2008). Ginsenoside Rg3 significantly inhibited growth and angiogenesis of ovarian cancer when used alone or combined with cyclophosphamide (CTX; Xu et al. 2007). Another study found that low-dose CTX combined with Rg3 produced significant antiangiogenic effects, without overt toxicity, because Rg3 is capable of specific blockade of activated endothelial cell survival mechanisms (Zhang, Kang, and Zhoa 2006). These studies indicated that a ginsenoside Rg3 and CTX combination reinforced the antitumor effect on each other and improved the living quality and survival time of mice with tumors. As an antiangiogenic method, this regimen has the advantage of a lowered susceptibility to drug-resistance mechanisms and improved animal survival. Another ginsenoside, Rb1, suppressed the formation of endothelial tube-like structures through modulation of pigment epithelium-derived factors through estrogen β receptors (Leung et al. 2007). These findings demonstrated several novel mechanisms of these ginsenosides that may have value in anticancer and antiangiogenesis therapy. Ginsenoside 20(S)-PPD inhibited the proliferation and invasion of human fibrosarcoma HT1080 cells due to downregulation of the expression MMP-2 (Li et al. 2006). A ginseng saponin metabolite (C-K) suppressed phorbol ester-induced MMP-9 expression through inhibition of AP-1 and MAP kinase signaling pathways in human astroglioma cells (Jung et al. 2006). Ginsenoside Rp1, a semisynthesized ginseng saponin, strongly inhibited metastatic lung transfer of B16-melanoma cells by downregulation of β1-intergrin activation and further directly blocked the viability of cancer cells (Park, Park et al. 2008).
8.4.4. Anticarcinogenic Activities and Synergistic Effect in Combination with Chemical Therapeutic Agents
Several studies have been conducted to evaluate the inhibitory effect of ginseng on carcinogenesis induced by various chemical carcinogens. Earlier studies showed that long-term oral administration of KRG extract inhibited the incidence and the proliferation of tumors induced by 7,12-DMBA, urethane, and aflatoxin B1 (Yun, Yun, and Han 1983). The chemopreventive potential of ginseng was evaluated using DMBA-induced skin tumorigenesis (Kumar 1993). There was a marked reduction not only in tumor incidence but also in cumulative tumor frequency at the initiation phase of tumorigenesis. Ginsenosides Rg3 and Rg5 showed statistically significant reduction of lung cancer, and Rh2 tended to decrease the incidence (Yun et al. 2001). Panwar et al. (2005) showed that P. ginseng extract inhibited lung adenoma induced by benzo[a]pyrene and decreased the frequencies of chromosomal aberrations and micronuclei. Another study showed that Rh2 had an antiproliferative effect on human lung adenocarcinoma A549 cells with G1 arrest by downregulation of cyclin proteins and kinases and further apoptosis mediated by caspase-8 (Cheng et al. 2005). Dietary administration of KRG suppressed colon carcinogenesis induced by 1, 2-dimethylhydrazine with inhibition of cell proliferation, acting on aberrant crypt foci in the colon mucosa (Fukushima, Wanibuchi, and Li 2001). In addition, an anticarcinogenic effect of KRG on the development of liver cancer induced by diethylnitrosamine in rats was identified in preventive and curative events (Wu, Zhu, and Li 2001). Ginsenoside Rh2 was shown to inhibit cell growth at low concentrations, to induce apoptosis at high concentrations, and, interestingly, to act either additively or synergistically with chemotherapeutic drugs on cancer cells, especially breast cancer cells to paclitaxel (Jia et al. 2004). Panaxadiol (PD) enhanced the anticancer effects of 5-fluorouracil (5-FU) in human colorectal cancer cells by inducing apoptosis (Li et al. 2009). The enhancement of S-phase arrest and the increased susceptibility to apoptosis are synergistic effects of PD on 5-FU.
8.4.5. Reducing Multidrug Resistance
One of the major obstacles to the effective treatment of human malignancy is the acquisition of broad anticancer drug resistance by tumor cells. This phenomenon is called multidrug resistance. MDR is a major problem in cancer chemotherapy, and it is correlated with the overexpression of P-glycoprotein (Pgp) in the plasma membrane of resistant cells (Gotteeman and Pastan 1993). Ginsenoside Rg1, Re, Rc, and Rd were found to have a moderate inhibitory effect on the drug efflux pump in MDR mouse lymphoma and to increase intracellular drug accumulation (Molnar et al. 2000). Ginsenoside Rg3, among several ginseng components, was shown to have the most potent inhibitory activity on MDR human fibroblast carcinoma KBV20C (Park et al. 1996). Rg3 treatment of drug-resistant KBV20C cells specifically inhibited Pgp-mediated drug accumulation and further increased life span in mice implanted with adriamycin-resistant murine leukemia P388 cells in vivo (Kim et al. 2003). Subsequent studies demonstrated that Rg3 was cytotoxic against a multidrug-resistant human fibrocarcinoma KBV20C cells but not against normal WI cells in vitro, and Rg3 also promoted the accumulation of rhodamine 123 in adriamycin-resistant murine leukemia P388 cells in vivo by mediating decreased membrane fluidity, thereby blocking drug efflux (Kwon et al. 2008). Another study showed that ginsenoside metabolites Rh2, PPD, and PPT significantly enhanced the cytotoxicity of mitoxantrone (MX) to human breast carcinoma and may be potential inhibitors of breast cancer resistance protein (BCRP) in MCF-7/MX cells, which overexpress BCRP (Jin et al. 2006).
The protective influence and the complementary therapeutic potential of ginseng for cancer treatment have been shown by extensive laboratory, preclinical, and epidemiological studies. Two Korean cohort studies have suggested that ginseng consumers are associated with a 60-70% reduction in the risk of gastric cancer. However, ginseng consumers in the Shanghai women’s cohort study showed no beneficial effects on gastric cancer risk (Kamangar et al. 2007). Further careful evaluation in Asian cohort studies may help clarify ginseng’s effect on gastric carcinogenesis and other cancers. Additional clinical studies are needed to evaluate the potential beneficial effects of ginseng on chemoprevention and complementary therapy of cancers.
8.5. REDUCTION OF BLOOD GLUCOSE LEVEL AND IMPROVEMENT OF DIABETES TREATMENT
Diabetes, affecting almost 3.5% of the world’s population, is one of the major global health problems. The root of P. ginseng has been used to treat diabetes and has been given as a tonic for chronic use without adverse effects. More than 90% of patients with diabetes have type 2 diabetes, which is related to aging, low physical activity, diet, and lifestyle. In this section, we focus the effects of ginseng on type 2 diabetes rather than type 1 diabetes.
Animal studies support that the roots of P. ginseng and other ginseng species, including American ginseng, possess antihyperglycemic activity (Kimura et al. 1981; Chung and Choi 2001; Dey et al. 2003). It has been documented that ginseng therapy decreased fasting glucose, lowered body weight (Sotaniemi, Haapakoski, and Rautio 1995), and increased glucose utilization and insulin regulation in diabetic patients (Vulksan et al. 2008). Furthermore, American ginseng has the ability to attenuate postprandial glycemia in healthy individuals (Vulksan et al. 2001). Recently, it was observed that oral administration of P. ginseng root had the ability to improve insulin resistance in rats receiving a fructose-rich diet (Liu, Liu, and Cheng 2005). These observations suggest that ginseng is beneficial for patients with type 2 diabetes and for nondiabetic subjects to prevent development of diabetes.
8.5.1. Modulation of Insulin Secretion
Ginseng might mediate its antidiabetic action through a variety of mechanisms, including actions on the insulin-secreting pancreatic β-cells and the target tissues that take up glucose (Xie, Mehendale, and Yuan 2005). Korean white ginseng (KWG) and KRG, one of the heat-processed Korean ginsengs, have a long history as herbal remedies with antidiabetic effects. KWG has been reported to stimulate glucose-induced insulin release from pancreatic islets as a potentiator (Kimura et al. 1981; Su, Cheng, and Li 2007). The mode of the insulinotropic action of KRG was to act as an initiator for insulin release, not in a glucose-dependent manner. In general, the heat-processed KRG has been reported to have more potent pharmacological activities than nonprocessed KWG (Kim et al. 2000). KRG significantly evoked a stimulation of insulin release in normal pancreatic rat islets and may act by inhibiting the KATP channel, thereby depolarizing the β-cells and stimulating Ca2+ influx (Kim and Kim 2008). These findings suggest that P. ginseng has beneficial effects in the treatment of diabetes at least in part via the stimulation of insulin release.
Antihyperglycemic and antiobese effects of P. ginseng berry extract have been observed; its major constituent is ginsenoside Re (Attele et al. 2002). Ginsenoside Rg3 enhanced glucose-stimulated insulin secretion (Park, Ha, and Chung 2008) and was further metabolized to ginsenoside Rh2 by human intestinal bacteria, which seems to be more effective (Bae et al. 2002). Intravenous injection of ginsenoside Rh2 into rats decreased plasma glucose and increased plasma insulin by activation of muscarinic M3 receptors in pancreatic β-cells via acetylcholine (ACH) release from cholinergic terminals (Lee, Kao et al. 2006). PPD ginsenoside potentiated an insulin secretion stimulated by a low concentration of glucose, and C-K, a final metabolite of PPD ginsenoside, showed the most potent insulin secretion in pancreatic β-cells through action on the KATP-channeldependent pathway. These observations were confirmed in an oral glucose tolerance test in ICR mice (Han et al. 2007). In db/db mice, multiple administration of C-K showed hypoglycemic effects and improved glucose tolerance with β-cell preservation. Both Rh2 and C-K appear to have some therapeutic value for the treatment of diabetes and might be useful candidates for the development of new antidiabetic drugs.
8.5.2. Control of Blood Glucose Level and Glucose Transport
There are numerous reports of ginseng root improving diabetic conditions in both human and animal studies. In animal studies, orally administrated ginseng root was able to counteract the effect of high fructose-induced insulin resistance in rats after 4 weeks, decreasing glucose concentration and inhibiting insulin resistance (Liu, Liu, and Cheng 2005). Ethanol extract of wild ginseng root prevented weight gain and elevated fasting blood glucose, triglycerides, and high free fatty acid levels in a high fat-induced hyperglycemia mouse model (Yun et al. 2004). Ginsenoside Re decreased blood glucose, cholesterol, and triglyceride levels as well as decreased oxidative stress in the eye and kidney of diabetic rats (Cho et al. 2006). It is suggested that ginseng is useful for the prevention of diabetes in healthy people and for improved glycemic control in type 2 diabetes patients (Luo and Luo 2008). Clinical studies have reported that American ginseng lowers blood glucose in diabetic patients (Vulksan et al. 2000; Luo, Yano, and Luo 2003). In these studies, both type 2 diabetic patients and nondiabetic subjects were shown to benefit from intake of American ginseng in terms of stabilizing postprandial glycemia. More studies are required to confirm that ginseng administration decreases the dietary glycemic index, an indicator of carbohydrate’s ability to raise blood glucose level.
P. ginseng has been shown to increase glucose transport-2 protein in the liver of normal and hyperglycemic mice (Lee 1992). Recently, Shang et al. (2008) showed that ginsenoside Rb1 stimulated basal and insulin-mediated glucose uptake in a time- and dose-dependent manner in 3T3-L1 adipocytes and C2C12 myotubes. In adipocytes, Rb1 promoted GLUT1 and GLUT4 translocation to the cell membrane and further increased the phosphorylation of insulin receptor substrate-1, protein kinase B, and stimulated phosphatidylinositol 3(P13)-kinase activity in the absence of the activation of the insulin receptor. Ginsenoside Rg3 enhanced glucose-stimulated insulin secretion and AMP-activated protein kinase (AMPK) in HIT-T15 cells, and further lowered the plasma glucose level by stimulating insulin secretion in ICR mice associated with ATP-sensitive K+ channels (Park, Ha, and Chung 2008). AMPK is considered a master switch, regulating glucose and lipid metabolism, and an enzyme that works as a fuel gauge that is activated in conditions of high-energy phosphate depletion. Collectively, the findings provide insight into the hypoglycemic and antidiabetic properties of ginseng and ginsenosides and their potential to provide beneficial treatment for diabetes.
8.5.3. Regulation of Adipogenic Transcriptional Factor PPAR-γ and AMPK
Obesity is a major obstacle to human health because it predisposes individuals to various diseases, such as type 2 diabetes, cardiovascular diseases, and cancer. Two major proteins regulate adipocyte differentiation: AMPK and the peroxisome proliferator-activated receptor (PPAR; Yin, Mu, and Birnbaum 2003; Zhang, Lavan, and Greggore 2004). Both AMPK and PPAR-γ are major regulatory proteins involved in both obesity and diabetes. PPAR-γ is activated under conditions of adipocyte differentiation (Nedergaard et al. 2005; Lehrke and Lazar 2005). AMPK plays a role in intracellular energy homeostasis. The AMPK signaling pathway is induced by genistein, epigallocatechin gallate, and capsaicin and by decreasing 3T3-L1 adipocyte differentiation (Hwang et al. 2005). Ginsenoside Rh2 effectively inhibited adipocyte differentiation via PPAR-γ inhibition and activated AMPK in 3T3 L1 adipocytes (Hwang et al. 2007). Another study showed that ginsenoside Rb1 and Rg1 suppress triglycerides accumulation in 3T3-L1 adipocytes by activating PKA with increased intracellular cAMP (Park, Ahn et al. 2008). However, the insulin-stimulated glucose uptake was enhanced by Rb1 and Rg1 via activation of P13-kinase, and these ginsenosides promoted glucose-stimulated insulin secretion and cell viability in Min6 cells through PKA, which was associated with insulin response substrate 2 expression to insulin and insulin-like growth factor 1 signaling.
Some ginsenosides in ginseng improve insulin resistance by decreasing intracellular triglycerides accumulation. Ginsenoside Rb1 reduces rat liver triglycerides (Park et al. 2002) and Rh2 decreases triglyceride accumulation through AMPK activation in 3T3-L1 adipocytes (Hwang et al. 2007). Ginsenoside Rg3 was effective in inhibiting 3T3-L1 adipocyte differentiation through PPAR-γ induction by rosiglitazone and also was effective in activating AMPK (Hwang et al. 2009). The antiobesity effects of ginseng and ginsenosides Rg3, Rh2, and Rb1 may involve the AMPK and PPAR-γ signaling pathways. Further studies on the connection between the AMPK and PPAR-γ signaling pathways may be desirable to understand the antiobesity qualities of ginseng and its use in antidiabetic treatment.
8.6. EFFECTS OF GINSENG ON CENTRAL NERVOUS SYSTEM FUNCTIONS AND DEGENERATIVE DISEASES
Learning is the acquisition and storage of information as a consequence of experience, and memory is the relatively permanent storage form of the learned information, although it is not a single, unitary phenomenon. Alzheimer’s disease is the predominant age-related neurodegenerative disorder, and it is known mainly for its progressive memory loss and consequent dementia in the elderly. Ginsenoside, the active principle in P. ginseng root, has been demonstrated to show both neurotrophic effects in memory and learning and neuroprotective actions for the prevention of neuron degeneration.
8.6.1. Learning and Memory
Various memory-impairment models have been used to evaluate the effects of ginseng and its active ingredients on learning and memory. In passive avoidance test, ginsenoside Rg1 improved learning and memory acquisition, consolidation, and retrieval, indicating that Rg1 can improve all stages of memory (Zhang et al. 1990). To study the effect of ginsenoside Rg1 on learning and memory loss induced by β-amyloid, passive avoidance and performance in the Morris water maze were assayed after the final treatment. Ginsenoside Rg1 significantly decreased latency and swimming distance, improved corresponding changes in search strategies in the Morris water maze, and increased step-through latency (Wang and Zhang 2001). In another study, Rg1 significantly improved memory deficits in aged rats, ovariectomized rats, and cerebral ischemia-reperfusion rats (Qiu et al. 1995; Chen, Gong, and Zhang 2001). Results showed that ginseng extract and ginsenosides Rg1 and Rb1 facilitated acquisition and retrieval of memory. Moreover, these ginsenosides also antagonized memory loss and cognitive deficit under various pathological conditions, such as cerebral ischemia and dementia (Qiu et al. 1995).
Among the mechanisms underlying the positive impact on brain aging related to impairment of cognitive function and memory, ginsenosides might potentiate the cholinergic system in CNS. ACH is a very important neurotransmitter in the brain, and its scarcity often leads to learning and memory impairment. Ginsenosides Rg1 and Rb1 were found to enhance the functions of the cholinergic system by increasing the density of central M-cholinergic receptors and increasing the level of ACH in the CNS (Zhang et al. 1988). Glutamate, another neurotransmitter, is also important for learning, memory, and cognitive function. Ginsenosides Rb1 and Rg1 facilitate the release of glutamate evoked by 4-aminopyridine, a potassium channel blocker that depolarizes nerve terminals in vitro (Chang et al. 2008), in a manner corresponding to in vivo depolarization (Tibbs et al. 1989). Ginsenosides Rb1 and Rg1 mediated facilitations of glutamate release are associated with an enhancement of vesicular exocytosis, an increase in Ca2+ influx through presynaptic N- and P/Q-type voltage-dependent Ca2+ channels and protein kinase A, which subsequently enhances Ca2+ entry to cause an increase in evoked glutamate release from rat cortical synaptosomes (Chang et al. 2008). Further study of this group showed that ginsenosides Rb1 and Rg1 enhanced glutamate exocytosis from rat cortical nerve terminals by affecting vesicle mobilization through the activation of protein kinase C (Chang and Wang 2008).
8.6.2. Neurodegenerative Diseases
Apoptosis is a process by which a cell actively commits suicide under tightly controlled circumstances, and it plays a fundamental role in the development of multicellular organisms, maintenance of homeostasis, and numerous pathophysiological processes. However, defective control of apoptosis might play a role in the etiology of cancer, autoimmune diseases, and neurodegenerative disorders. It was first reported that ginsenoside Rg1 inhibited apoptosis induced by withdrawing serum from the culture system of primary cortical neurons (Li, Zhang, and Zhang 1997). An antiapoptotic effect of Rg1 was shown in aged rats in vivo. Further studies demonstrated that mechanisms of Rg1 on apoptosis involved decreasing NO content and NOS activity, reducing intracellular calcium concentration and enhancing superoxide dismutase activity. Li et al. (1997) found that both NOS expression and the activity of NOS were elevated significantly in aged rats, which leads to increased NO concentration in rat cortex. NO played a role in the acceleration of senescence, and the inhibitory effect of Rg1 on NOS activity may be related to its antiaging function. Other studies on the antiapoptotic effect of Rg1 on neurons suggest that the effect of Rg1 may contribute to enhancing the ratio of Bcl-2 to Bax protein and inhibiting activation of caspase-3 (Chen et al. 2002).
Among 11 ginsenosides (Rb1, Rb2, Rc, Rd, Re, Rf, Rg1, Rg2, Rg3, Rh1, and Rh2), Rg3 was the most effective ginsenoside in terms of inhibitory activity of N-methyl-D-aspartic acid (NMDA) on hippocampal neurons (Kim, Kim et al. 2004). Selective blockers of the active glycine site on NMDA receptors are considered to be promising therapeutics that may decrease the devastating effects of excitotoxicity (Lee, Zipfel, and Choi 1999). It was demonstrated that ginsenoside Rg3 significantly protects neurons from NMDA-induced neurotoxicity by blocking the glycine-binding site. Homocysteine could exert its excitotoxicity through NMDA receptor activation. It was shown that ginsenoside Rg3 significantly and dose-dependently inhibits homocysteine-induced hippocampal cell death. Ginsenoside Rg3 not only significantly lowered homocysteine-induced DNA damage, but also in vitro attenuated caspase-3 activity in a dose-dependent manner (Kim, Cho et al. 2007). Furthermore, it was also demonstrated that Rg3 dose-dependently inhibits homocysteine-induced increase of intracellular Ca2+ levels. In addition, ginsenoside Rg3 dose-dependently inhibited homocysteine-induced currents in Xenopus oocytes expressing NMDA receptors (Kim, Cho et al. 2007). These results collectively suggest that ginsenoside Rg3 protects from homocysteine-induced neurotoxicity in rat hippocampus; this effect is likely to be due to inhibition of homocysteine-mediated activation of NMDA receptors.
Ginsenoside Rh2 was identified as an active ingredient of ginseng that can act at the hippocampal NMDA receptors (Lee, Kim et al. 2006), but its neuroprotective activity came from an in vivo experiment showing that at 100 mg/kg p.o., ginsenoside Rh2 protects brain from ischemia-reperfusion injury (Park et al. 2004). These results indicate that ginsenoside Rg3 protects neurons in vitro from NMDA-induced neurotoxicity and in vivo ginsenoside Rh2 protects from ischemia-reperfusion brain injury. The neuroprotective activity of these ginsenosides can be attributed to the specific inhibition of NMDA-induced receptor activation.
Alzheimer’s disease, characterized microscopically by the deposition of amyloid plaques and formation of neurofibrillary tangles in the brain, has become the most common cause of senile dementia. Loss of cholinergic neurons along with muscarinic ACH receptors in the cerebral cortex and hippocampus is closely associated with Alzheimer’s disease. It was reported that Rg3 effectively decreased inflammatory cytokine expression in Abeta42-treated murine BV-2 microglial cells, inhibited the binding of NF-κB p65 to its DNA consensus sequences, and significantly decreased expression of TNF-α in activated microglia. Results suggest that inhibition of the inflammatory repertoire of microglia, neuroprotection, and increased macrophage scavenger receptor type A expression induced by Rg3 may at least partly explain its therapeutic effects in chronic neurodegenerative diseases (Joo et al. 2008). In addition, the effect of ginsenoside Rg3 on the metabolism of Abeta40 and Abeta42 was investigated in SK-N-SH cells transfected with Swedish mutant β-amyloid precursor protein (Yang et al. 2009). The results of enzyme-linked immunosorbent assay (ELISA) and Western blot analysis showed that Rg3 significantly lowered levels of Abeta40 and Abeta42, leading to the suggestion that Rg3 would be useful for treating patients suffering from Alzheimer’s disease (Yang et al. 2009).
Pituitary adenylate cyclase-activating polypeptide (PACAP) is introduced as a neurotrophic factor to promote cell survival. Ginsenoside Rh2 stimulated PACAP gene expression and cell proliferation in type 1 rat brain astrocytes (RBA1) cells and ameliorated the RBA1 growth inhibition of Abeta. These results suggested that Rh2 can induce an increase in PACAP to activate PAC1 and thereby lead to attenuating Abeta-induced toxicity (Shieh et al. 2008). Thus, it is suggested that ginseng is useful in the prevention of age-related neurodegenerative diseases such as dementia.
To summarize this section, ginsenosides Rg1, Rb1, Rg3, and Rh2 were shown to be effective in potentiating learning and memory acquisition, enhancing releases of ACH and glutamate, inhibiting apoptosis, and protecting neurons from neurotoxic insults. Ginsenosides Rg3 and Rh2 were particularly effective in protecting CNS, preventing neurodegenerative diseases, and might also be useful in the treatment of Alzheimer’s disease.
8.7. SUMMARY AND RESEARCH NEEDS
Ginseng has been widely used as a folk medicine in East Asian countries for thousands of years, mainly as a general tonic and adaptogen to maintain the body’s resistance to adverse factors and homeostasis, including improving physical and sexual function, general vitality, and antiaging. Ginseng and ginsenosides seem to be beneficial for immunity, cancer, diabetes, CNS functions, and other conditions. Although a single ginsenoside is demonstrated to be beneficial regarding some effects or conditions, it remains to be determined whether a single component or mixtures of components derived from ginseng can maximize benefit across several diseases and conditions. Therefore, more research works concerning the structure-activity relationship between ginseng constituents, acting individually or synergistically in a mixture, are required for predicting and ensuring physiological and pharmacological efficacy. In addition, as many steps must be taken to standardize the usage of ginseng root through isolating specific ginsenosides, the formulated standardization of ginseng extract and ginsenoside isolation is clearly required to have constant results and desirable efficacy in animal and human experiments. Finally, large-scale, controlled clinical studies are needed to validate the results in terms of their applicability to humans to extend those reported experiments that have been performed using animal models.
REFERENCES
- Ahn B. Z, Kim S. I, Lee Y. H. Acetyl panaxydol and panxydolchlorohydrin, two new polyenes from Korean ginseng with cytotoxic activity against L1210 cells. Arch Pharm. 1989;322:223–6. [PubMed: 2751411]
- Attele A. S, Zhou Y. P, Xie J. T, editors. et al. Antidiabetic effects of Panax ginseng berry extract and the identificationof an effective component. Diabetes. 2002;51:1851–8. [PubMed: 12031973]
- Bae E. A, Han M. J, Choo M. K, Park S. Y, Kim D. H. Metabolism of 20(S)- and 20(R)-gensenoside Rg3 by human intestinal bacteria and its relation to in vivo biological activities. Biol Pharm Bull. 2002;25:58–63. [PubMed: 11824558]
- Chang Y, Huang W. J, Tien L. T, Wang S. J. Ginsenosides Rg1 and Rb1 enhance glutamate release through activation of protein kinase A in rat cerebrocortical nerve terminals (synaptosomes). Eur J Pharmacol. 2008;578:28–36. [PubMed: 17949708]
- Chang Y, Wang S. J. Ginsenosides Rg1 and Rb1 enhance glutamate exocytosis from rat corticalnerve terminals by affecting vesicle mobilization through the activation of protein kinase C. Eur J Pharmacol. 2008;590:74–9. [PubMed: 18571158]
- Chen X. C, Chen Y, Zhu Y. G, Fang F, Chen L. M. Protective effect of ginsenoside Rg1 against MPTP-induced apoptosisin mouse substantia nigra neurons. Acta Pharmacol Sin. 2002;23:829–34. [PubMed: 12230953]
- Chen J, Gong Y. S, Zhang J. T. Effects of 17 estradiol and total ginsenoside on the spatial learning and memory impairment of ovariectomy rats. Chin Pharm J. 2001;36:522–6.
- Cheng C. C, Yang S. M, Huang C. Y, Chen J. C, Chang W. H, Hsu S. L. Molecular mechanisms of ginsenoside Rh2-mediated G1 growth arrest and apoptosis in human lung adenocarcinoma A549 cells. Cancer Chemother Pharmacol. 2005;55:531–40. [PubMed: 15739095]
- Cho W. C, Chung W. S, Lee S. K, Leung A. W, Cheng C. H, Yue K. K. Ginsenoside Re of Panax ginseng possesses significant antioxidant and antihyperlipidemic efficiency in streptozotocin-induced diabetic rats. Eur J Pharmacol. 2006;550:173–9. [PubMed: 17027742]
- Choi S, Kim T. W, Singh S. V. Ginsenoside Rh2-mediated G1 phase cell cycle arrest in human breast cancer cells is caused by p15Ink4B and p27 Kip1-dependent inhibition of cyclin-dependent kinases. Pharm Res. 2009;26(10):2280–8. [PMC free article: PMC2745112] [PubMed: 19629651]
- Christensen L. P. Ginsenosides: Chemistry, biosynthesis, analysis, and potential health effects. Adv Food Nutr Res. 2009;55:1–99. [PubMed: 18772102]
- Chung S. H, Choi C. G. Comparisons between white ginseng radix and rootlet for antidiabetic mechanism in KKAy mice. Arch Pharm Res. 2001;24:214–8. [PubMed: 11440080]
- Dey L, Xie J. T, Wang A, Wu J, Maleckar S. A, Yuan C. S. Anti-hyperglycemic effects of ginseng: Comparison between root and berry. Phytomedicine. 2003;10:600–5. [PubMed: 13678250]
- DiGiovanni J. Multistage carcinogenesis in mouse skin. Pharmacol Ther. 1992;54:63–128. [PubMed: 1528955]
- Etou H, Sakata T, Fujimoto K, editors. et al. Ginsenoside Rb1 as a suppressor in central modulation of feeding in the rat. Nippon Yakurigaku Zasshi. 1988;91(1):9–15. [PubMed: 3286417]
- Friedl R, Moeslinger T, Kopp B, Spieckermann P. G. Stimulation of nitric oxide synthesis by the aqueous extract of Panax ginseng root in RAW 264.7 cells. Br J Pharmacol. 2001;134:1663–70. [PMC free article: PMC1572905] [PubMed: 11739242]
- Fujimoto K, Sakata T, Ishimaru T, editors. Attenuation of anorexia induced by heat or surgery during sustained administration of ginsenoside Rg1 into rat third ventricle. Psychopharmacology. 1989;99(2):257–60. [PubMed: 2508164]
- Fukushima S, Wanibuchi H, Li W. Inhibition of ginseng of colon carcinogenesis in rats. J Korean Med Sci. 2001 16:S75–80. [PMC free article: PMC3202205] [PubMed: 11748381]
- Gao H, Wang F, Lien E. J, Trousdale M. D. Immunostimulating polysaccharides from Panax notoginseng. Pharm Res. 1996;13:1196–200. [PubMed: 8865311]
- Gillis C. N. Panax ginseng pharmacology: A nitric oxide link? Biochem Pharmacol. 1997;54:1–8. [PubMed: 9296344]
- Gotteeman M. M, Pastan I. Biochemistry of multidrug resistance mediated by the multidrug trans-port. Annu Rev Biochem. 1993;62:385–427. [PubMed: 8102521]
- Guo L, Song L, Wang Z, Zhao W, Mao W, Ming Y. Panaxydol inhibits the proliferation and induces the differentiation of human hepatocarcinoma cell lineHepG2. Chem Biol Interact. 2009;181:138–43. [PubMed: 19450571]
- Han C. G, Ko S. K, Sung J. H, Chung S. H. Compound K enhances insulin secretion with beneficial metabolic effects in db/db mice. J Agric Food Chem. 2007;55:10641–8. [PubMed: 18034458]
- Han S. K, Song J. Y, Yun Y. S, Yi S. Y. Ginseng improved Th1 immune response inhibited by gamma radiation. Arch Pharm Res. 2005;28:343–50. [PubMed: 15832824]
- Hasegawa H, Sung J. H, Matsumiya S, Uchiyama M. Main ginseng saponin metabolitesformed by intestinal bacteria. Planta Med. 1996;62:453–7. [PubMed: 8923812]
- Helms S. Cancer prevention and therapeutics: Panax ginseng. Altern Med Rev. 2004;9:259–74. [PubMed: 15387718]
- Huang K. C. The Pharmacology of Chinese Herbs. Boca Raton, FL: CRC Press; 1999.
- Hwang J. T, Kim S. H, Lee M. S, editors. et al. Anti-obesity effects of ginsenoside Rh2 are associated with the activation of AMPK signaling pathway in 3T3-L1 adipocyte. Biochem Biophys Res Commun. 2007;364:1002–8. [PubMed: 17971295]
- Hwang J. T, Lee M. S, Kim H. J, editors. et al. Antiobesity effect of ginsenoside Rg3 involves the AMPK and PPAR-?signal pathways. PhytotherRes. 2009;23:262–6. [PubMed: 18844326]
- Hwang J. T, Park J. I, Shin Y. K, editors. et al. Genistein, EGCG, and capsaicin inhibit adipocyte differentiation process via activating AMP-activated protein kinase. Biochem Biophys Res Commun. 2005;338:694–9. [PubMed: 16236247]
- Jia W. W, Bu X, Philips D, editors. et al. Rh2, a compound extracted from ginseng, hypersensitizes multidrug-resistance tumor cells to chemotherapy. Can J Physiol Pharmacol. 2004;82:431–7. [PubMed: 15389289]
- Jin J, Shahi S, Kang H. K, Veen H.W. van, Fan T. P. Metabolite of ginsenosides as novel BCRP inhibitors. Biochem Biophys Res Commun. 2006;345:1308–14. [PubMed: 16729968]
- Joo S. S, Yoo Y. M, Ahn B. W, editors. Prevention of inflammation-mediated neurotoxicity by Rg3 and its role in microglial activation. Biol Pharm Bull. 2008;31:1392–6. [PubMed: 18591781]
- Jung N. P, Jin S. H. Studies on the physiological and biochemical effect of Korean ginseng. Korean J Ginseng Sci. 1996;20:431–71.
- Jung S. H, Woo M. S, Kim S. Y, editors. et al. Ginseng saponin metabolite suppresses phorbol ester-induced matrix mpproteinase-9 expression through inhibition of activator protein-1 and mitogen-activated protein kinase signaling pathway in human astroglioma cells. Int J Cancer. 2006;118:490–7. [PubMed: 16049964]
- Kamangar F, Gao Y. T, Shu X. O, editors. et al. Ginseng intake and gastric cancer risk in the Shanghai women's health study cohort. Cancer Epidemiol Biomarkers Prev. 2007;16:629–630. [PubMed: 17372265]
- Kasai R, Besso H, Tanaka O, Saruwatari Y. I, Mizutare T. Saponins of red ginseng. Chem Pharm Bull. 1983;31:2120–5.
- Kim J. H, Cho S. Y, Lee J. H, editors. et al. Neuroprotective effects of ginsenoside Rg3 against homocysteine-induced excitotoxicity in rat hippocampus. Brain Res. 2007;1136:190–9. [PubMed: 17239831]
- Kim K, Kim H. Y. Korean red ginseng stimulates insulin release from isolated rat pancreatic islets. J Ethnopharmacol. 2008;120:190–5. [PubMed: 18773949]
- Kim S, Kim T, Ahn K, Park W. K, Nah S. Y, Rhim H. Ginsenoside Rg3 antagonizes NMDA receptors through a glycine modulatory site in rat cultured hippocampal neurons. Biochem Biophys Res Commun. 2004;323:416–24. [PubMed: 15369768]
- Kim H. J, Kim M. H, Byon Y. Y, Park J. W, Jee Y, Joo H. G. Radioprotective effects of an acidic polysaccharide of Panax ginseng on bone marrow cells. J Vet Sci. 2007;8:39–44. [PMC free article: PMC2872695] [PubMed: 17322772]
- Kim W. Y, Kim J. M, Han S. B, editors. et al. Steaming of ginseng at high temperature enhances biological activity. J Nat Prod. 2000;63:1702–4. [PubMed: 11141123]
- Kim S. W, Kwon H. Y, Chi D. W, editors. et al. Reversal of P-glycoprotein-mediated multidrug resistance by ginsenoside Rg(3). Biochem Pharmacol. 2003;65:58–61. [PubMed: 12473381]
- Kim H. S, Lee E. H, Ko S. R, Choi K. J, Park J. H, Im D. S. Effects of ginsenosides Rg3 and Rh2 on the proliferation of prostate cancer cells. Arch Pharm Res. 2004;27:429–35. [PubMed: 15180309]
- Kimura M, Waki I, Chujo T, editors. et al. Effects of hypoglycemic components in ginseng radix on blood insu-linlevel in alloxan diabetic mice and on insulin release from perfused rat pancreas. J Pharmacobiodyn. 1981;4:410–7. [PubMed: 7026762]
- Kitagawa I, Yoshikawa M, Yoshihara M, Hayashi T, Taniyama T. Chemical studies on crude drug processing I. On the constituents of Ginseng Radix Rubra. Yakugaku Zasshi. 1983;103:612–22. [PubMed: 6655550]
- Kumar A. Chemopreventive action of ginseng on DMBA-induced papillomagenesis in the skin of mice. Proceedings of the 6th International Ginseng Symposium. 1993:66–8. Seoul, Korea.
- Kwon H. Y, Kim E. H, Kim S. W, Kim S. N, Park J. D, Rhee D. K. Selective toxicity of ginsenoside Rg3 on multidrug resistance cells by membrane fluidity modulation. Arch Pharm Res. 2008;31:171–7. [PubMed: 18365686]
- Lee F. C. About Ginseng: The Elixir of Life. Elizabeth, NJ: Hollin International Corp; 1992.
- Lee J. H, Han Y. Ginsenoside Rg1 helps mice resist to disseminated candidiasis by Th1 type dif-ferentiation of CD4+ T cell. Int Immunopharmacol. 2006;6:1424–30. [PubMed: 16846836]
- Lee W. K, Kao S. T, Liu I. M, Cheng J. T. Increase of insulin secretion by ginsenoside Rh2 to lower plasma glucose in Wistar rats. Clin Exp Pharmcol Physiol. 2006;33:27–32. [PubMed: 16445695]
- Lee E, Kim S, Chun K. C, editors. et al. 20(S)-ginsenoside Rh2, a newly identified active ingredient of ginseng, inhibits NMDA receptors in cultured rat hippocampal neurons. Eur J Pharmacol. 2006;536:69–77. [PubMed: 16563373]
- Lee E. J, Ko E, Lee J, editors. et al. Ginsenoside Rg1 enhances CD4(+) T-cell activities and modulates Th1/Th2 differentiation. Int Immunopharmacol. 2004;4:235–44. [PubMed: 14996415]
- Lee J. Y, Shin J. W, Chun K. S, editors. et al. Antitumor promotional effects of a novel intestinal bacterial metabolite (IH-901) derived from the protopanaxadiol-type ginsenosides in mouse skin. Carcinogenesis. 2005;26:359–67. [PubMed: 15498788]
- Lee J. M, Zipfel G. J, Choi D. W. The changing landscape of ischaemic brain injury mechanisms. Nature. 1999;399:A7–14. [PubMed: 10392575]
- Lehrke M, Lazar M. A. The many faces of PPARgamma. Cell. 2005;123:993–9. [PubMed: 16360030]
- Leung K. W, Cheung L. W. T, Pon Y. L, editors. et al. Ginsenoside Rb1 inhibits tube-like structure formation of endothelial cells by regulating pigment epithelium-derived factor through the oestrogen ?receptor. British J Pharm. 2007;152:207–15. [PMC free article: PMC1978254] [PubMed: 17603552]
- Li J. Q, Li Z. K, Duan H, Zhang J. T. Effect of age and ginsenoside Rg1 on nitric oxide content and nitric oxide synthase activity of cerebral cortex on rats. Acta Pharm Sin. 1997;32:251–4. [PubMed: 11499025]
- Li X. L, Wang C. Z, Mehendale S. R, Sun S, Wang Q, Yuan C. S. Panaxadiol, a purified ginseng component, enhances the anti-cancer effects of 5-fluorouracil in human colorectal cancer cells. Cancer Chemother Pharmacol. 2009;64(6):1097–104. [PubMed: 19277659]
- Li G, Wang Z, Sun Y, Liu K, Wang Z. Ginsenoside 20(S)-protopanaxadiol inhibits the proliferation and invasion of human fibrosarcoma HT1080 cells. Basic Clin Pharmacol Toxicol. 2006;98:588–92. [PubMed: 16700822]
- Li J, Zhang G, Zhang J. T. Inhibition of apoptosis by ginsenoside Rg1 in cultured cortical neurons. Acta Pharm Sin. 1997;32:406–10. [PubMed: 11596321]
- Liao B. C, Hou R. C, Wang J. S, Jeng K. C. Enhancement of the release of inflammatory mediators by substance P in rat basophilic leukemia RBL-2H3 cells. J Biomed Sci. 2006;13:613–9. [PubMed: 16847722]
- Liou C. J, Huang W. C, Tseng J. Long-term oral administration of ginseng extract modulates humoral immune response and spleen cell functions. Am J Chin Med. 2005;33:651–61. [PubMed: 16173538]
- Liu T. P, Liu I. M, Cheng J. T. Improvement of insulin resistance by Panax ginseng in fructose-rich chow-fed rats. Horm Metab Res. 2005;37:333–9. [PubMed: 15824968]
- Luo J. Z, Luo L. Ginseng on hyperglycemia: Effects and mechanisms. eCAM. 2008;6:423–7. [PMC free article: PMC2781779] [PubMed: 18955300]
- Luo J. Z, Yano N, Luo L. American ginseng stimulates insulin production and prevents apoptosis induced by IL-1 in pancreatic ?cells. Diabetes. 2003;52 1:A354–1534-P.
- Mochizuki M, Yoo Y. C, Matsuzawa K. Inhibitory effect of tumor metastasis in mice by saponins, ginsenoside Rb2, 20(R)- and 20(S)-ginsenoside-Rg3, of red ginseng. Biol Pharm Bull. 1995;18:1197–202. [PubMed: 8845804]
- Molnar J, Szabo D, Pusztal R, editors. et al. Membrane associated antitumor effects of crocine-, ginsenoside-and cannabinoid derivatives. Anticancer Res. 2000;20:861–7. [PubMed: 10810367]
- Nedergaard J, Petrovic N, Lindgren E. M, editors. et al. PPARgamma in the control of brown adipocyte differentiation. Biochem Biophys Acta. 2005;1740:293–304. [PubMed: 15949696]
- Panwar M, Samarth R, Kumar M, Yoon W. J, Kumar A. Inhibition of benzo(a)pyrene induced lung adenoma by Panax ginseng extract, EFLA400, in Swiss albino mice. Biol Pharm Bull. 2005;28:2063–7. [PubMed: 16272690]
- Park S, Ahn I. S, Kwon D. Y, Ko B. S, Jun W. K. Ginsenoside Rb1 and Rg1 suppress triglyceride accumulation in 3T3-L1 adipocytes and enhance ?-cell insulin secretion and viability in Min6 cells via PKA-dependent pathways. Biosci Biotechnol Biochem. 2008;72:2815–23. [PubMed: 18997435]
- Park E. K, Choo M. K, Oh J. K, Ryu J. H, Kim D. H. Ginsenoside Rh2 reduces ischemic brain injury in rat. Biol Pharm Bull. 2004;27:433–6. [PubMed: 14993818]
- Park M. W, Ha J, Chung S. H. 20(S)-ginsenoside Rg3 enhances glucose-stimulated insulin secretion and activates AMPK. Biol Pharm Bull. 2008;31(4):748–51. [PubMed: 18379076]
- Park J. D, Kim D. S, Son S. K, editors. et al. Effect of ginseng saponin on modulation of multidrug resistance. Arch Pharm Res. 1996;19:213–8.
- Park S. E, Park C, Kim S. H, editors. et al. Korean red ginseng extract induces apoptosis and decreases telomerase activity in human leukemia cells. J Ethnopharmacol. 2009;121:304–12. [PubMed: 19041934]
- Park T. Y, Park M. H, Shin W. C, editors. et al. Anti-metastatic potential of ginsenoside Rp1, a novel ginsenoside derivative. Biol Pharm Bull. 2008;31:1802–5. [PubMed: 18758081]
- Park J. D, Rhee D. K, Lee Y. H. Biological activities and chemistry of saponins from Panax ginseng C. A. Meyer. Phytochem Rev. 2005;4:159–75.
- Park K. H, Shin H. J, Song Y. B, editors. et al. Possible role of ginsenoside Rb1 onregulation of rat liver triglycerides. Biol Pharm Bull. 2002;25:457–60. [PubMed: 11995924]
- Qiu Y, Du G. H, Qu Z. W, Zhang J. T. Protective effects of ginsenoside on the learning and memory impairment induced by transient cerebral ischemia-reperfusion in mice. Chin Pharmacol Bull. 1995;11:299–302.
- Quan F. S, Compans R. W, Cho Y. K, Kang S. M. Ginseng and Salviae herbs play a role as immune activators and modulate immune responses during influenza virus infection. Vaccine. 2007;25:272–82. [PubMed: 16945454]
- Shang W, Yang Y, Zhou L, editors. et al. Ginsenoside Rb1 stimulates glucose uptake through insulin-like signaling pathway in 3T3-L1 adipocytes. J Endocrinol. 2008;198:561–9. [PubMed: 18550785]
- Shibata S, Tanaka O, Shoji J, Saito H. Chemistry and pharmacology of Panax ginseng. Wagner H, Kikino H, Farnsworth N. R New York: Academic Press; Economic and Medicinal Plant Research. 1995;vol. 1:217–84.
- Shieh P. C, Tsao C. W, Li J. S, editors. et al. Role of pituitary adenylate cyclase-activating polypeptide (PACAP) in the action of ginsenoside Rh2 against beta-amyloid-induced inhibition of rat brain astrocytes. Neurosci Lett. 2008;434:1–5. [PubMed: 18313848]
- Song Z, Moser C, Wu H, Faber V, Kharazmi A, HØiby N. Cytokine modulating effect of ginseng treatment in a mouse model of Pseudomonas aeruginosa lung infection. J Cyst Fibros. 2003;2:112–9. [PubMed: 15463859]
- Song X, Zang L, Hu S. Amplified immune response by ginsenoside-based nanoparticles (ginsomes). Vaccine. 2009;27:2306–11. [PubMed: 19428844]
- Sotaniemi E. A, Haapakoski E, Rautio A. Ginseng therapy in non-insulin dependent diabetic patients. Diabetes Care. 1995;18:1373–5. [PubMed: 8721940]
- Su C. F, Cheng J. T, Li I. M. Increase of acetylcholine release by Panax ginseng root enhances insulin secretion in Wistar rats. Neurosci Lett. 2007;412:101–4. [PubMed: 17123721]
- Sun J, Hu S, Song X. Adjuvant effects of protopanaxadiol and protopanaxatriol saponins from ginseng roots on the immune responses to ovalbumin in mice. Vaccine. 2007;25:1114–20. [PubMed: 17069940]
- Surh Y. J, Lee J. Y, Choi K. J, Ko S. R. Effects of selected ginsenosides on phorbol ester-induced expression of cyclooxygenase-2 and activation ofNF-kBand ERK1/2 in mouse skin. Ann N Y Acad Sci. 2002;973:396–401. [PubMed: 12485900]
- Takagi K, Saito H, Nabata H. Pharmacological studies of Panax ginseng root: Estimation of pharmacological actions of Panax ginseng root. Jpn J Pharmacol. 1972;22:245–9. [PubMed: 4538416]
- Takei M, Tachikawa E, Hasegawa H, Lee J. J. Dendritic cells maturation promoted by M1 and M4, end products of steroidal ginseng saponins metabolized in digestive tracts, drive a potent Th1 polarization. Biochem Pharmacol. 2004;68:441–52. [PubMed: 15242811]
- Tibbs G. R, Barrie A. P, Van Mieghem F. J, McMahon H. T, Nicholls D. G. Repetitive action potentials on isolated nerve terminals in the presence of 4-aminopyridine: Effects on cytosolic free Ca2+ and glutamate release. J Neurochem. 1989;53:1693–9. [PubMed: 2553862]
- Tomoda M, Hirabayashi K, Shimizu N, Gonda R, Ohara N, Takada K. Characterization of two novel polysaccharides having immunological activities from the root of Panax ginseng. Biol Pharm Bull. 1993;16(11):1087–90. [PubMed: 8312860]
- Vulksan V, Sievenpiper J. L, Koo V. Y, editors. et al. American ginseng (Panax quinquefolius L.) reduces postprandial glycemia in nondiabetic subjects with type 2 diabetes mellitus. Arch Intern Med. 2000;160:1009–13. [PubMed: 10761967]
- Vulksan V, Sievenpiper J, Wong J, editors. et al. American ginseng (Panax quinquefolius L.) attenuates postprandial glycemia in a time-dependent but not dose-dependent manner in healthy individuals. Am J Clin Nutr. 2001;73:753–8. [PubMed: 11273850]
- Vulksan V, Sung M. K, Sievenpiper J. L, editors. et al. Korean red ginseng (Panax ginseng) improves glucose and insulin regulation in well-controlled study of efficacy and safety. Nutr Metab Cardiovasc Dis. 2008;18:46–56. [PubMed: 16860976]
- Wang C. Z, Yuan C. S. Potential role of ginseng in the treatment of colorectal cancer. Am J Chin Med. 36:1019–28. [PMC free article: PMC2676850] [PubMed: 19051332]
- Wang X. Y, Zhang J. T. Effects of ginsenoside Rg1 on learning and memory impairment induced by ?-amyloid peptide (25-35) and its mechanism of action. Acta Pharm Sin. 2001;36:1–4. [PubMed: 12579850]
- Weinstein I. B. Cancer prevention: Recent progress and future opportunities. Cancer Res. 1991;51 (18 Suppl.:5080s–5s. [PubMed: 1884384]
- Wu X. G, Zhu D. H, Li W. Anticarcinogenic effect of red ginseng on the development of liver cancer induced by diethylnitrosamine in rats. J Korean Med Sci. 2001 16:S61–5. [PMC free article: PMC3202202] [PubMed: 11748378]
- Xie J. T, Mehendale S, Yuan C. S. Ginseng and diabetes. Am J Chin Med. 2005;33:397–404. [PubMed: 16047557]
- Xu T. M, Cui M. H, Jiang M, editors. et al. Inhibitory effect of ginsenoside Rg3 on ovarian cancer. Chin Med J. 2008;121:1394–7. [PubMed: 18959116]
- Xu T. M, Xin Y, Cui M. H, Jiang X, Gu L. Inhibitory effect of ginsenoside Rg3 combined with cyclophosphamide on growth and angiogenesis of ovarian cancer. Chin Med J. 2007;120:584–8. [PubMed: 17442207]
- Yang L, Hao J, Zhang J, editors. et al. Ginsenoside Rg3 promotes beta-amyloid peptide degradation by enhancing gene expression of neprilysin. J Pharm Pharmacol. 2009;61:375–80. [PubMed: 19222911]
- Yang C. S, Ko S. R, Cho B. G, editors. et al. The ginsenoside metabolite compound K, a novel agonist of glucocorticoid receptor induces tolerance to endotoxin-induced lethal shock. J Cell Mol Med. 2008;12:1739–53. [PMC free article: PMC3918090] [PubMed: 18053081]
- Yin W, Mu J, Birnbaum M. J. Role of AMP-activated protein kinase in cyclin AMP-dependent lipolysis in 3T3-L1 adipocytes. J Biol Chem. 2003;278:43074–80. [PubMed: 12941946]
- Yoshikawa M, Murakami T, Yashiro K, editors. et al. Bioactive saponins and glycosides, XI. Structure of new dammarane-type triterpene oligoglycosides, quinquenosides II, I, IV, III, and V, from American ginseng, the roots of Panax quinquefolium L. Chem Pharm Bull. 1998;46:647–54. Tokyo. [PubMed: 9579041]
- Yun T. K. Experimental and epidemiological evidence on non-organ specific cancer preventive effect of Korean ginseng and identification of active compounds. Mutat Res. 2003;523-524:63–74. [PubMed: 12628504]
- Yun T. K, Lee Y. S, Lee Y. H, Kim S. I, Yun H. Y. Anticarcinogenic effect of Panax ginseng C.A. Meyer and identification of active compounds. J Korean Med Sci. 2001 16:S6–18. [PMC free article: PMC3202204] [PubMed: 11748383]
- Yun S. N, Moon S. J, Ko S. K, editors. et al. Wild ginseng prevents the onset of high-fat diet induced hyper glycemia and obesity in ICR mice. Arch Pharm Res. 2004;27:790–6. [PubMed: 15357009]
- Yun T. K, Yun Y. S, Han I. W. Anticarcinogenic effect of long-term oral administration of red ginsengon new mice exposed to various chemical carcinogens. Cancer Detect Prev. 1983;6:515–25. [PubMed: 6420059]
- Zeng X. I, Tu Z. G. In vitro induction of differentiation by ginsenoside Rh2 in SMMC-7721 hepatocarcinoma cell line. Pharmcol Toxicol. 2003;93:275–83. [PubMed: 14675461]
- Zhang Q, Kang X, Zhao W. Antiangiogenic effect of low-dose cyclophosphamide combined with ginsenoside Rg3 on Lewis lungcarcinoma. Biochem Biophys Res Commun. 2006;342:824–8. [PubMed: 16499874]
- Zhang F, Lavan B, Greggore F. M. Peroxisome proliferator-activated receptors as attractive anti-obesity targets. Drug News Prespect. 2004;17:661–9. [PubMed: 15696231]
- Zhang J. T, Liu Y, Qu Z. W, Zhang X. L, Xiao H. L. Influence of ginsenoside Rb1 and Rg1 on some central neurotransmitter receptors and protein biosynthesis in mouse brain. Acta Pharm Sin. 1988;23:12–16. [PubMed: 2840797]
- Zhang H, Lu Z, Tan G. T, editors. et al. Polyacetyleneginsenoside-Ro, a novel triterpene saponin from Panax ginseng. Tetrahedron Lett. 2002;43:973–7.
- Zhang J. T, Qu Z. W, Liu Y, Deng H. L. Preliminary study on antiamnestic mechanism of ginsenoside Rg1 and Rb1. Chin Med J. 1990;103:932–8. [PubMed: 2177392]
- INTRODUCTION
- STRUCTURAL PROPERTIES OF GINSENG
- IMMUNOMODULATORY FUNCTION OF GINSENG
- ANTICARCINOGENIC FUNCTION OF GINSENG
- REDUCTION OF BLOOD GLUCOSE LEVEL AND IMPROVEMENT OF DIABETES TREATMENT
- EFFECTS OF GINSENG ON CENTRAL NERVOUS SYSTEM FUNCTIONS AND DEGENERATIVE DISEASES
- SUMMARY AND RESEARCH NEEDS
- REFERENCES
- Chemical Diversity of Panax ginseng, Panax quinquifolium, and Panax notoginseng.[J Ginseng Res. 2012]Chemical Diversity of Panax ginseng, Panax quinquifolium, and Panax notoginseng.Kim DH. J Ginseng Res. 2012 Jan; 36(1):1-15.
- Chemical and pharmacological studies of saponins with a focus on American ginseng.[J Ginseng Res. 2010]Chemical and pharmacological studies of saponins with a focus on American ginseng.Yuan CS, Wang CZ, Wicks SM, Qi LW. J Ginseng Res. 2010 Sep 1; 34(3):160-7.
- Red American ginseng: ginsenoside constituents and antiproliferative activities of heat-processed Panax quinquefolius roots.[Planta Med. 2007]Red American ginseng: ginsenoside constituents and antiproliferative activities of heat-processed Panax quinquefolius roots.Wang CZ, Aung HH, Ni M, Wu JA, Tong R, Wicks S, He TC, Yuan CS. Planta Med. 2007 Jun; 73(7):669-74. Epub 2007 May 31.
- Review Red ginseng and cancer treatment.[Chin J Nat Med. 2016]Review Red ginseng and cancer treatment.Wang CZ, Anderson S, DU W, He TC, Yuan CS. Chin J Nat Med. 2016 Jan; 14(1):7-16.
- Review Effects of Red and Fermented Ginseng and Ginsenosides on Allergic Disorders.[Biomolecules. 2020]Review Effects of Red and Fermented Ginseng and Ginsenosides on Allergic Disorders.Han MJ, Kim DH. Biomolecules. 2020 Apr 20; 10(4). Epub 2020 Apr 20.
- Biological Activities of Ginseng and Its Application to Human Health - Herbal Me...Biological Activities of Ginseng and Its Application to Human Health - Herbal Medicine
- LOC105372156 [Homo sapiens]LOC105372156 [Homo sapiens]Gene ID:105372156Gene
- Gm26562 predicted gene, 26562 [Mus musculus]Gm26562 predicted gene, 26562 [Mus musculus]Gene ID:105246095Gene
Your browsing activity is empty.
Activity recording is turned off.
See more...