NCBI Bookshelf. A service of the National Library of Medicine, National Institutes of Health.
Baron S, editor. Medical Microbiology. 4th edition. Galveston (TX): University of Texas Medical Branch at Galveston; 1996.
General Concepts
Clinical Manifestations
Staphylococci can cause many forms of infection. (1) S aureus causes superficial skin lesions (boils, styes) and localized abscesses in other sites. (2) S aureus causes deep-seated infections, such as osteomyelitis and endocarditis and more serious skin infections (furunculosis). (3) S aureus is a major cause of hospital acquired (nosocomial) infection of surgical wounds and, with S epidermidis, causes infections associated with indwelling medical devices. (4) S aureus causes food poisoning by releasing enterotoxins into food. (5) S aureus causes toxic shock syndrome by release of superantigens into the blood stream. (6) S saprophiticus causes urinary tract infections, especially in girls. (7) Other species of staphylococci (S lugdunensis, S haemolyticus, S warneri, S schleiferi, S intermedius) are infrequent pathogens.
Structure
Staphylococci are Gram-positive cocci 1μm in diameter. They form clumps.
Classification
S aureus and S intermedius are coagulase positive. All other staphylococci are coagulase negative. They are salt tolerant and often hemolytic. Identification requires biotype analysis.
Natural Habitat
S aureus colonizes the nasal passage and axillae. S epidermidis is a common human skin commensal. Other species of staphylococci are infrequent human commensals. Some are commensals of other animals.
Pathogenesis
S aureus expresses many potential virulence factors. (1) Surface proteins that promote colonization of host tissues. (2) Factors that probably inhibit phagocytosis (capsule, immunoglobulin binding protein A). (3) Toxins that damage host tissues and cause disease symptoms. Coagulase-negative staphylococci are normally less virulent and express fewer virulence factors. S epidermidis readily colonizes implanted devices.
Host Defenses
Phagocytosis is the major mechanism for combatting staphylococcal infection. Antibodies are produced which neutralize toxins and promote opsonization. The capsule and protein A may interfere with phagocytosis. Biofilm growth on implants is impervious to phagocytosis.
Treatment
Infections acquired outside hospitals can usually be treated with penicillinase-resistant β-lactams. Hospital acquired infection is often caused by antibiotic resistant strains and can only be treated with vancomycin.
Antibiotic Resistance
Multiple antibiotic resistance is increasingly common in S aureus and S epidermidis. Methicillin resistance is indicative of multiple resistance. Methicillin-resistant S aureus (MRSA) causes outbreaks in hospitals and can be epidemic.
Epidemiology
Epidemiological tracing of S aureus is traditionally performed by phage typing, but has limitations. Molecular typing methods are being tested experimentally.
Diagnosis
Diagnosis is based on performing tests with colonies. Tests for clumping factor, coagulase, hemolysins and thermostable deoxyribonuclease are routinely used to identify S aureus. Commercial latex agglutination tests are available. Identification of S epidermidis is confirmed by commercial biotyping kits.
Control
Patients and staff carrying epidemic strains, particularly MRSA, should be isolated. Patients may be given disinfectant baths or treated with a topical antibiotic to eradicate carriage of MRSA. Infection control programs are used in most hospitals.
Introduction
Bacteria in the genus Staphylococcus are pathogens of man and other mammals. Traditionally they were divided into two groups on the basis of their ability to clot blood plasma (the coagulase reaction). The coagulase-positive staphylococci constitute the most pathogenic species S aureus. The coagulase-negative staphylococci (CNS) are now known to comprise over 30 other species. The CNS are common commensals of skin, although some species can cause infections. It is now obvious that the division of staphylococci into coagulase positive and negative is artificial and indeed, misleading in some cases. Coagulase is a marker for S aureus but there is no direct evidence that it is a virulence factor. Also, some natural isolates of S aureus are defective in coagulase. Nevertheless, the term is still in widespread use among clinical microbiologists.
S aureus expresses a variety of extracellular proteins and polysaccharides, some of which are correlated with virulence. Virulence results from the combined effect of many factors expressed during infection. Antibodies will neutralize staphylococcal toxins and enzymes, but vaccines are not available. Both antibiotic treatment and surgical drainage are often necessary to cure abscesses, large boils and wound infections. Staphylococci are common causes of infections associated with indwelling medical devices. These are difficult to treat with antibiotics alone and often require removal of the device. Some strains that infect hospitalized patients are resistant to most of the antibiotics used to treat infections, vancomycin being the only remaining drug to which resistance has not developed.
Taxonomy
DNA-ribosomal RNA (rRNA) hybridization and comparative oligonucleotide analysis of 16S rRNA has demonstrated that staphylococci form a coherent group at the genus level. This group occurs within the broad Bacillus-Lactobacillus-Streptococcus cluster defining Gram-positive bacteria with a low G + C content of DNA.
At least 30 species of staphylococci have been recognized by biochemical analysis and in particular by DNA-DNA hybridization. Eleven of these can be isolated from humans as commensals. S aureus (nares) and S epidermidis (nares, skin) are common commensals and also have the greatest pathogenic potential. S saprophyticus (skin, occasionally) is also a common cause of urinary tract infection. S haemolyticus, S simulans, S cohnii, S warneri and S lugdunensis can also cause infections in man.
Identification of Staphylococci in the Clinical laboratory
Structure
Staphylococci are Gram-positive cocci about 0.5 – 1.0 μm in diameter. They grow in clusters, pairs and occasionally in short chains. The clusters arise because staphylococci divide in two planes. The configuration of the cocci helps to distinguish micrococci and staphylococci from streptococci, which usually grow in chains. Observations must be made on cultures grown in broth, because streptococci grown on solid medium may appear as clumps. Several fields should be examined before deciding whether clumps or chains are present.
Catalase Test
The catalase test is important in distinguishing streptococci (catalase-negative) staphylococci which are catalase positive. The test is performed by flooding an agar slant or broth culture with several drops of 3% hydrogen peroxide. Catalase-positive cultures bubble at once. The test should not be done on blood agar because blood itself will produce bubbles.
Isolation and Identification
The presence of staphylococci in a lesion might first be suspected after examination of a direct Gram stain. However, small numbers of bacteria in blood preclude microscopic examination and require culturing first.
The organism is isolated by streaking material from the clinical specimen (or from a blood culture) onto solid media such as blood agar, tryptic soy agar or heart infusion agar. Specimens likely to be contaminated with other microorganisms can be plated on mannitol salt agar containing 7.5% sodium chloride, which allows the halo-tolerant staphylococci to grow. Ideally a Gram stain of the colony should be performed and tests made for catalase and coagulase production, allowing the coagulase-positive S aureus to be identified quickly. Another very useful test for S aureus is the production of thermostable deoxyribonuclease. S aureus can be confirmed by testing colonies for agglutination with latex particles coated with immunoglobulin G and fibrinogen which bind protein A and the clumping factor, respectively, on the bacterial cell surface. These are available from commercial suppliers (e.g., Staphaurex). The most recent latex test (Pastaurex) incorporates monoclonal antibodies to serotype 5 and 8 capsular polysaccharide in order to reduce the number of false negatives. (Some recent clinical isolates of S aureus lack production of coagulase and/or clumping factor, which can make identification difficult.)
The association of S epidermidis (and to a lesser extent of other coagulase-negative staphylococci) with nosocomial infections associated with indwelling devices means that isolation of these bacteria from blood is likely to be important and not due to chance contamination, particularly if successive blood cultures are positive. Nowadays, identification of S epidermidis and other species of Staphylococcus is performed using commercial biotype identification kits, such as API Staph Ident, API Staph-Trac, Vitek GPI Card and Microscan Pos Combo. These comprise preformed strips containing test substrates.
Epidemiology of Staphylococcus Aureus Infections
Because S aureus is a major cause of nosocomial and community-acquired infections, it is necessary to determine the relatedness of isolates collected during the investigation of an outbreak. Typing systems must be reproducible, discriminatory, and easy to interpret and to use. The traditional method for typing S aureus is phage-typing. This method is based on a phenotypic marker with poor reproducibility. Also, it does not type many isolates (20% in a recent survey at the Center for Disease Control and Prevention), and it requires maintenance of a large number of phage stocks and propagating strains and consequently can be performed only by specialist reference laboratories.
Many molecular typing methods have been applied to the epidemiological analysis of S aureus, in particular, of methicillin-resistant strains (MRSA). Plasmid analysis has been used extensively with success, but suffers the disadvantage that plasmids can easily be lost and acquired and are thus inherently unreliable. Methods designed to recognize restriction fragment length polymorphisms (RFLP) using a variety of gene probes, including rRNA genes (ribotyping), have had limited success in the epidemiology of MRSA. In this technique the choice of restriction enzyme used to cleave the genomic DNA, as well as the probes, is crucial. Random primer PCR offers potential for discriminating between strains but a suitable primer has yet to be identified for S aureus. The method currently regarded as the most reliable is pulsed field gel electrophoresis, where genomic DNA is cut with a restriction enzyme that generates large fragments of 50-700 kb.
Clinical Manifestations of S Aureus
S aureus is notorious for causing boils, furuncles, styes, impetigo and other superficial skin infections in humans (Figure 12-1). It may also cause more serious infections, particularly in persons debilitated by chronic illness, traumatic injury, burns or immunosuppression. These infections include pneumonia, deep abscesses, osteomyelitis, endocarditis, phlebitis, mastitis and meningitis, and are often associated with hospitalized patients rather than healthy individuals in the community. S aureus and S epidermidis are common causes of infections associated with indwelling devices such as joint prostheses, cardiovascular devices and artificial heart valves (Fig. 12-2).
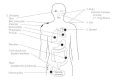
Figure 12-1
Pathogenesis of staphylococcal infections.
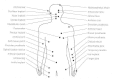
Figure 12-2
Infections associated with indwelling devices.
Pathogenesis of S Aureus Infections
S aureus expresses many cell surface-associated and extracellular proteins that are potential virulence factors. For the majority of diseases caused by this organism, pathogenesis is multifactorial. Thus it is difficult to determine precisely the role of any given factor. This also reflects the inadequacies of many animal models for staphylococcal diseases.
However, there are correlations between strains isolated from particular diseases and expression of particular factors, which suggests their importance in pathogenesis. With some toxins, symptoms of a human disease can be reproduced in animals with pure proteins. The application of molecular biology has led to recent advances in the understanding of pathogenesis of staphylococcal diseases. Genes encoding potential virulence factors have been cloned and sequenced and proteins purified. This has facilitated studies at the molecular level on their modes of action, both in in vitro and in model systems. In addition, genes encoding putative virulence factors have been inactivated, and the virulence of the mutants compared to the wild-type strain in animal models. Any diminution in virulence implicates the missing factor. If virulence is restored when the gene is returned to the mutant then “Molecular Koch's Postulates” have been fulfilled. Several virulence factors of S aureus have been confirmed by this approach.
Adherence
In order to initiate infection the pathogen must gain access to the host and attach to host cells or tissues.
S aureus Adheres to Host Proteins
S aureus cells express on their surface proteins that promote attachment to host proteins such as laminin and fibronectin that form part of the extracellular matrix (Figure 12-3). Fibronectin is present on epithelial and endothelial surfaces as well as being a component of blood clots. In addition, most strains express a fibrinogen/fibrin binding protein (the clumping factor) which promotes attachment to blood clots and traumatized tissue. Most strains of S aureus express fibronectin and fibrinogen-binding proteins.
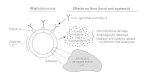
Figure 12-3
Summary of virulence factors of Staphylococcus aureus.
The receptor which promotes attachment to collagen is particularly associated with strains that cause osteomyelitis and septic arthritis. Interaction with collagen may also be important in promoting bacterial attachment to damaged tissue where the underlying layers have been exposed.
Evidence that these staphylococcal matrix-binding proteins are virulence factors has come from studying defective mutants in vitro adherence assays and in experimental infections. Mutants defective in binding to fibronectin and to fibrinogen have reduced virulence in a rat model for endocarditis, suggesting that bacterial attachment to the sterile vegetations caused by damaging the endothelial surface of the heart valve is promoted by fibronectin and fibrinogen. Similarly, mutants lacking the collagen-binding protein have reduced virulence in a mouse model for septic arthritis. Furthermore, the soluble ligand-binding domain of the fibrinogen, fibronectin and collagen-binding proteins expressed by recombinant methods strongly blocks interactions of bacterial cells with the corresponding host protein.
Role of Adherence in Infections Associated with Medical Devices
Infections associated with indwelling medical devices ranging from simple intravenous catheters to prosthetic joints and replacement heart valves can be caused by S aureus and S epidermidis (Figure 12-2). Very shortly after biomaterial is implanted in the human body it becomes coated with a complex mixture of host proteins and platelets. In one model system involving short-term contact between biomaterial and blood, fibrinogen was shown to be the dominant component and was primarily responsible for adherence of S aureus in subsequent in vitro assays. In contrast, with material that has been in the body for longer periods (e.g., human intravenous catheters) the fibrinogen is degraded and no longer promotes bacterial attachment. Instead, fibronectin, which remains intact, becomes the predominant ligand promoting attachment.
Adherence to Endothelial Cells
S aureus can adhere to the surface of cultured human endothelial cells and become internalized by a phagocytosis-like process. It is not clear if attachment involves a novel receptor or a known surface protein of S aureus. Some researchers think that S aureus can initiate endocarditis by attaching to the undamaged endothelium. Others feel that trauma of even a very minor nature is required to promote attachment of bacteria.
Avoidance of Host Defenses
S aureus expresses a number of factors that have the potential to interfere with host defense mechanisms. However, strong evidence for a role in virulence of these factors is lacking.
Capsular Polysaccharide
The majority of clinical isolates of S aureus express a surface polysaccharide of either serotype 5 or 8. This has been called a microcapsule because it can be visualized only by electron microscopy after antibody labeling, unlike the copious capsules of other bacteria which are visualized by light microscopy. S aureus isolated from infections expresses high levels of polysaccharide but rapidly loses it upon laboratory subculture. The function of the capsule is not clear. It may impede phagocytosis, but in in vitro tests this was only demonstrated in the absence of complement. Conversely, comparing wild-type and a capsule defective mutant strain in an endocarditis model suggested that polysaccharide expression actually impeded colonization of damaged heart valves, perhaps by masking adhesins.
Protein A
Protein A is a surface protein of S aureus which binds immunoglobulin G molecules by the Fc region (Fig. 12-3). In serum, bacteria will bind IgG molecules the wrong way round by this non-immune mechanism. In principle this will disrupt opsonization and phagocytosis. Indeed mutants of S aureus lacking protein A are more efficiently phagocytozed in vitro, and studies with mutants in infection models suggest that protein A enhances virulence.
Leukocidin
S aureus can express a toxin that specifically acts on polymorphonuclear leukocytes. Phagocytosis is an important defense against staphylococcal infection so leukocidin should be a virulence factor. This toxin is discussed in more detail in the next section.
Damage to the Host
S aureus can express several different types of protein toxins which are probably responsible for symptoms during infections. Some damage the membranes of erythrocytes, causing hemolysis; but it is unlikely that hemolysis is relevant in vivo. The leukocidin causes membrane damage to leukocytes and is not hemolytic. Systemic release of α-toxin causes septic shock, while enterotoxins and TSST-1 cause toxic shock.
Membrane Damaging Toxins
(a) α-toxin
The best characterized and most potent membrane-damaging toxin of S aureus is α-toxin. It is expressed as a monomer that binds to the membrane of susceptible cells. Subunits then oligomerize to form hexameric rings with a central pore through which cellular contents leak.
Susceptible cells have a specific receptor for α-toxin which allows low concentrations of toxin to bind, causing small pores through which monovalent cations can pass. At higher concentrations, the toxin reacts non-specifically with membrane lipids, causing larger pores through which divalent cations and small molecules can pass. However, it is doubtful if this is relevant under normal physiological conditions.
In humans, platelets and monocytes are particularly sensitive to α-toxin. They carry high affinity sites which allow toxin to bind at concentrations that are physiologically relevant. A complex series of secondary reactions ensue, causing release of eicosanoids and cytokines which trigger production of inflammatory mediators. These events cause the symptoms of septic shock that occur during severe infections caused by S aureus.
The notion that α-toxin is a major virulence factor of S aureus is supported by studies with the purified toxin in animals and in organ culture. Also, mutants lacking α-toxin are less virulent in a variety of animal infection models.
(b) β-toxin
β-toxin is a sphingomyelinase which damages membranes rich in this lipid. The classical test for β-toxin is lysis of sheep erythrocytes. The majority of human isolates of S aureus do not express β-toxin. A lysogenic bacteriophage is inserted into the gene that encodes the toxin. This phenomenon is called negative phage conversion. Some of the phages that inactivate the β-toxin gene carry the determinant for an enterotoxin and staphylokinase (see below).
In contrast the majority of isolates from bovine mastitis express β-toxin, suggesting that the toxin is important in the pathogenesis of mastitis. This is supported by the fact that β-toxin-deficient mutants have reduced virulence in a mouse model for mastitis.
(c) δ-toxin
The δ-toxin is a very small peptide toxin produced by most strains of S aureus. It is also produced by S epidermidis and S lugdunensis. The role of δ-toxin in disease is unknown.
(d) γ-toxin and leukocidin
The γ-toxin and the leukocidins are two-component protein toxins that damage membranes of susceptible cells. The proteins are expressed separately but act together to damage membranes. There is no evidence that they form multimers prior to insertion into membranes. The γ-toxin locus expresses three proteins. The B and C components form a leukotoxin with poor hemolytic activity, whereas the A and B components are hemolytic and weakly leukotoxic.
The classical Panton and Valentine (PV) leukocidin is distinct from the leukotoxin expressed by the γ-toxin locus. It has potent leukotoxicity and, in contrast to γ-toxin, is non-hemolytic. Only a small fraction of S aureus isolates (2% in one survey) express the PV leukocidin, whereas 90% of those isolated from severe dermonecrotic lesions express this toxin. This suggests that PV leukocidin is an important factor in necrotizing skin infections.
PV-leukocidin causes dermonecrosis when injected subcutaneously in rabbits. Furthermore, at a concentration below that causing membrane damage, the toxin releases inflammatory mediators from human neutrophils, leading to degranulation. This could account for the histology of dermonecrotic infections (vasodilation, infiltration and central necrosis).
Superantigens: enterotoxins and toxic shock syndrome toxin
S aureus can express two different types of toxin with superantigen activity, enterotoxins, of which there are six serotypes (A, B, C, D, E and G) and toxic shock syndrome toxin (TSST-1). Enterotoxins cause diarrhea and vomiting when ingested and are responsible for staphylococcal food poisoning. When expressed systemically, enterotoxins can cause toxic shock syndrome (TSS) - indeed enterotoxins B and C cause 50% of non-menstrual TSS. TSST-1 is very weakly related to enterotoxins and does not have emetic activity. TSST-1 is responsible for 75% of TSS, including all menstrual cases. TSS can occur as a sequel to any staphylococcal infection if an enterotoxin or TSST-1 is released systemically and the host lacks appropriate neutralizing antibodies. Tampon-associated TSS is not a true infection, being caused by growth of S aureus in a tampon and absorption of the toxin into the blood stream. TSS came to prominence with the introduction of super-absorbent tampons; and although the number of such cases has decreased dramatically, they still occur despite withdrawal of certain types of tampons from the market.
Superantigens stimulate T cells non-specifically without normal antigenic recognition (Figure 12-4). Up to one in five T cells may be activated, whereas only 1 in 10,000 are stimulated during antigen presentation. Cytokines are released in large amounts, causing the symptoms of TSS. Superantigens bind directly to class II major histocompatibility complexes of antigen-presenting cells outside the conventional antigen-binding grove. This complex recognizes only the Vβ element of the T cell receptor. Thus any T cell with the appropriate Vβ element can be stimulated, whereas normally antigen specificity is also required in binding.
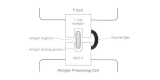
Figure 12-4
Superantigens and the non-specific stimulation of T cells.
Epidermolytic (exfoliative) toxin (ET)
This toxin causes the scalded skin syndrome in neonates, with widespread blistering and loss of the epidermis. There are two antigenically distinct forms of the toxin, ETA and ETB. There is evidence that these toxins have protease activity. Both toxins have a sequence similarity with the S aureus serine protease, and the three most important amino acids in the active site of the protease are conserved. Furthermore, changing the active site of serine to a glycine completely eliminated toxin activity. However, ETs do not have discernible proteolytic activity but they do have esterase activity. It is not clear how the latter causes epidermal splitting. It is possible that the toxins target a very specific protein which is involved in maintaining the integrity of the epidermis.
Other Extracellular Proteins
Coagulase
Coagulase is not an enzyme. It is an extracellular protein which binds to prothrombin in the host to form a complex called staphylothrombin. The protease activity characteristic of thrombin is activated in the complex, resulting in the conversion of fibrinogen to fibrin. This is the basis of the tube coagulase test, in which a clot is formed in plasma after incubation with the S aureus broth-culture supernatant. Coagulase is a traditional marker for identifying S aureus in the clinical microbiology laboratory. However, there is no evidence that it is a virulence factor, although it is reasonable to speculate that the bacteria could protect themselves from host defenses by causing localized clotting. Notably, coagulase deficient mutants have been tested in several infection models but no differences from the parent strain were observed.
There is some confusion in the literature concerning coagulase and clumping factor, the fibrinogen-binding determinant on the S aureus cell surface. This is partly due to loose terminology, with the clumping factor sometimes being referred to as bound coagulase. Also, although coagulase is regarded as an extracellular protein, a small fraction is tightly bound on the bacterial cell surface where it can react with prothrombin. Finally, it has recently been shown that the coagulase can bind fibrinogen as well as thrombin, at least when it is extracellular. Genetic studies have shown unequivocally that coagulase and clumping factor are distinct entities. Specific mutants lacking coagulase retain clumping factor activity, while clumping factor mutants express coagulase normally.
Staphylokinase
Many strains of S aureus express a plasminogen activator called staphylokinase. The genetic determinant is associated with lysogenic bacteriophages. A complex formed between staphylokinase and plasminogen activates plasmin-like proteolytic activity which causes dissolution of fibrin clots. The mechanism is identical to streptokinase, which is used in medicine to treat patients suffering from coronary thrombosis. As with coagulase there is no evidence that staphylokinase is a virulence factor, although it seems reasonable to imagine that localized fibrinolysis might aid in bacterial spreading.
Enzymes
S aureus can express proteases, a lipase, a deoxyribonuclease (DNase) and a fatty acid modifying enzyme (FAME). The first three probably provide nutrients for the bacteria, and it is unlikely that they have anything but a minor role in pathogenesis. However, the FAME enzyme may be important in abscesses, where it could modify anti-bacterial lipids and prolong bacterial survival. The thermostable DNase is an important diagnostic test for identification of S aureus.
Coagulase Negative Staphylococci
Staphylococci other than S aureus can cause infections in man. S epidermidis is the most important coagulase-negative staphylococcus (CNS) species and is the major cause of infections associated with prosthetic devices and catheters. CNS also cause peritonitis in patients receiving continuous ambulatory peritoneal dialysis and endocarditis in those with prosthetic valves. These infections are not usually nosocomially acquired. Other species such as S haemolyticus, S warneri, S hominis, S capitis, S intermedius, S schleiferi and S simulans are infrequent pathogens. S lugdunesis is a newly recognized species. It is probably more pathogenic than are other CNS species, with cases of endocarditis and other infections being reported. It is likely that the incidence of infections caused by these organisms is underestimated because of difficulties in identification.
Diagnosis of CNS infections is difficult. Infections are often indolent and chronic with few obvious symptoms. This is due to the smaller array of virulence factors and toxins compared to those in the case of S aureus. S epidermidis is a skin commensal and is one of the most common contaminants of samples sent to the diagnostic laboratory, while S lugdunensis is often confused with S aureus. Precise identification of CNS species requires the use of expensive test kits, such as the API-Staph.
In contrast to S aureus, little is known about mechanisms of pathogenesis of S epidermidis infections. Adherence is obviously a crucial step in the initiation of foreign body infections. Much research has been done on the interaction between S epidermidis and plastic material used in implants, and a polysaccharide adhesion (PS/A) has been identified. Mutants lacking PS/A are less virulent in an animal model for foreign body infection, and immunization with purified PS/A is protective. Bacteria-plastic interactions are probably important in colonization of catheters through the point of entry. However, host proteins are quickly deposited on implants. S epidermidis does not bind to fibrinogen but most isolates bind fibronectin, albeit less avidly than S aureus. However, it is not known if a protein analogous to the fibronectin binding protein of S aureus is involved.
A characteristic of clinical isolates of S epidermidis is the production of “slime.” This is a controversial topic. Some feel that slime is an in vitro manifestation of the ability to form a biofilm in vivo, for example on the surface of a prosthetic device, and is thus a virulence marker. In vitro, slime is formed during growth in broth as a biofilm on the surface of the growth vessel. The composition of this slime is probably influenced by the growth medium. One study with defined medium showed that the slime was predominantly secreted teichoic acid, a polymer normally found in the cell wall of staphylococci. Some polysaccharides in slime from bacteria grown on solid medium are derived from the agar.
Resistance of Staphylococci to Antimicrobial Drugs
Hospital strains of S aureus are often resistant to many different antibiotics. Indeed strains resistant to all clinically useful drugs, apart from the glycopeptides vancomycin and teicoplanin, have been described. The term MRSA refers to methicillin resistance and most methicillin-resistant strains are also multiply resistant. Plasmid-associated vancomycin resistance has been detected in some enterococci and the resistance determinant has been transferred from enterococci to S aureus in the laboratory and may occur naturally. S epidermidis nosocomial isolates are also often resistant to several antibiotics including methicillin. In addition, S aureus expresses resistance to antiseptics and disinfectants, such as quaternary ammonium compounds, which may aid its survival in the hospital environment.
Since the beginning of the antibiotic era S aureus has responded to the introduction of new drugs by rapidly acquiring resistance by a variety of genetic mechanisms including (1) acquisition of extrachromosomal plasmids or additional genetic information in the chromosome via transposons or other types of DNA insertion and (2) by mutations in chromosomal genes (Table 12-1).
Table 12-1
Antimicrobial Resistance.
Many plasmid-encoded determinants have recently become inserted into the chromosome at a site associated with the methicillin resistance determinant. There may be an advantage to the organism having resistance determinants in the chromosome because they will be more stable.There are essentially four mechanisms of resistance to antibiotics in bacteria: (1) enzymatic inactivation of the drug, (2) alterations to the drug target to prevent binding, (3) accelerated drug efflux to prevent toxic concentrations accumulating in the cell, and (4) a by-pass mechanism whereby an alternative drug-resistant version of the target is expressed (Table 12-1).
Future Prospects
Antimicrobial Drugs
Ever since the first use of penicillin, S aureus has shown a remarkable ability to adapt. Resistance has developed to new drugs within a short time of their introduction. Some strains are now resistant to most conventional antibiotics. It is worrisome that there do not seem to be any new antibiotics on the horizon. Any recent developments have been modifications to existing drugs.
The original strategy used by the pharmaceutical industry to find antimicrobial drugs was to screen natural products and synthetic chemicals for antimicrobial activity. The mechanism of action was then investigated.
New approaches are being adopted to find the next generation of antimicrobials. Potential targets such as enzymes involved in an essential function (e.g., in cell division) are identified based on knowledge of bacterial physiology and metabolism. Screening methods are then developed to identify inhibitors of a specific target molecule. In addition, with detailed molecular knowledge of the target molecule, specific inhibitors can be designed.
Vaccines and New Approaches to Combatting Nosocomial Infections
No vaccine is currently available to combat staphylococcal infections. There may now be a case for considering methods to prevent disease, particularly in hospitalized patients.
Hyperimmune serum from human volunteer donors or humanized monoclonal antibodies directed towards surface components (e.g., capsular polysaccharide or surface protein adhesions) could both prevent bacterial adherence and also promote phagocytosis of bacterial cells. Indeed a prototype vaccine based on capsular polysaccharide from S aureus has been administered to volunteers to raise hyperimmune serum, which could be given to patients in hospital before surgery. A vaccine based on fibronectin binding protein induces protective immunity against mastitis in cattle and might also be used as a vaccine in humans.
When the molecular basis of the interactions between the bacterial surface proteins and the host matrix protein ligands are known it might be possible to design compounds that block the interactions and thus prevent bacterial colonization. These could be administered systemically or topically.
References
- Bhakdi S, Tranum-Jensen J. Alpha-toxin of Staphylococcus aureus. Microbiol Rev. 1991;55:733. [PMC free article: PMC372845] [PubMed: 1779933]
- Easmon CSF, Adlam C: Staphylococci and staphylococcal infections. Vols 1 and 2. Academic Press, London, 1983 .
- Foster TJ. Potential for vaccination against infections caused by Staphylococcus aureus. Vaccine. 1991;9:221. [PubMed: 2058264]
- Foster TJ, McDevitt D: Molecular basis of adherence of staphylococci to biomaterials. p. 31, In Bisno AL, Waldvogel FA (eds): Infections Associated with Indwelling Medical Devices, 2nd Edition. American Society for Microbiology, Washington, D.C., 1994.
- Lyon BR, Skurray R. Antimicrobial resistance in Staphylococcus aureus: genetic basis. Microbiol Reviews. 1987;51:88. [PMC free article: PMC373094] [PubMed: 3031442]
- Prevost G, Couppie P, Prevost P. et al. Epidemiological data on Staphylococcus aureus strains producing synergohymenotropic toxins. J Med Microbiol. 1995;42:237. [PubMed: 7707330]
- Rupp ME, Archer GL. Coagulase-negative staphylococci: pathogens associated with medical progress. Clin Infect Dis. 1994;19:231. [PubMed: 7986894]
- Schlievert PM. Role of superantigens in human disease. J Infect Dis. 1993;167:997. [PubMed: 8486972]
- Skinner GRB, Ahmad, A: Staphylococcal vaccines - present status and future prospects. p. 537. In Mollby R, Flock JI, Nord CE, Christensson B (eds): Staphylococci and Staphylococcal Infections. Zbl. Bakt. Suppl. 26, Fischer Verlag, Stuttgart, 1994 .
- Tenover F, Arbeit R, Archer G. et al. Comparison of traditional and molecular methods of typing isolates of Staphylococcus aureus. J Clin Microbiol. 1994;32:407. [PMC free article: PMC263045] [PubMed: 7908673]
- Vaudaux PE, Lew DP, Waldvogel FA: Host factors predisposing to and influencing therapy of foreign body infections. p. 1. In Bisno AL, Waldvogel FA (eds): Infections Associated with Indwelling Medical Devices. 2nd Ed. American Society for Microbiology, Washington, D.C., 1994 .
- General Concepts
- Introduction
- Taxonomy
- Identification of Staphylococci in the Clinical laboratory
- Epidemiology of Staphylococcus Aureus Infections
- Clinical Manifestations of S Aureus
- Pathogenesis of S Aureus Infections
- Adherence
- Avoidance of Host Defenses
- Damage to the Host
- Other Extracellular Proteins
- Enzymes
- Coagulase Negative Staphylococci
- Resistance of Staphylococci to Antimicrobial Drugs
- Future Prospects
- References
- Role of coagulase-negative staphylococci in human disease.[Vet Microbiol. 2009]Role of coagulase-negative staphylococci in human disease.Piette A, Verschraegen G. Vet Microbiol. 2009 Feb 16; 134(1-2):45-54. Epub 2008 Sep 11.
- The diversities of staphylococcal species, virulence and antibiotic resistance genes in the subclinical mastitis milk from a single Chinese cow herd.[Microb Pathog. 2015]The diversities of staphylococcal species, virulence and antibiotic resistance genes in the subclinical mastitis milk from a single Chinese cow herd.Xu J, Tan X, Zhang X, Xia X, Sun H. Microb Pathog. 2015 Nov; 88:29-38. Epub 2015 Aug 11.
- [Coagulase-negative staphylococci in normal and chronically inflamed conjunctiva].[Ophthalmologe. 1995][Coagulase-negative staphylococci in normal and chronically inflamed conjunctiva].Grasbon T, Miño de Kaspar H, Klauss V. Ophthalmologe. 1995 Dec; 92(6):793-801.
- Review [Correlation of slime production and pathogenicity of coagulase-negative staphylococci].[Orv Hetil. 1990]Review [Correlation of slime production and pathogenicity of coagulase-negative staphylococci].Végh Z, Gacs M. Orv Hetil. 1990 Feb 4; 131(5):231-4.
- Review Coagulase-negative staphylococci: role as pathogens.[Annu Rev Med. 1999]Review Coagulase-negative staphylococci: role as pathogens.Huebner J, Goldmann DA. Annu Rev Med. 1999; 50:223-36.
- Staphylococcus - Medical MicrobiologyStaphylococcus - Medical Microbiology
- ykqA [Bacillus subtilis subsp. subtilis str. 168]ykqA [Bacillus subtilis subsp. subtilis str. 168]Gene ID:939408Gene
Your browsing activity is empty.
Activity recording is turned off.
See more...