NCBI Bookshelf. A service of the National Library of Medicine, National Institutes of Health.
Center for Substance Abuse Treatment. Treatment for Stimulant Use Disorders. Rockville (MD): Substance Abuse and Mental Health Services Administration (US); 1999. (Treatment Improvement Protocol (TIP) Series, No. 33.)
This publication is provided for historical reference only and the information may be out of date.
Over the last several decades, research on substances of abuse has vastly improved our understanding of human behavior and physiology and the nature of substance abuse and dependence. Basic neurobiological research has enhanced our understanding of the biological and genetic causes of addiction. These discoveries have helped establish addiction as a biological brain disease that is chronic and relapsing in nature (Leshner, 1997). By mapping the neural pathways of pleasure and pain through the human brain, investigators are beginning to understand how abused psychoactive substances, including stimulants, interact with various cells and chemicals in the brain.
This new information has also improved our understanding of appropriate treatment approaches for different substance use disorders. This chapter describes the effects that cocaine and methamphetamine (MA) use have on the user's brain and behavior, which in turn leads to the stimulant users' unique needs. Knowledge of these effects provides the foundation for stimulant-specific treatment approaches. This knowledge will give treatment providers greater insight into stimulant users and why certain treatment approaches are more effective.
Stimulant Abuse And the Brain
According to National Institute on Drug Abuse Director Alan I. Leshner, Ph.D., the fundamental problem in dealing with any substance of abuse is to understand "the target" (i.e., the user). Therefore, to understand why people take drugs such as cocaine and MA and why some people become addicted, we must first understand what these drugs are doing to their target; that is, how stimulants affect the user.
Discussions of substance abuse and dependence often involve some discussion of the root causes--the societal and risk factors that lead to these conditions. To date, investigators have identified as many as 72 risk factors for substance use and dependence (Leshner, 1998). Among them are poverty, racism, social dysfunction, weak families, poor education, poor upbringing, and substance-abusing peer groups. These risk factors--as well as other environmental and genetic factors--only influence an individual's initial decision to use substances of abuse. But after initial use, an individual continues to use a substance because she likes its effects: Use modifies mood, perception, and emotional state. All of these effects are modulated through the brain; in order to understand this phenomenon, it is important to understand some basic neuroscience.
For substances of abuse to exert their effects, they must first get to the brain. The four most common routes of administering psychoactive (mood-changing) substances are (1) oral consumption (i.e., swallowing), (2) intranasal consumption (i.e., snorting), (3) inhalation into the lungs (generally by smoking), and (4) intravenously via hypodermic syringe.
A swallowed substance goes to the stomach and on to the intestinal tract. Some substances easily pass through the digestive tract into the bloodstream. Other substances are broken down into their chemical components (i.e., metabolized) in the digestive system, thereby destroying the substance.
Substances that are inhaled into the lungs adhere to the lining of the nasal passages (the nasal mucosa) through which they enter directly into the bloodstream. Inhaled substances are usually first changed into a gaseous form by igniting (e.g., marijuana) or volatilizing by intense heat (e.g., crack cocaine, the ice form of MA). The lungs offer a large surface area through which the gaseous form may quickly pass directly into the bloodstream.
Injected substances obviously enter the bloodstream directly, although at a somewhat regulated rate. In these last three routes of administration, substances enter the bloodstream in their unmetabolized form.
Once a substance enters the bloodstream, it is transported throughout the body to various organs and organ systems, including the brain. Substances that enter the liver may be metabolized there. Substances that enter the kidney may be excreted. If a female substance user is pregnant, and the substance is able to cross the placenta, then the substance will enter the fetus' bloodstream. Nursing babies may ingest some substances from breast milk.
To enter the brain, a substance's molecules must first get through its chemical protection system, which consists mainly of the blood-brain barrier. Tight cell-wall junctions and a layer of cells around the blood vessels keep large or electrically charged molecules from entering the brain. However, small neutral molecules like those of cocaine and MA easily pass through the blood-brain barrier and enter the brain. Once inside the brain, substances of abuse begin to exert their psychoactive effects.
Fundamentals of the Nervous System
The human nervous system is an elaborately wired communication system, and the brain is the control center. The brain processes sensory information from throughout the body, guides muscle movement and locomotion, regulates a multitude of bodily functions, forms thoughts and feelings, modulates perception and moods, and essentially controls all behavior.
The brain is organized into lobes, which are responsible for specialized functions like cognitive and sensory processes and motor coordination. These lobes are made up of far more complex units called circuits, which involve direct connections among the billions of specialized cells that the various substances of abuse may affect.
The fundamental functional unit of the brain's circuits is a specialized cell called a neuron, which conveys information both electrically and chemically. The function of the neuron is to transmit information: It receives signals from other neurons, integrates and interprets these signals, and in turn, transmits signals on to other, adjacent neurons (Charness, 1990).
A typical neuron (see Figure 2-1) consists of a main cell body (which contains the nucleus and all of the cell's genetic information), a large number of offshoots called dendrites (typically 10,000 or more per neuron), and one long fiber known as the axon. At the end of the axon are additional offshoots that form the connections with other neurons. Within neurons, the signals are carried in the form of electrical impulses. But when signals are sent from one neuron to another, they must cross the gap at the point of connection between the two communicating neurons. This gap is called a synapse. At the synapse, the electrical signal within the neuron is converted to a chemical signal and sent across the synapse to the target (i.e., receiving) neuron. The chemical signal is conveyed via messenger molecules called neurotransmitters that attach to special structures called receptors on the outer surface of the target neuron (Charness, 1990). The attachment of the neurotransmitters to the receptors consequently triggers an electrical signal within the target neuron. Approximately 50 to 100 different neurotransmitters have been identified in the human body (Snyder, 1986). Figure 2-2 illustrates a typical synaptic connection and depicts the chemical communication mechanism. Neurotransmitters may have different effects depending on what receptor they activate. Some increase a receiving neuron's responsiveness to an incoming signal--an excitatory effect--whereas others may diminish the responsiveness--an inhibitory effect. The responsiveness of individual neurons affects the functioning of the brain's circuits, as well as how the brain functions as a whole (how it integrates, interprets, and responds to information), which in turn affects the function of the body and the behavior of the individual. The accurate functioning of all neurotransmitter systems is essential for normal brain activities (National Institute on Alcohol Abuse and Alcoholism [NIAAA], 1994; Hiller-Sturmhfel, 1995).

Figure
Figure 2-1: The Typical Neuron.
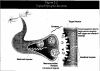
Figure
Figure 2-2: Typical Synaptic Junction.
The Limbic Reward System
The brain circuit that is considered essential to the neurological reinforcement system is called the limbic reward system (also called the dopamine reward system or the brain reward system). This neural circuit spans between the ventral tegmental area (VTA) and the nucleus accumbens (see Figure 2-3). Every substance of abuse--alcohol, cocaine, MA, heroin, marijuana, nicotine--has some effect on the limbic reward system. Substances of abuse also affect the nucleus accumbens by increasing the release of the neurotransmitter dopamine, which helps to regulate the feelings of pleasure (euphoria and satisfaction). Dopamine also plays an important role in the control of movement, cognition, motivation, and reward (Wise, 1982; Robbins et al., 1989; Di Chiara, 1995). High levels of free dopamine in the brain generally enhance mood and increase body movement (i.e., motor activity), but too much dopamine may produce nervousness, irritability, aggressiveness, and paranoia that approximates schizophrenia, as well as the hallucinations and bizarre thoughts of schizophrenia. Too little dopamine in certain areas of the brain results in the tremors and paralysis of Parkinson's disease.

Figure
Figure 2-3: The Limbic Reward System.
Natural activities such as eating, drinking, and sex activate the nucleus accumbens, inducing considerable communication among this structure's neurons. This internal communication leads to the release of dopamine. The released dopamine produces immediate, but ephemeral, feelings of pleasure and elation. As dopamine levels subside, so do the feelings of pleasure. But if the activity is repeated, then dopamine is again released, and more feelings of pleasure and euphoria are produced. The release of dopamine and the resulting pleasurable feelings positively reinforce such activities in both humans and animals and motivate the repetition of these activities.
Dopamine is believed to play an important role in the reinforcement of and motivation for repetitive actions (Di Chiara, 1997; Wise, 1982), and there is an increasing amount of scientific evidence suggesting that the limbic reward system and levels of free dopamine provide the common link in the abuse and addiction of all substances. Dopamine has even been labeled "the master molecule of addiction" (Nash, 1997).
When the nucleus accumbens is functioning normally, communication among its neurons occurs in a consistent and predictable manner. First, an electrical signal within a stimulated neuron reaches its point of connection (i.e., the synapse) with the target neuron. The electrical signal in the presynaptic neuron triggers the release of dopamine into the synapse. The dopamine travels across the synaptic gap until it reaches the target neuron. It then binds to the postsynaptic neuron's dopamine-specific receptors, which in turn has an excitatory effect that generates an internal electrical signal within this neuron. However, not all of the released dopamine binds to the target neuron's receptors. Extra dopamine may be chemically deactivated, or it may be quickly reabsorbed by the releasing neuron through a system called the dopamine reuptake transporter (see Figure 2-4). As soon as the extra dopamine has been deactivated or reabsorbed, the two cells are "reset," with the releasing neuron prepared to send another chemical signal and the target neuron prepared to receive it. Substances of abuse, and especially stimulants, affect the normal functioning of the dopamine neurotransmitter system (Snyder, 1986; Cooper et al., 1991).

Figure
Figure 2-4: Dopamine's Normal Action.
Neurological Reinforcement Systems
Psychologists have long recognized the importance of positive and negative reinforcement for learning and sustaining particular behaviors (Koob and LeMoal, 1997). Beginning in the late 1950s, scientists observed in animals that electrically stimulating certain areas of the brain led to changes in mental alertness and behavior. Rats and other laboratory animals could be taught to self-stimulate pleasure circuits in the brain until exhaustion. If stimulants such as cocaine or amphetamine were administered, for example, sensitivity to pleasurable responses was so enhanced that the animals would choose electrical stimulation of the pleasure centers in their brains over eating or other normally rewarding activities.
The process just described in which a pleasure-inducing action becomes repetitive is called positive reinforcement. Conversely, abrupt discontinuation of the psychoactive substances following chronic use was found to result in discomfort and behaviors consistent with craving. The motivation to use a substance in order to avoid discomfort is called negative reinforcement. Positive reinforcement is believed to be controlled by various neurotransmitter systems, whereas negative reinforcement is believed to be the result of adaptations produced by chronic use within the same neurotransmitter systems.
Experimental evidence from both animal and human studies supports the theory that stimulants and other commonly abused substances imitate, facilitate, or block the neurotransmitters involved in brain reinforcement systems (NIAAA, 1994). In fact, researchers have posited a common neural basis for the powerful rewarding effects of abused substances (for a review, see Restak, 1988). Natural reinforcers such as food, drink, and sex also activate reinforcement pathways in the brain, and it has been suggested that stimulants and other drugs act as chemical surrogates of the natural reinforcers. A key danger in this relationship, however, is that the pleasure produced by substances of abuse can be more powerfully rewarding than that produced by natural reinforcers (NIAAA, 1996).
Stimulants' Mechanisms of Action
On a short-term basis, stimulants exert their effects by disrupting or modifying the normal communication that occurs among brain neurons and brain circuits. Cocaine and MA have both been shown to specifically disrupt the dopamine neurotransmitter system. This disruption is accomplished by overstimulating the receptors on the postsynaptic neuron, either by increasing the amount of dopamine in the synapse through excessive presynaptic release or by inhibiting dopamine's pattern of reuptake or chemical breakdown (Cooper et al., 1991).
The use of cocaine and MA increases the amount of available dopamine in the brain, which leads to mood elevation (e.g., feelings of elation or euphoria) and increased motor activity. With cocaine, the effects are short-lived; with MA the duration of effect is much longer. As the stimulant level in the brain decreases, the dopamine levels subside to normal, and the pleasurable feelings dwindle away.
A growing body of scientific research based on animal research and brain imaging studies in humans suggests that the chronic use of stimulants affect dopaminergic neurons in limbic reward system structures (e.g., the VTA, nucleus accumbens). These effects may underlie addiction to stimulants. Although the neurochemical pathways of stimulant addiction are not definitively established, a few researchers have found evidence of changes in the structure and function of brain neurons after chronic stimulant use in humans. Some researchers propose that the changes may come from dopamine depletion, changes in neurotransmitter receptors or other structures, or changes in other brain messenger pathways that could cause the changes in mood, behavior, and cognitive function associated with chronic stimulant abuse (Self and Nestler, 1995).
Animal studies have demonstrated that high doses of stimulants can have permanent neurotoxic effects by damaging neuron cell-endings (e.g., Selden, 1991). The question of whether stimulants can produce similar effects in humans remains to be answered. Researchers hope that recently developed brain imaging techniques will help provide the answer. At this time, there is only speculation that such permanent damage may underlie the long-term cognitive impairments seen in some chronic stimulant users. The continuing development and application of new technologies will help expand our knowledge of the neurological effects of stimulants in humans. (The medical aspects of stimulant use disorders are discussed in Chapter 5.)
Abuse and Dependence
Addiction is a complex phenomenon with important psychological and social causes and consequences. However, at its core, it involves a biological process: the effects of repeated exposure to a biological agent (a substance) on a biological substrate (the brain) over time (Nestler and Aghajanian, 1997). Ultimately, adaptations that substance exposure elicits in individual neurons alter the functioning of those neurons, which in turn alters the functioning of the neural circuits in which those neurons operate. This eventually leads to the complex behaviors (e.g., dependence, tolerance, sensitization, craving) that characterize addiction (Koob, 1992; Kreek, 1996; Wise, 1996; Koob and LeMoal, 1997).
A general definition of substance abuse is the habitual use of a substance not needed for therapeutic purposes, such as solely to alter one's mood, affect, or state of consciousness. The continued abuse of the substance may lead to adverse physiological, behavioral, and social consequences. A substance-dependent individual will continue his use despite these adverse consequences. Moderate chronic use or severe short-term use of substances may lead to abuse, which may eventually lead to addiction components (Ellinwood, 1974; Hall et al., 1988; Kramer, 1969).
Chronic substance abuse results in a complex set of physiological and neurological adaptations. These adaptations are simply the body's attempt to adjust to or compensate for substance-induced impairments. Repeated exposure to a substance can also lead to adaptations in the reward circuitry that opposes and/or neutralizes a substance's effects (i.e., counteradaptation). Substance addiction (or substance dependence) is manifested by (1) psychological craving (see the following section); (2) tolerance (the need for increasing amounts of the substance to reproduce the initial level of response, or sometimes to simply stave off the unpleasant effects of withdrawal); (3) sensitization (discussed in the section on the medical effects of stimulants); and (4) symptoms of withdrawal upon cessation of use, indicating physiological dependence.
Social and behavioral manifestations of dependence include the reduced ability to function at work or home and may include displays of erratic, moody, or anxious behavior.
Similar to other substances of abuse, moderate chronic use or severe short-term use of stimulants in any form may lead to abuse or dependence (Ellinwood, 1974; Hall et al., 1988; Kramer, 1969). Clinical observations of abuse patterns for both cocaine and MA have noted that, in general, there is an estimated 2- to 5-year latency period between first use and full-blown addiction. However, clinical experience and anecdotal evidence suggest that the latency period may be shortened to less than 1 year by rapid routes of administration (e.g., injection, smoking) and increased stimulant purity (e.g., ice, crack). With increasing use, the user may develop tolerance to the effects of stimulants and may need to keep increasing the amount taken to produce the desired psychological effects. As chronic abuse progresses, users prefer the stimulant over enjoyable activities and eventually may prefer it over food and sex (Hall et al., 1988). At that point, the individual will usually continue her use even when faced with continuing adverse consequences--the hallmark of substance dependence. Abrupt discontinuation of the psychoactive substance following chronic use generally results in discomfort, dysphoria, and behaviors consistent with craving. The user is now motivated to use a substance in order to avoid discomfort and dysphoria. This shift from substance use as positive reinforcement to negative reinforcement is, perhaps, one of the foremost characteristics of late-stage addiction.
Drug Craving and Memory
The degree to which learning and memory sustain the addictive process has only recently been addressed. Researchers believe that each time a neurotransmitter like dopamine floods across a synapse, circuits that trigger thoughts and memories and that motivate action become hardwired in the brain. The neurochemistry supporting addiction is so powerful that people, objects, and places associated with substance use are also imprinted on the brain.
Craving, a central aspect of addiction, is a very strong learned response with powerful motivational properties often associated with specific memories (i.e., conditioned cues and triggers). Cues--any stimuli (substance-using friends, locations, paraphernalia, moods) repeatedly paired with substance use over the course of a client's addiction--can become so strongly associated with the substance's effects that the associated (conditioned) stimuli can later trigger arousal and an intense desire for the substance and lead to relapse. High relapse rates are common in cocaine addiction even after physical withdrawal and abstinence have been achieved.
Brain-imaging studies have shown that cue-induced drug craving may be linked to distinct brain systems involved in memory (e.g., London et al., 1990; Stapleton et al., 1995). Brain structures involved in memory and learning, including the dorsolateral prefrontal cortex, amygdala, and cerebellum, have been linked to cue-induced craving (Grant et al., 1995). A network of these brain regions integrates emotional and cognitive aspects of memory and triggers craving when it reacts to cues and memories. These cues and memories also play an important role in reinforcing substance use (Grant et al., 1995).
Most substance treatment programs recognize the power of these factors in triggering relapse and warn clients to avoid everything previously associated with their substance use--a tall order for a client in an urban environment saturated with the substance and its associated reminders. Treatment approaches that address these learning and memory issues of addiction may prove effective. For example, Childress developed treatment strategies to help clients reduce craving and arousal during encounters with substance-related stimuli (Childress, 1994). In the laboratory, clients are given repeated, passive exposure to substance-reminding cues in a substance-free protected environment. The research finds that initial arousal and strong craving produced by the cues eventually decrease (Childress, 1994). Better understanding of the relationship of learning and memory to the addiction process may lead to new treatment approaches.
Role of New Technologies
The recent development of noninvasive brain imaging has created a powerful new tool for demonstrating not only the short-term effects of substance use, but also the longer term consequences of chronic substance abuse and addiction. These tools have allowed researchers to boldly go where they previously could not--literally into the depths of a living human brain. Such noninvasive techniques can depict normal and abnormal functioning of different brain areas by measuring metabolic activity (i.e., glucose utilization). They can identify substance-induced structural changes and physiological adaptations. Through a combination of techniques, they can observe the altered "processing" of information in various circuits as the brain responds to substance use.
Using these techniques, investigators have been able to identify brain structures involved in craving, map the emotions of substance users, plot the neurobiological basis of substance-induced euphoria, and more. For example, researchers have used magnetic resonance imaging (MRI) and spectroscopy to see how brain structures change as substances produce their effects. Others have used a functional imaging technique called phosphorus magnetic resonance spectroscopy (31P MRS) to show that chronic substance abuse is accompanied by abnormal metabolism in some areas of the brain that seems to return to normal when people stop using substances (Christensen et al., 1996). Positron emission tomography (PET) has revealed subtle alterations in the dopamine receptors of stimulant users' brains (Iyo et al., 1993). More recent PET studies have demonstrated long-term vulnerability to chronic stimulant abuse (Melega et al., 1997a; Volkow et al., 1996, 1997b). Another PET study has established a dose-response relationship between cocaine and the drug's subjective effects: The greater the amount of cocaine that is administered, the greater the high experienced by the user (Volkow et al., 1997a).
Other researchers combined electroencephalograms (EEGs) and MRI to produce a topographic brain map showing increased electrical activity (in the form of beta waves) during stimulant withdrawal (Herning et al., 1997). Mapping EEG activity during stimulant use and withdrawal may allow researchers to further document substance-induced neuropsychological impairments.
Although much is known about the effects of stimulants in animals, there is little such knowledge of these effects in humans (CSAT, 1997). The continuing development and application of new technologies such as noninvasive brain imaging will allow researchers to improve their understanding of how stimulants affect the human brain. Greater understanding of the underlying neuronal impairments of stimulant abuse will aid in the development of new, more effective treatment approaches.
General Effects Of Stimulants
Substances of abuse--and stimulants in particular--appear to increase the brain's levels of free dopamine in a dose-dependent manner; that is, more dopamine is available when higher doses of the substance are administered (Nash, 1997). The higher the substance dose, the greater the feelings of elation, euphoria, and satisfaction, and as the dopamine levels and pleasurable feeling subside, there is an intense desire to replicate the feelings of pleasure by administering another dose of the substance. This tendency toward repeated administration is characteristic of stimulant abuse and underlies most of the other effects of stimulants, as well as most other addictive substances.
Continued use often leads to adverse consequences, which may include neuropsychological impairment and diminished physical health. Work performance and social and family relations can be adversely affected, and the risk of arrest and conviction on drug-related charges increases. Even after a stimulant user discontinues use, impairments in cognition and functioning may persist, and there may even be persistent psychiatric symptoms (Wada and Fukui, 1990). Cravings for the stimulant's effects tend to linger, even after abstinence has been achieved, and the potential for relapse is high.
Medical Effects
Acute effects
The general acute effects of stimulants have been well documented. Among a range of physiological responses, stimulants are known to raise both systolic and diastolic blood pressure, increase heart rate, increase respiration rate, increase body temperature, cause pupillary dilation, heighten alertness, and increase motor activity (CSAT, 1997).
Acute effects from excessive doses include dangerously rapid and erratic heartbeat, cerebral hemorrhaging, seizures/convulsions, respiratory failure, stroke, heart failure, brain damage, coma, and death (CSAT, 1997).
Stimulants are also known to cause sensitization (i.e., the opposite of tolerance), for which multiple drug exposures eventually produce some new adverse reaction. For example, in animals, seizures do not typically occur after single low-to-moderate doses. But with repeated exposure, an animal can become sensitized to the stimulant and may have a seizure after a single, previously harmless, dose.
Chronic effects
Although the effects of chronic stimulant abuse in humans has not been well documented, some of the chronic effects include organ toxicity, compromised health (e.g., underfed, malnourished, poor hygiene), dental problems, and dermatitis. (For a complete discussion of the medical aspects of stimulant use, see Chapter 5.)
Psychological Effects
The immediate psychological effects of stimulant administration include a heightened sense of well-being, euphoria, excitement, heightened alertness, and increases in motor activity. Stimulants also reduce food intake, reduce sleep time, and may increase socialization activities. Stimulants may also enhance performance of certain types of psychomotor tasks.
High doses may result in restlessness and agitation, and excessive doses may produce stereotypic behaviors (repetitive and automatic acts). Chronic psychological effects of stimulant use include various psychiatric disorders such as psychosis, paranoia, and suicidal tendencies.
There may also be neurological impairments and cognitive deficits. Tolerance eventually develops to many of the behavioral effects of stimulants, so that increasing doses are required to achieve the same effect.
The administration of stimulants--particularly if smoked or injected intravenously--can have immediate and often very intense effects on the user. However, the "rush" and subsequent feelings of euphoria may just as quickly fade. The intense effects can be followed by a dysphoric "crash." To stave the crash, the user will administer another dose of the stimulant, which again produces a rush and subsequent crash.
This cycle will go on again and again. This pattern of frequently repeated dosing known as bingeing may continue for up to 3 sleepless days. During this period, the user may not eat and may lapse into a severe depression, followed by worsening paranoia, belligerence, and aggression--a period known as tweaking.
Bingeing eventually ends when the user depletes his supply of stimulants or simply collapses from sheer exhaustion. The stimulant user may then sleep for several days, only to awaken and begin the cycle again.
There is a great amount of anecdotal evidence on the relationship of stimulant use and various sexual behaviors. Stimulants may be used during sexual activities to intensify sexual acts, heighten pleasure, lengthen the duration of intercourse, and lessen inhibitions. The abuse of stimulants is also known to lead to uncharacteristically aberrant or deviant sexual behaviors, the use of prostitutes, and HIV high-risk behaviors (Rawson et al., 1998b).
Effects of Route of Administration
Cocaine and MA can be smoked, snorted, injected, or ingested orally. These various routes of administration differ in dosage and in the rapidity and intensity of effect, which may affect the course of abuse and dependence. Some evidence suggests that the onset of dependence varies according to the route of administration (DEA, 1995). The route of administration affects the amount (i.e., the dosage) of stimulant delivered to the brain, the speed at which it is delivered, and the resulting intensity of the stimulant's effects.
The intensity of the psychological effects of stimulants, as with most psychoactive drugs, depends on the dose and rate of entry to the brain. For example, when snorted, stimulants generally reach the brain within 3 to 5 minutes, and the resulting rush or "high" may not be perceived as immediate; intravenous administration produces a rush in about 15 to 30 seconds; whereas smoking produces an almost immediate effect (ONDCP, 1998a).
Because of the rapidity of delivery and higher dosages, the smoking of stimulants produces a high that is said to be far more intense than those produced through other routes of administration.
Route of administration has been shown to affect the resulting level of stimulant in the body. In a comparison of oral ingestion versus smoking, Cook measured plasma levels of MA after oral administration and after smoking (see Figure 2-5) (Cook, 1991). For the oral dose of 0.25 mg/kg, plasma levels began to rise 30 minutes after ingestion and reached peak levels (approximately 38 ng/mL) at about 3 hours after ingestion. Plateau levels were maintained until about hour 4 and then slowly declined over the next 4 hours. After smoking (dose of about 21 mg/subject), MA plasma levels approximated 80 percent of peak levels within minutes, peaked (approximately 42 ng/mL) at about 2 hours after administration, maintained a peak plateau for another 2 hours, and then slowly declined over the next 4 hours. By comparison, plasma levels of smoked cocaine and smoked MA both peaked rapidly (Cook, 1991). Plasma levels of smoked cocaine (dose of 21 to 22 mg/subject) peaked at approximately 240 ng/mL at about 5 to 10 minutes after administration. Cocaine plasma levels then declined rapidly, dropping to 50 percent of maximum level (half-life) within 1 hour. Smoked MA (dose of 21 to 22 mg/subject) neared peak levels (approximately 50 ng/mL) within minutes and continued to climb until about 2 hours after administration before slowly tapering off. However, half-life levels were not reached until 11 to 12 hours after administration (see Figure 2-6). The long plateau effect and the much longer half-life of MA versus cocaine suggests considerable dangers in repeated smoking of MA because remarkably higher plasma concentrations could be expected to occur if the dose is repeated, even at fairly long intervals (Cook, 1991). Because stimulants exert their effects in a dose-dependent manner, the route of administration has serious neurological, medical, psychiatric, and neurocognitive implications for the stimulant user and the treatment provider. The intense highs produced by smoking crack cocaine or ice MA can lead to equally intense "lows" during withdrawal (e.g., dysphoria, depression, irritability, anxiety, paranoia, dramatic mood swings). The subsequent cravings can also be extremely intense. Prolonged high doses of stimulants (e.g., during bingeing) may cause greater and longer lasting neurological damage, which in turn may lead to greater and longer lasting cognitive deficits. The onset of stimulants' chronic effects varies across individuals, and although there are few data to predict how long it will take for any user to begin suffering from the chronic effects of stimulant abuse and dependence, onset is probably related to the size of the doses, the frequency of dosing, and the route of administration. However, in general, the higher the doses and the more frequently the doses are administered, the more quickly the chronic effects will appear. From a treatment provider's perspective, a stimulant user's preferred route of administration affects the extent and depth of chronic effects and, therefore, has implications for choosing the most appropriate treatment approach. (See Chapter 4 for a full discussion on the practical applications of treatment strategies. For a discussion on route-of-administration effects on toxicity and adverse reactions, see Chapter 5.)
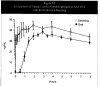
Figure
Figure 2-5: Comparison of Plasma Levels of Methamphetamine After Oral Administration and Smoking.

Figure
Figure 2-6: Comparison of Plasma Levels of Methamphetamine And Cocaine After Smoking.
Cocaine
Acute Effects
Cocaine has two main pharmacological actions. It is both a local anesthetic and a central nervous system (CNS) stimulant--the only drug known to possess both of these properties. Cocaine exerts its local anesthetic actions by blocking the conduction of sensory impulses within nerve cells. This effect is most pronounced when cocaine is applied to the skin or to mucous membranes. Cocaine hydrochloride has approved medical use as a local anesthetic in surgery of the nose, throat, and larynx.
As a CNS stimulant, cocaine affects a number of neurotransmitter systems, but it is through its interaction with the dopamine and the limbic reward system that cocaine produces some of its most important effects, including its positive reinforcing effects. The major influence of cocaine on the dopamine system is its ability to block the synaptic reuptake of dopamine. As shown in Figure 2-7, cocaine does not directly "stimulate" the dopamine system; rather, it causes the system to be stimulated by preventing dopamine from being removed from the intracellular space. Cocaine blockade of the dopamine reuptake transporter extends the availability of dopamine in the synaptic space where it continues to occupy the dopamine receptor and causes the postsynaptic neurons to fire for a longer than normal period. This extended firing of the postsynaptic neurons resulting from prolonged dopamine receptor activity is initially experienced subjectively by the cocaine user as a positive sensation involving increased energy, arousal, and stimulation. A recent study has demonstrated a relationship between the intensity of cocaine's subjective effects and the degree to which the dopamine reuptake transporter is blocked (Volkow et al., 1997a). The effects experienced by users of cocaine during the initial period of their use are generally mood-altering in a positive manner (Washton, 1989). For most individuals, the subjective experience of the acute effects includes a generalized state of euphoria in combination with feelings of increased energy, confidence, mental alertness, and sexual arousal.

Figure
Figure 2-7: Cocaine Blockade of the Dopamine Reuptake Transporter.
Under the proper environmental circumstances, individuals also report that cocaine heightens their ability to concentrate, increases sexual excitement, increases their sociability, and decreases any preexisting shyness, tension, fatigue, depression, or boredom. Many people feel more talkative, more intensely involved in their interactions with others, and more playful and spontaneous when high on cocaine. As they come down from their cocaine high, some users experience temporary unpleasant reactions and aftereffects, which may include restlessness, anxiety, agitation, irritability, and insomnia. During this "rebound" period, suspiciousness, confusion, hyperarousal, and other elements of paranoid thinking may also appear.
With continued escalating use of cocaine, the user becomes progressively tolerant to the positive effects while the negative effects steadily intensify (Washton, 1989). Users report that the highs are not so high anymore and the rebound aftereffects increasingly lead to a dysphoric, depressed state. These new "lows" may reinitiate the desire for more cocaine in a futile attempt at mood normalization. The search for the previously experienced high will eventually leave the user in the depths of depression and despair.
When snorted, smoked, or injected intravenously, cocaine quickly produces an intense high. But because it is rapidly metabolized in the body, this high is short-lived. Efforts to replicate the initial high prompt users to take it often and repeatedly. Because of its mechanism of action, cocaine may produce strong craving and strong conditioning of cues associated with its use. The results of a recent brain imaging study revealed that cocaine's fast uptake in the brain has a major role in its rewarding effects and that its fast clearance from the brain sets the stage for frequent abuse, craving, and the binge pattern in cocaine addiction (Volkow et al., 1996). These researchers postulated that dopaminergic activation of the limbic reward system is involved in the rewarding effects of cocaine (and perhaps most, if not all, substances of abuse) and that continued activation of this system may lead to long-term changes in the associated neural circuits that perpetuate the compulsive administration of this drug (see below).
Cocaine use also has acute adverse physiological effects involving the respiratory, cardiovascular, and central nervous systems. Systemic toxicity to cocaine is characterized by profound sympathetic stimulation of the respiratory, cardiovascular, and central nervous systems, producing a combination of medical and psychological effects sometimes known as the "cocaine reaction." (For additional details on the medical aspects of cocaine abuse, see Chapter 5.)
Chronic Effects
For many cocaine users, the initial experimental use begins to give way to more frequent or regular use. With continued, intensified use, the "casual" user will progress to the abuse stage, requiring larger and larger doses to achieve the desired effects. The abuser may become obsessed with the rituals of cocaine administration and may find that many common items or situations trigger cravings for the drug. For some, abuse will lead to full-fledged addiction. There will be overwhelming urges and cravings for cocaine, and there may be an inability to self-limit or control use. The cocaine addict will deny that she has a drug use problem and will continue use of cocaine despite the negative consequences. At this stage, the adverse consequences of cocaine addiction have probably affected all aspects of the user's life. The addict has succumbed to what Dr. Sidney Cohen called cocaine's "pharmacological imperative" (Washton, 1989). Figure 2-8 lists the characteristics of the stages of cocaine addiction.
The timetable for the onset of the chronic effects of cocaine use varies across individuals and may depend on the size of the doses, the frequency of dosing, and the route of administration. There are no data to base a prediction on how long it will take for any individual to begin to suffer from the chronic effects of cocaine use. However, similar to the effects of MA, the higher the doses and the more frequently the doses are administered, in general, the more quickly the chronic effects of cocaine will appear. In addition, intranasal administration (snorting) is associated with slower onset of chronic effects than if cocaine is smoked (freebase or crack) or injected intravenously. Clearly, there are tremendous individual differences in this timetable, with some individuals reporting an ability to use for extended periods with few signs of negative consequences and others reporting a very dramatic onset of severe detrimental effects as soon as a few weeks or months after initiation of cocaine use.
Physically, the cocaine addict may appear thin or even emaciated. Personal hygiene and self-care may be neglected, and medical and dental needs may go unmet. Because cocaine suppresses appetite, the user fails to eat properly and may suffer from vitamin deficiencies. Severe addicts may ignore food, clothing, shelter, and sexual needs.
Psychologically, cocaine's chronic effects are exactly the opposite of the desired initial effects. Continued cocaine use increases paranoia and confusion and causes an inability to concentrate and an inability to perform sexually. The same substance that acutely produced a mild sensation of arousal and decreased fatigue, on a chronic basis results in chest pain, insomnia, anorexia, episodic depression, and extreme fatigue.
From a treatment perspective, the curious thing is that the user often accurately perceives and attributes the pleasurable, acute effects to the use of cocaine. However, he frequently is unable or unwilling to recognize the relationship of the negative, chronic effects to the use of cocaine. Although it may be dramatically apparent to family and friends that the effects of cocaine are highly detrimental and destructive to the user, the user may insist that the use of cocaine is very helpful and beneficial. The extensive health-compromising effects of cocaine abuse are apparent when examining the behavioral and psychological profile of clients as they enter substance treatment. Generally, these clients exhibit a pronounced disruption in healthy behaviors and an elevation in dysphoric emotions including anxiety, depression, and paranoia (Castro et al., 1992).
Chronic abuse of cocaine may cause neuropsychological impairments (O'Malley et al., 1992) as well as neuropsychiatric syndromes (Herning et al., 1997). Cocaine-induced cognitive deficits can last up to 3 months after heavy use before baseline functioning is restored. In their review, Weinrieb and O'Brien found a strong association between the chronic use of cocaine and deficiencies in short-term auditory recall, memory, concentration--especially for nonverbal abstracting and problem solving--and slowed reaction time (Weinrieb and O'Brien, 1993).
The physical, psychological, and cognitive effects of chronic cocaine use reflect the underlying physiological effects; at the heart of these effects is cocaine's impact on the neurotransmitter dopamine.
Methamphetamine
Acute Effects
Although research efforts continue to focus on the effects of MA, there are limited data on MA's effects on humans (CSAT, 1997). Much of the available information has been surmised from the literature on cocaine. However, the physiological effects of MA are generally similar to those of cocaine: increased heart rate, elevated blood pressure, elevated body temperature, increased respiratory rate, and pupillary dilation. Other acute effects include rapid heart rate, irregular heart rate, and irreversible, stroke-producing damage to small blood vessels in the brain.
MA's psychological effects, like those of cocaine, include a heightened sense of well-being or euphoria, increased alertness, increased vigor, decreased food intake, and decreased sleep time. Acute administration has been shown to increase socialization in humans. High doses may produce repetitive and automatic acts in both humans and animals, and in humans, may cause irritability, aggressive behavior, excitement, auditory hallucinations, and paranoia (delusions and psychosis). Dangerously elevated body temperature and convulsions occur with MA overdoses, and if not treated immediately, can result in death. With continued use, tolerance develops to the behavioral effects, and repeated exposure may produce sensitization. MA users tend to engage in violent behavior. Mood changes are common, with the user rapidly changing from friendly to hostile.
The course of addiction to MA is believed to be similar to that of cocaine. Even the underlying neurological effects of MA are similar to the effects produced by cocaine: increased levels of free dopamine in the brain's limbic reward system. The MA "withdrawal syndrome" is like that of cocaine, but due to the longer effects of MA, withdrawal may be more intense and protracted. Several hours after last use, the MA user experiences a drastic drop in mood and energy levels. Sleep--which may be promoted by the use of secondary substances such as alcohol, barbiturates, and benzodiazepines--finally begins and may last for several days. Upon awakening, the user may experience severe depression, perhaps lasting for several weeks. While in this depressed state, the user has an increased risk of suicide. But once the user feels that she "has recovered" from a bingeing episode, cravings set in, and the cycle often begins again.
There are three essential differences between cocaine and MA. First, MA is thought to enhance CNS neurotransmission by increasing the presynaptic release of dopamine within the limbic reward system. Second, recent research has demonstrated MA's neurotoxicological effects in animals and has begun to support the hypothesis that MA is neurotoxic in humans. Unlike cocaine, MA does cross neuronal cell membranes and will enter into the microscopic sacs (called vesicles) where neurons store dopamine. MA is believed to damage the storage sacs and the neurons' axonal endings such that dopamine leaks uncontrollably into the synapse (see Figure 2-9). MA can also cause neurotoxicity indirectly by mobilizing dopamine out of the safe storage vesicles within the neuron and into the neuron's cytoplasm (i.e., the cell's internal material) where it is converted to toxic and reactive chemicals. Third, cocaine is rapidly metabolized by plasma and tissue enzymes, whereas MA is metabolized at a much slower rate, which results in a longer duration of action (Cook, 1991; ONCDP, 1998b). Although the half-life (effective duration of action) of cocaine is 1 to 2 hours, a single dose of MA may produce an effect for 8 to 12 hours. The fact that MA is metabolized at a slower rate also allows more time for MA to exert its neurotoxicological effects.
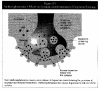
Figure
Figure 2-9: Methamphetamine's Effects on Synaptic and Intraneuronal Dopamine Leakage.
The sustained high plasma levels suggest considerable dangers in repeated smoking of MA because remarkably higher plasma concentrations could be expected to occur if the dose is repeated, even at fairly long intervals (Cook, 1991).
Chronic Effects
Chronic abuse of MA may result in inflammation of the heart lining and, among users who inject the drug, damaged blood vessels and skin abscesses. Chronic users may also have episodes of violent behavior, paranoia, anxiety, confusion, and insomnia. Heavy users show progressive social and occupational deterioration. Psychotic symptoms may sometimes persist for months or years after use has ceased.
Some of the most frightening research findings about MA suggest that its prolonged use not only modifies behaviors, but literally changes the brain in fundamental and long-lasting ways. Animal studies have shown that chronic use of MA can significantly reduce brain dopamine levels for up to 6 months after last use, with less significant reductions persisting for up to 4 years. MA impairs the functioning of both the dopamine system and the serotonin system (serotonin is another important CNS neurotransmitter). MA-induced neuronal toxicity is specific to certain brain regions (primarily the limbic reward system), and this toxicity is reflected both biochemically and anatomically. The adverse effects produced by MA are often long-lasting, and there is some speculation that some types of damage may be permanent. Finally, these impairments in brain functioning may underlie the cognitive and emotional deficits seen in many MA users. Understanding the chronic effects of MA use is essential for treatment providers who serve this population.
Animal studies have shown that high dose regimens of MA significantly deplete neurotransmitter levels, particularly those of dopamine (e.g., Seiden et al., 1976). Subsequent studies replicated these findings (e.g., Ricaurte et al., 1980) and demonstrated that these depletions were evident up to 4 years after cessation of MA administration (Woolverton et al., 1989). A more recent study demonstrated that chronic amphetamine exposure in monkeys could produce long-term effects on the brain's ability to produce dopamine (Melega et al., 1997a). Significant depletion of dopamine persisted 6 months later; even after 1 year, the brain dopamine levels were only at 80 percent of their preexposure levels. In a radiotracer study of humans, Iyo and colleagues (Iyo et al., 1993) revealed reductions in dopamine receptor binding availability in brain areas such as the frontal cortex and striatum in MA users. Although there is little current evidence on MA's chronic effects in humans, animal research has proven that prolonged or heavy use of MA dramatically reduces the brain's ability to produce dopamine.
Numerous animal studies have demonstrated that MA can damage both dopamine and serotonin systems (e.g., Peat et al., 1983; Robinson and Becker, 1986; Seiden et al., 1976; Trulson and Trulson, 1982a, 1982b; Wagner et al., 1979). MA toxicity occurs after repeated high-dose administration, and it is selective for certain neuronal systems, particularly those in the limbic reward system (e.g., striatum, substantia nigra, nucleus accumbens). Within these brain circuits, MA has been shown to reduce the number of nerve fibers, impair normal physiological functioning, and destroy both axons and axon terminals (i.e., at synaptic junctions). These studies have also shown that MA toxicity is highly dependent on dose, route of administration, and frequency with which the drug is given.
Prolonged or heavy use of MA decreases the brain's ability to manufacture dopamine. This impairment may persist for up to 1 year after the user has stopped taking MA. Researchers now believe that those changes in dopamine and the damage done to dopamine and serotonin neurons are responsible for the chronic effects of MA use that are much more pronounced than the acute effects.
If MA does indeed cause damage to dopamine and serotonin systems in humans, then there are ramifications to consider. One of the outcomes of chronic MA use is psychosis. Psychotic individuals are often treated with drugs to reverse or return their brain functions to normal, and most antipsychotic medications work by changing the activities of the dopamine and serotonin neurons. The unanswered question is: Will antipsychotic medications be able to effectively treat MA-induced psychoses in individuals whose dopamine and serotonin systems have been impaired by chronic MA abuse? To date, there have been few, if any, studies investigating antipsychotic medications for the treatment of chronic MA abuse and dependence.
In summary, although there is much evidence of MA's neurotoxicity in animals, the issue of whether MA causes permanent damage to dopamine and serotonin neurons in humans remains very much an unanswered question. Because of the inherent dangers associated with this type of research, the information will have to come from postmortem studies, advanced neuroimaging studies, and the development of new strategies for detecting neurotoxicity--possibly through the use of operant behavioral pharmacology. Finally, the degree of neurotoxicity must be placed in perspective, and the functional consequences require further scrutiny to determine the impact of chronic MA abuse on human brain function.
Summary
Recent research has shown how stimulants such as cocaine and MA exert their effects on the user's nervous system and change the user's feelings, emotions, and behavior. There is now a greater understanding of neurological reinforcement systems, how substance use can lead to dependence, and the roles that craving and memory play in sustaining addiction. Although there is currently a dearth of research regarding the neurologic, medical, psychiatric, and neurocognitive effects of stimulants in humans (CSAT, 1994b, 1997), animal studies have demonstrated cocaine's and MA's ability to disrupt normal brain function and cause long-lasting and perhaps permanent neurological impairments. With continuing research and the development of new imaging technologies, the full extent of these stimulants' effects on humans will eventually be revealed. This new information should continue to assist in the development of new and improved approaches for treating stimulant use disorders.
- Chapter 2—How Stimulants Affect the Brain and Behavior - Treatment for Stimulant...Chapter 2—How Stimulants Affect the Brain and Behavior - Treatment for Stimulant Use Disorders
- AKR1C5P aldo-keto reductase family 1 member C5, pseudogene [Homo sapiens]AKR1C5P aldo-keto reductase family 1 member C5, pseudogene [Homo sapiens]Gene ID:100996684Gene
- STK_RS11890 [Sulfurisphaera tokodaii str. 7]STK_RS11890 [Sulfurisphaera tokodaii str. 7]Gene ID:1460225Gene
- PPBPP1 pro-platelet basic protein pseudogene 1 [Homo sapiens]PPBPP1 pro-platelet basic protein pseudogene 1 [Homo sapiens]Gene ID:728045Gene
- FOXN3-AS1 FOXN3 antisense RNA 1 [Homo sapiens]FOXN3-AS1 FOXN3 antisense RNA 1 [Homo sapiens]Gene ID:400236Gene
Your browsing activity is empty.
Activity recording is turned off.
See more...