NCBI Bookshelf. A service of the National Library of Medicine, National Institutes of Health.
Madame Curie Bioscience Database [Internet]. Austin (TX): Landes Bioscience; 2000-2013.
In this review, we summarized the historical and experimental basis of cancer immunity and the role of fever and of artificial elevation of temperature on immunity. The interactions of heat in vitro and in vivo on cytotoxicity of immune competent cells are discussed as their positive contribution on the various cancer mmunotherapeutic strategies. Furthermore we have described the link existing among Heat shock proteins, Toll like receptors and innate immunity justifying the use of temperature elevation for treating cancer. The disputed and life threatening effect of local and whole body hyperthermia on metastasization is also reviewed.
Introduction
The role of the immune system in eradicating malignant cells is not yet clarified, however spontaneous regression of some cancers has been demonstrated to be associated to the induction of fever and activation of immunity.1-3 The crucial importance of fever in these regressions justifies the attempt to induce artificial thermal elevation of body temperature (hyperthermia) for mimicking natural fever effects on cancer.3-7
Tumor Immunity
Background
Tumor regression in vivo is mediated by a complex interplay between two main mechanisms: innate and adaptive immune response (Tables 1, 2), that is involved with the immune recognition of cancer cells. Innate mechanism [involving soluble and cellular components (Table 1)8-10 may trigger inflammatory events in the tumor microenvironment and in presence of a local adequate cytokine combination (IL-2, IL-12, IL-18, IL-23), stimulate dendritic cells (DCs),11 the most specialized antigen presenting cells (APCs), to react against tumor specific surface antigens (TAAs).12-16 After engulfment by DCs, TAAs are presented to naive T cells associated to Major Histocompatibility complex (MHC).16 Naive T cells activation occurs when the antigenic peptide-MHC complex interacts with the T Cell Receptor (TCR). However TCR receptor binding is not sufficient for a full activation of T cells unless costimulatory molecules interact with the specific ligands on the surface of APC. The presence or absence of costimulatory signals like B7-1(CD80), B7-2(CD86) and CD40/CD40L), determines whether immune response becomes anergic or tolerant. CD40/CD40L is expressed transiently following TCR activation on the surface of CD4+ cells and it is a key molecule in mediating the activation of B cells and in controlling CD8 T cells. Antigens can be associated to MHC I or MHC II class complexes and are presented by DCs to TCR of CD8+ and CD4+ T cells, respectively associated to the proper costimulatory molecules (fig. 1). Both CD4+ and CD8+ cells after activation and costimulation produce a series of cytokines that differentiate T-Helper (CD4+) lymphocytes in two subpopulations (TH 1, TH 2 cells). TH 1 cells produce IL-2, IFN-γ, TNF-α and granulocyte macrophage colony stimulating factor (GM-CSF) that increase the activity of macrophages, and the expression of MHC class 1 molecules on the surfaces of CD8+ cells. TH 2 secretes another group of cytokines IL-4, IL-5 and IL-10, that induces naive B cells to produce antibodies. The shifting towards TH 2 pattern has recently been associated with an increased tumor metastasisation and a decreased survival in many human and animal neoplasia (fig. 4).12,14,17,18
Table 1
Principal cellular and soluble antitumoral components of innate immune system.
Table 2
Principal antitumoral components of adaptive immune system.
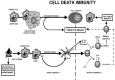
Figure 4
After heat stress the HSPs can induce tumor immunity by two mechanisms: Increasing Antigen presentation through CD91 receptors, or by TLRs receptors.
CD8+, cytotoxic T cells (CTLs) are the major effectors of tumor regression;19 however, CD4+T cells collaborate to their activation. Once activated CTLs do not need costimulation, since MHC-1-bound antigen is sufficient. For eliminating target cells (neoplastic cells) CTLs use three effector molecules: Perforins, Granzyme and Fas ligand. Associated to these killing mechanisms, CTLs secrete specific cytokines, such as: IFN-γ, TNF-α and TNF-β. This pattern of cytokines plays an important role in the activation of macrophages which can exert a direct tumor cytotoxic or, conversely, stimulate tumor progression, depending on the tumor microenvironment.20
Other cells morphologically and functionally distinct, such as Natural Killer cells (NKs), macrophages and neutrophils, use pattern-recognition receptors and other cell-surface molecules to detect tumor cells directly.8,20 Differently from T cells, NKs inhibit tumor growth in a MHC-non restricted manner. Frequently tumor cells (like stressed cells) express on their surfaces different glycoproteins (MICA and MICB) which function as ligands for NKG2D receptors on NK cells. Once activated, these receptors stimulate NK cell activity.21 By contrast, DCs use CD36 and αvβ5 integrin to recognize and phagocytize apoptotic tumor cells. Apoptotic tumor cells in turn release Heat Shock Proteins that, after specific interaction with CD91 receptors on DCs, induce their maturation: thus providing a tailored immune response (See: fig. 1 and fig. 4).22
Tumour Microenvironment as Regulator of Cancer Immunity and Immune Evasion
The switch to an angiogenic phenotype is a fundamental determinant of neoplastic growth and tumor progression. This occurs following the local secretion of specific angiogenic cytokines, especially Vascular Endothelial Growth Factor (VEGF).23-28
Recently VEGF has been demonstrated not only to promote angiogenesis but to suppress anti-tumor immune response principally by hampering leukocyte recruitment29 and by inhibiting CD34+ cell differentiation into dendritic cells.28,30
Tumor immunotherapy success or failure is strongly dependent from leukocyte migration into tumor area.8,31-33 In fact, leukocytes and macrophages, before reaching the target tissue (tumor site), undergo a series of sequential steps during extravasation from blood into tissues: tethering, rolling, adhesion and diapedesis. Among these steps leukocytes adhesion to tumor endothelium is critical and it occurs through the expression of specific adhesion molecules, such as: L-selectin ligands, alpha-4beta-7 integrin adhesion receptors (a4b7) and mucosal addressin cell adhesion molecule-1 (CAM-1). Several animal experiments have shown that in presence of VEGF a significant decreased expression of these adhesion molecules occurs, determining a decline in leukocyte infiltration into the tumors mass.20,29,34-36
Besides, this impaired tumor infiltration by immune competent cells, tumor environment (hypoxia, acidic pH) itself unfavourably modifies T lymphocytes, NK cells and macrophages activity.37 In fact, this kind of tumor environment alters the pattern of secreted cytokines towards an immunosuppressive TH2 pattern, permitting tumour escape from immune surveillance.29,32
Heat Shock Proteins (HSPs), Their Role in Antigen Presentation and in Cancer Immunity
When cells are submitted to a variety of stressful events (e.g., heat, hypoxia, glucose deprivation), there is a rapid and coordinated increase in the expression of a group of proteins, the so-called heat shock proteins (HSPs).1
HSPs are one of the most conserved groups of proteins throughout evolution and are classified into several families according to their molecular weight in kilodaltons (e.g., HSPs 100, 90, 70, s60, s40) and their compartmentalization inside the cell (cytosol or endoplasmatic reticulum, mitochondria).22,38-40 HSPs fulfil different important intracellular processes, such as protein synthesis, folding and they are activated by a specific set of genes induced by different physical stress such as elevated temperature, hypoxia, glucose deprivation and oxidative reagents.39 Linearly at molecular level, heat stress increases the synthesis of HSP 70 until a certain threshold temperature that varies according to cell type. Beyond this threshold temperature their synthesis is inhibited and an exponential cell death follows.41,42
Initially, the role of HSPs, peculiarly of HSP70, appeared to be implicated in the thermotolerance.41 Recently, they have been recognized to activate the immune system becoming a specialized carriers of antigenic peptides in vivo as well.43,44 In fact, Srivastava et coll.44 have established that HSPs are not immunogenic per se, but when they are complexed with antigenic peptides, become powerful immunogens. In fact it has been found that cancer derived HSPs are highly specific and this specificity is associated with the agglomerate HSP/ peptide. Once HSP-complexes (MCH1/MCH2+HSPs) are exposed at the outer surface of cancer cells, they interact with macrophages and dendritic cells through specific surface receptors.40,45 HSP70 binds to the surface of monocytes through the Cluster of Differentiation (CD)14 (CD14) receptor, whereas gp96 binds with the α-2 macroglobulin/LDL receptor related protein or CD91. Furthermore HSP60 has been demonstrated to be a ligand for the Toll-like receptors 4 (TLR4 and TLR2) complex on macrophages.40,46 These data support the data that APCs (macrophages, dendritic cells) have evolved receptors for detecting danger signals (HSPs complexes) released during neoplasia.
The exposure of the HSP chaperoned peptides by the MCH1 and MCH2 molecules to macrophages or dendritic cells triggers a secretion of inflammatory cytokines and costimulatory molecules, such as: IL-6,IL-12; TNF-α, B7 that induce the maturation of DCs towards the TH 1 phenotype.47,48 Their association, with a broad array of peptides generated within cells, make HSPs a good candidate for cancer vaccines.49 In fact HSP-peptide complexes, isolated from a patient's tumor, can be utilized as tailored patient specific antigens, which would avoid the search for specific epitopes. HSPs, isolated from cancer cells but not those derived from normal cells, can generate an immune response, as observed by Tamura and Srivastava.50,51 The potentiality of HSPs in tumor eradication has been validated in more than ten types of tumor models of different histologies and in different animal species, demonstrating that: (a) microgram quantities of HSPs are sufficient to generate substantial immune response;40,45 (b) the immunogenicity of antigens expressed by dying cells occurs via necrosis or apoptosis. Among the two dying mechanisms only necrotic cells or heat stressed cells have been demonstrated to be able to elicit a tumor-specific immunity.52,53 Several non randomised clinical trials with heat shock protein-peptide complexes on human cancers are actually in progress.22
Therapeutic Modalities
Immunotherapy may be either active or passive, specific or nonspecific depending on the process of host immune system stimulation, (see Table 3).16,54,55
Table 3
Classification of cancer immunotherapy.
Passive Nonspecific Immunotherapy (LAK Cells, TIL)
Passive adoptive immunotherapy involves the transfer of immune cells into patients from an external source. This cellular adoptive transfer consists in the generation of cells with antitumor activity obtained in vitro in presence of IL-2. According to their derivation they can generate Lymphokine-Activated Killer Cells (LAK cells) or Tumor Infiltrating Lymphocytes (TIL).
LAK cells are generated by culturing in vitro patient peripheral blood leukocytes with IL-2. These LAK cells are injected back into the patient with IL-2. Adoptive LAK therapy has been applied to advanced cases of renal carcinoma and melanomas with variable efficacy.
TIL cells are generated from mononuclear cells obtained from infiltrates around the tumor after surgical resection. This approach, even if more specific, has yielded only limited success.12
Active and Passive Nonspecific Immunotherapy (Cytokines, IL-2, INF-;α, GM-CSF)
IL-2 has been the first cytokine used alone or in combination with LAK cells for the treatment of different types of metastatic cancers. Patients with metastatic renal cell carcinoma and melanoma receiving IL-2+LAK had a higher rate of complete response. IL-2 has been administered with different regimes and doses demonstrating an elevated toxicity.12-14
Numerous other cytokines have been identified and tried clinically, however only Interferon-α (INF-α) and Granulocyte Macrophage Colony Stimulating Factor (GM-CSF) are currently used given the high toxicity of Interferon- γ and IL-12.8,12,14
Active Specific Immunotherapy (Vaccine, Gene Therapy, Heat Shock Proteins)
Serological identification of antigens by recombinant expression cloning technique (SEREX) has recently permitted to detect more than 1500 TAAs holding a specific antitumor activity.52,56
Tumour vaccination (TV) is a therapeutic form of therapy involving patients with detectable disease and it is used for triggering a robust, appropriate and specific immune response towards a well characterized TAAs, avoiding immune-tolerance and providing a long lasting immune response.52,57 The first tumor vaccines were obtained by irradiating tumor cells. The obtained results were not enthusiastic, inducing several authors to combine tumor vaccines with nonspecific immune modulators, such as BCG, New Castle Disease virus (NDV) and Detox; however the survival rate was comparable to the group treated with chemotherapy. To ameliorate the results, newer vaccines, including allogenic or autologous tumor cells, were genetically manipulated in order to produce a stronger immune response. Tumor cells, were encoded with different cytokines, however only those engineered to produce GM-CSF proved to induce tumor rejection.58
A large number of clinical trials, involving different strategies, are actually being conducted and have been reviewed elsewere.54,58-62
Some of them will however be discussed for a positive interaction with hyperthermia treatment (see Table 7). These are: dendritic cells, gene therapy and heat shock proteins.
Table 7
Effects of WBHT and LHT on different immune treatment modalities.
Dendritic Cells (DCs)
Are a distinct population of leukocytes that play a crucial role as antigen presenting cells and as initiator of antitumor immunity.59 DCs reside mainly in peripheral nonlymphoid tissue, after antigen uptake; however they migrate to the draining lymph node to present antigens to T cells. Preclinical studies have demonstrated that antigen pulsed DCs can generate a potent antitumor activity.63,64 DCs are artificially loaded with antigens by different techniques and can stimulate both innate and adaptive immune system.63,64 Many clinical trials, testing dendritic cell-based vaccines are in progress and have proved a partial clinical efficacy.65,66 In every case, preclinical studies have also demonstrated that the direct intratumoral injection of ex vivo—generated DCs can avoid the need for a tumour antigen loading ex vivo by improving antigen presentation or migration to lymph nodes. A required condition is that injected DCs must be genetically modified in vitro to produce cytokines and chemokines, such as: IL-2, IL-7, IL-12, CD40L, GM-CSF, lymphotactin or secondary lymphoid tissue chemokine (SLC).67,68 The presence of these cytokines may render DCs more able to capture and process TAAs. This overcomes the increased immune suppressive factors (IL-10, VEGF and TGFβ PGE2) present in the tumour medium.69-72
Gene Therapy
Is defined as the method of delivering genetic material into a cell, by altering the cellular phenotype permanently or transiently.73
The efficient and precise targeting of a gene to specific cells or tissues remains the technical hurdle of gene therapy. The delivering methods consist in use of viral or nonviral vectors and in vivo or ex vivo gene therapy approach.74 The ex vivo approach involves the removal of patient's own cells and the readministration after genetic modification. By contrary, in vivo, therapy involves the direct injection of genetic material into patients.
The most promising designed vectors for in vivo therapy are viral vectors. The viral vectors enter into cells by receptor mediated endocytosis and escape from endosomes after DNA delivery to nuclei. They have some disadvantages such as: (a) patient immune recognition of viral proteins with a consequent immune attack and destruction of infected cells, (b) restriction in size and quantity of transgene, (c) inability of repeated viral administration.75,76 Most clinical studies have been carried out using retroviruses or adenoviruses as transfer vector with various advantages and disadvantages.77,78 The targets of viral therapy can be: inactivation of oncogenes (i.e., ras, c-myc, c-erB-2, abl, bcl-2);77 genes involved in tumor progression (i.e., Triple —helix formation); immunomodulatory genes encoding cytokines (TNF-α,IL-2; GM-CSF, INF-γ) costimulatory molecules (i.e., MCH molecules, CD40ligand. B7, Cd28);76 angiogenic factors (i.e., VEGF)70,75 and tumor associated antigen genes.79
Non viral vectors (naked DNA, lipids, liposome) make use of physical methods of gene transfer. They are free of the side effects of viral vectors, but they suffer of specificity.75 Ex vivo gene delivery is the best system for such vectors. Naked DNA in the form of plasmid can be injected into muscle tissues or conjugated with gold particles and bombarded into the tissues. Muscle tissue injection is the most effective delivery method of administration. Repeated injections determine an improvement in therapeutic gene expression but the effect does not last for long.75,77
Another route of DNA administration is the use of lipid vehicles. Two classes of lipid aggregates have been used : (1) cationic lipids [lipoplexes]; (2) stabilized liposomes. Lipoplexes are positively charged and interact with negatively charged DNA forming a stable complex. As outlined by Clark,80 cationic lipids have many benefits, such as: easy and inexpensive way of production, non toxicity and potential of delivering large quantity of polynucleotides into cells. However, in order to develop commercially these vector systems, several barriers must be overcome, especially formulation and stability of manufacture.80 The presence of an excessive number of particles positively charged favour precipitation and aggregation. To circumvent this problem of flocculation, hydrophilic polymers like polyethylene glycol polymers (PEG) have been created.80 These sterically stabilized liposomes (PEG liposomes) allow efficient encapsulation of DNA and oligonucletides. They also permit to prepare well-defined and uniform particles suitable for drug carrier.81,82 Furthermore PEG liposomes show an improvement in half life time due to a reduced renal and cellular cleareance. Also they show an enhanced protection from proteolysis with reduction of toxicity.81,82 Other types of liposomes based on polyethyleneimine (PEI) have proved to be stable to nebulization and to be efficiently delivered as aerosol DNA plasmids to lung parenchyma,83,84 exhibiting a greater concentration into lungs when compared to other route of administration such as intravenous route.84,85
Concluding, vaccine therapy is a tailored specific therapy. However, critical issues to overcome are the choice of the delivery system and the identification of singular epitopes.
Heat Shock Proteins
Meet the request for searching specific epitopes. In fact, they have been implicated at multiple points in the immune response, including initiation of proinflammatory cytokine production, antigen recognition and processing, and phenotypic maturation of DCs.86 A preliminary clinical study on colorectal tumor metastasised to liver treated with autologous HSP-96, has clearly demonstrated important points.86 Namely, HSP-complex induced a significant increase in committed lymphocytes, as response patients have a better clinical outcome as overall survival and as disease free survival and no toxicity was observed.46,87 The importance of hyperthermia in generating HSPs with the aim of immunization will be described later.
Active Non Specific Immune Therapies: Coley Toxins (CTs)
In 1868 W. Busch in Germany concluded that fever induced by certain bacteria from erysipelas can cause tumor regression or cure cancer. This was suggested after the observations on a patient with a soft tissue sarcoma of the neck infected by erysipelas.3,4 The causative agent (streptococcus) at that time was not identified. Subsequently, in 1892, a young American surgeon W. B. Coley, unaware of Busch's findings, observed a regression of soft tissue sarcomas in a patient infected by erysipelas. Stunned by that finding, he searched the medical literature and found many publications confirming his observation.4,5 Coley prepared initially a culture of streptococci that he injected at the tumor site with encouraging results. He even noted that the presence of Serratia Marcenscens could enhance the virulence of streptococci and an injection remote from the tumor site could equally result in tumor regression. After these observations Coley incorporated Serratia Marcenscens into the streptococcal vaccine just obtaining the so called “Coley's toxin” or “Mixed Bacterial Vaccine” (MBV). The intravenous route was the most effective; the toxin dose was considered sufficient only if accompanied by fever (39-40°C). Fever and the sustained pyrexia, now recognized to be elicited by tumor necrosis alpha (TNF-α) and by other cytokines.1,2,3,5 Were considered the critical points in the tumor regression.4,5 It was also observed that those who developed the highest fever were most often the ones with the longest survival.
Coley should be considered the first scientist to apply with a certain continuity, induced hyperthermia (fever) as immune treatment.1,3,6 He noted that tumor regression was obtained only in presence of fever. Since then the interaction between hyperthermia (in whatever way obtained) and immunity has not been completely elucidated.7
To our opinion, a better comprehension of the effects of heat on immunity is obtained by understanding the effects of thermal component of fever on immunity (see Table 4).
Table 4
Effects of thermal component of fever on innate and adaptive immunity.
Effects of Thermal Component of Fever on Immune System
Often, in presence of tumor and microrganisms host replies by increasing body temperature. 1-3
Fever is a complex neuroendocrine adaptive response due to an increase in the set - point temperature regulator found in hypothalamic area.88 After their entry into organism, bacteria or viruses induce macrophages to produce a series of proinflammatory cytokines such as: interleukins (ILs) -1,2,6, tumor necrosis factor alpha (TNF-α), Interferon - α (IFN-α), IFN-γ. These cytokines, with an additional mediator [prostaglandin E2 (PG E2) cyclooxygenase 2 prostaglandins derivate (COX-2)], act on the thermoregulatory area and reset it to a higher level of temperature, producing the febrile response.89-91 Temperature elevation has been suggested to be beneficial for the host.92,93 It is unlikely that evolution has maintained such an expensive defensive metabolic mechanism, without a role.2,3 However, the role of temperature on immune defence is not completely understood. Different studies support the idea that temperature rise has a beneficial role, such as an improved efficiency of macrophage killing activity and an increase on survival in mice infected by herpes virus or with rabies.94-96
Leukocyte adhesion to endothelium and emigration to the site of inflammation are positively affected by heat as the antigen-non specific defence systems (chemotaxis, phagocytosis, complement haemolysis) (see fig. 2 and fig. 3).97 Even antigen-specific activation, proliferation, cytokine expression differentiation and antibody secretion by lymphocytes are affected by temperature. T cell responsiveness to mitogens, IL-1, IL-2 and antigens increase linearly until a temperature of 39°C; beyond this limit a decline is observed.96-98 Furthermore, it has been shown that antibody secretion in vitro is temperature dependent and this effect declines in the absence of T-helper cells.97

Figure 2
Time-dependent exemplary induction of IL-1 in a patient after induction of fever up to 39.8°C with a biological pyrogen (Vaccineurin®).

Figure 3
Emigration, homing, proliferation and activation of leukocytes in a patient after induction of fever up to 39.8°C with a biological pyrogen (Vaccineurin®) depending on time.
A summary of temperature effect on specific and non specific immunity is presented in Table 4. From it you can conclude, that temperature on the order of fever range (39-40°C) is the most favourable ones for the immune response.94,96,97 The effects of hyperthermia on the immune system are complex and pleiotropic and are dependent on temperature, time and microenvironment.
Effects of Induced Thermal Elevation (Hyperthermia) on Immunity
Temperature application above the physiologic range (≥ 42.5°C) has been demonstrated to induce various effects on immune system (see Tables 5, 6).6,7,99-103
Table 5
Effects of WBHT, and LHT on immune response.
Table 6
Effects of fever, WBHT, LHT on cytokines induction and activity.
In Vitro Studies
NK and LAK cell cytotoxicity was determined in a standard 4-h chromium releasing assay using K-562 human erythroleukemic cells as target. Cell viability was measured by exclusion of Trypan Blue dye.104 Some studies have analysed the leukocyte function in presence of temperature associated with IL-2, TNF-α or Interferon.105-111 The purpose was to verify the possible abrogation of the immunosuppressive effects of temperature and the rescue on their activity by these cytokines.112-115
Fuggetta et al115 have evaluated in vitro the influence of hyperthermia (HT) (1h, 42°C) on the cytotoxicity of IL-2 activated NK cells. Hyperthermia reduced the lytic activity of NK cells profoundly. The inhibition of this lytic activity has been demonstrated to be transient and not due to an aptosis but rather to an induced reduction of the effector cells.115 Furthermore these authors, in agreement with others have observed that the heat treatment of target cells alone (K562 and Daudi cells) did not alter their sensitivity to lysis.114
LAK cell cytotoxicity has been studied by Shen et al.116 They have demonstrated that LAK cell induced cytotoxicity was temperature dependent. It enhanced in presence of febrile temperature (≤ 40°C) but decreased by exposure to 1 h at 42°C.117 In accordance, investigations by Singh et coll. have shown that LAK cell cytotoxicity, as with NK cell induced cytotoxicity, decreased at temperature above 38.5 °C. TNF mediated cytotoxicity however was significantly enhanced at 40°C.109 By contrast, Fritz et al have obtained a certain variability on LAK cell mediated lysis in presence of heat and INF-γ.118 From the various studies analysed it appears that the immune suppressive effects of heat on NK and LAK cells become evident at temperatures above 39°C. The response of NK and LAK cells to hyperthermia is temperature and dose dependent and not related to cell viability or to the absolute number of cells.105,115,116 In fact, several authors have noted little loss of cell viability at 39°C,97,98,135 whereas a decrease was demonstrated at temperature above 42°C.99 The lytic function is more heat sensitive than the recognition and binding functions. The extent of recovery of activity after exposure to heat is inversely correlated with the temperature and the recovery time can be complete.119,120 Human and animal cells show a different behaviour during heat exposure. Human and animal NK cells show similar inhibitory behaviour after heat and seem the most sensitive among the immune competent cells. On the contrary, B cells are less affected by heat while murine lymphocytes, compared to the human counterpart, appear to be more heat sensitive.6
In Vivo Studies
Conditions in vitro cannot simulate those in vivo; therefore Kappel et al106 investigated the behaviour of NKs and other immune competent cells of healthy volunteers during whole body hyperthermia (WBHT) at 39.5°C for 2 h in vivo conditions. NK cells cytotoxicity increased in a temperature-dependent way. Cells incubated with IL-2 or INF-α had an enhancement in cytotoxicity and number compared with control values. At temperature above 39.5°C a slow decrease in number of CD3+ was evident with no variations regarding CD19 + cells (B cells).106
More recently, Atanackovic et coll.121 have examined the following immunological parameters during WBH treatment: (A) behavior of CTLs with a panel of Cluster of Differentiation (CD) activation markers; (B) serum cytokines; (C) intracellular cytokine levels (D) capacity of these cells to proliferate.71 They have classified the effects of WBH on patients immunity in different arbitrary phase defined by post treatment time. Immediately after WBH, a drastic increase of NK cells and CD56 CTLs was noted in peripheral blood. This phenomenon was transient and followed, after 3 h post WBH, by a short period of reduced T cell activity, indicated by diminished serum levels of soluble interleukins 2 receptors (sIL-2R). In this first phase, a short-lived increase (for the first 5 hs following treatment) in the serum concentration levels of IL-6 was found with a recovery to normal values after 24h. TNF-α increased significantly during the first 24 h, associated to a marked increase in the peripheral percentage of CTLs and CD56. CTLs, CD56, sIL-2R and lymphocytes expressing CD 69 markers reached their maximal concentration 48 h post WBH. CD69 is an antigen expressed early in the activation of lymphoid cells, and it is considered restricted to activated lymphocytes and undetectable on resting lymphocytes. CD 69 is normally induced, after stimulation, with mitogens or cytokines like INF-α, INF-γ or TNF-α. In agreement, the intracellular concentrations in CD8 cells and in serum of INF-γ and TNF-α were found elevated 24 h post WBH in the 80% of the patients.
Other authors have studied the production of other cytokines in the serum of patients treated with local hyperthermia (LHT) and WBHT or perfusional hyperthermia (PHT). The studies are summarized in Table 6. During LHT no detectable increase in IL-1, IL-6 or TNF-α was observed122 whereas after WBHT serum levels of IL-1 and IL-6 were increased.123 Robins et al124,125 investigated why, during WBHT at 41.8°C, an enhancement of radiation or chemotherapy response was found without a concomitant increase in myelosuppression. For this reason, they studied an expanded panel of serum cytokines in different patients and found an increase of IL-1β, IL-6, IL-8, IL-10, G-CSF and TNF-α within hours after WBHT.93,122,126,127 Moreover, they reported that bone marrow cells stimulated the production of IL-1 β, IL-6 and that TNF-α increased further their plasma levels. This interaction between tumor cells and cytokines, such as interleukin IL-6 resulted in a secondary induction in the bone marrow (BM) of IL-3 and GM-CSF. These factors are produced by BM stromal cells and by T lymphocytes. The plasma levels of the cytokine panel increased 1 h following WBH and diminished after every WBH application reaching the minimun concentration after 4 cycles.127
Alonso et coll. reported a similar cytokine increase with the addiction of IL-2,TGF-β, INF-γ and INF-α in patients undergoing extracorporal perfusion.128 A disagreement between the two groups about the TGF-β production has not been completely clarified. Some other aspects of immune response are affected by WBHT and LHT and are illustrated in Table 5.
The concentration of some adhesion molecules such as ICAM-1, L-selectin and α 4β7 have been demonstrated to be induced and increased both by LHT and WBHT, augmenting the homing effect into the tumor area of lymphocytes, leukocytes, neutrophils and macrophages.106,112,117,129-136
Several authors have tried to verify the possible modification of tumor cell immunogenicity after exposure in vitro to hyperthermia treatment.137,138 Dickson concluded that immunization and survival of mice with Ehrlich ascites did not increase after HT.137 By contrast Mondovì obtained an increase in the immunogenicity and found that heated cells had an higher immunogenicity compared to cells treated with irradiation. The phenomenon was dependent on the length of treatment and the choice of temperature was extremely important.138,139
Recent studies on rat T-9 glioma cells by Ito et coll140 seem to confirm Mondovi's experiments. In fact, these authors have shown a significant increase in MHC class 1 antigen on the surface of heated cells 24 h after heating associated to an increased expression of HSP70. No modifications of other surface immunologic mediators, such as ICAM-1 or MHC class II antigen were noted. The in vivo growth of T-9 glioma cells, using immunocompetent syngeneic rats (F344) was significantly inhibited and associate to a an increased cytotoxic specificity.140
Specific Effects of Hyperthermia on Immune Therapeutic Modalities
Several recent studies, suggest that hyperthermia is suitable as a complementary therapeutic modality for immunotherapy, (see Tables 6 and 7).
Radioimmunotherapy and Monoclonal Antibody Therapy
An enhancement of therapeutic outcome of radio-immunotherapy and monoclonal antibody (MAbs) activity in combination with WBH and LHT respectively has been demonstrated.141-143
The tumor uptake of MAbs against different experimental tumors (colon cancer,142 glioma, breast and prostate carcinomas143) increased from 12% in non heated tumors to 42% in tumors heated with local hyperthermia (41.8°C x 4h) and persisted in the heated tumors over 48 and 96 h. The increased uptake of MAbs obtained using local hyperthermia, seems not so be induced by a decrease in tumor interstitial fluid or modification of kinetic parameters, but rather to an increased extravasation.144-147
Gene Therapy
The use of gene therapy as potential therapeutic method is increasing. Different authors have demonstrated that HT enhances the effect of viral gene vectors encoding IL-12 and the treatment was effective, devoid of systemic side effects and associated to a substantial tumor growth delay, as compared to animals treated without HT.148,149
Mutated p53 genes are found in over 50% of all cancers and they are responsible for the decreased HT induced apoptosis. Adenovirus transfer of p53 associated with HT seems attractive for overcoming this resistance. In fact glioma and human salivary gland adenocarcinoma treated with this combination demonstrated a higher sensitivity to hyperthermia and radiotherapy.150,151
Other useful methods to obtain an efficient gene transduction is the use of liposomes. Several authors have demonstrated that the transduction of DNA plasmid complex (lipoplex) was more efficient under conditions of hyperthermia than at 37°C. An amelioration of this effect has been demonstrated using thermosensitive liposomes or magnetic cationic liposomes, recently developed.134,152,153
Cytokine Therapy
The treatment of tumors with cytokines such as IL-2, TNF-α, INF-α and GM-CSF, has been disappointing. This has induced many researchers to use IL-2, GMCSF, Interferons and TNF-α with WBHT and LHT.154-158 Generally, an additive effect has been demonstrated without an increase in toxicity. The majority of studies have been conducted on animals using IL-2 and TNF-α.159,160,161,162 Human studies have been conducted, primarily with WBH and perfusional hyperthermia (PH) associated to TNF-α.163-167
IL-2 administered before local hyperthermia treatment has demonstrated to be additive and useful to treat mice with lung metastases.116,168 The same additive effect has been demonstrated by Geehan regarding melanoma and sarcoma. The response was obtained using IL-2 simultaneously to WBHT application. The doses and toxicity were lower than those usually reported.161,169 Fritz et al have demonstrated that the potentiation of IL-2 combined with HT is mediated by TNF-α induction. In fact, the effect of IL-2 was abrogated by anti- TNF-α antibodies.160
Whole body hyperthermia or local hyperthermia combined with low-dose IL-2 was more effective on reducing tumor growth than each modality alone, and the response was more pronounced for macroscopic tumor than for microscopic one. Geehan et al suggest that this phenomena is to be ascribed by two factors: (A) selective increase in permeability in tumor vessels, as compared to normal tissues with a consequent accumulation of drug, (B) an augmented expression by HT of intercellular adhesion molecule -1 (ICAM-1) followed by an increased homing into tumor tissue of LAK cells.131,160,161
We refer to the review of Klostergaard et al170 for studies on combination of hyperthermia with TNF-α and INF-α. In brief in vitro and in vivo studies on human and animal tumors indicate a sensitization of TNF-α effect combined with heat. Sensitization was greater when tumor targets were treated with TNF-α prior heating treatment and the effect in vivo can be reached with lower dosage and with less toxicity. It appears that the effect of TNF-α in vivo is partially due to an increase on plasma membrane receptor expression or affinity and on tumor vasculature. Klostergaard et al170 reported similar effect using INF-α and INF-β both in vitro and in vivo. The maximum effect was observed with intratumoral administration and the time of administration with HT was less important. A combination of TNF and INF is possible with additive effect and decreased dosage. As for TNF-α the antiproliferative effect seems to be ascribed to a direct effect on plasma membrane receptor expression or affinity.
Recent studies are more oriented to use TNF-α combined with chemotherapy and WBHT or with limb or organ isolated perfusional HT. As reported by many authors a synergism among hyperthermia, melphalan (L-PAM) and TNF-α in the clinical setting of limb perfusion for malignant melanoma and sarcoma has been demonstrated.171 A TNF-α concentration superior to that achieved by bolus administration (10-20 μg/ml) can be given locally (1-2 μg/ml) associated only to mild toxicity (grade 1or 2) in 25% of patients treated.163,164,172 A similar combination of therapeutic regimen for treatment of unresectable liver malignancies (confined to liver) by using isolated hepatic perfusion (IHP) has been studied by Alexander et al. According to a critical evaluation by these authors IHP with L-PAM and TNF-α is the best combination regimen for obtaining a good response rate as compared to other chemotherapeutic regimens using drugs such as FUDR.166
Effects of Hyperthermia on Lymphocyte Homing
As previous mentioned, leukocyte infiltration into tumor mass is mediated by the expression of various adhesion molecules and cytokines.172,173 Among these adhesion molecules, ICAM-1 and α4β7 integrin are pivotal in regulation of the migration of leukocytes from the blood vessels.20 Their expression has been demonstrated to decrease in presence of VEGF,28,29 to increase in presence of febrile range temperature (38-40°C) and INF-α.136,174 In fact, there is an increasing evidence that local and whole body hyperthermia can enhance both L-selectin lymphocyte-endothelial cell adhesion.130,136,175 Regarding increase of adhesion molecules it appears that hyperthermia differs compared to other therapies. In fact the increase has been detected only on tumors microvasculature and on peritumor lymphatic not on surrounding normal vasculature.176 The mechanism underlying HT control of L-selectin have revealed that febrile temperature do not increase lymphocyte L-selectin surface density or L-selectin dependent recognition of soluble carbohydrates but the avidity of preexisting adhesion molecules for physiologic ligands.176 This up regulation of adhesion process suggests the use of LHT and WBHT in clinical settings for delivering selectively cytotoxic T-cells or gene armed lymphocytes only into the tumor area.
The Danger Model and Hyperthermia-Effects on Dendritic Cell Maturation and Stimulation of Innate-Adaptive Immunity
During the last decade, we have learned a great deal about the molecular mechanisms responsible for the modulation of tumor immunity; however, little advances have been made clinically so far. The reasons for this failure may be the result of several mechanisms:
- not complete control of tumor microenvironment177
- non-appropriate presentation of antigens178
- innate immunity not adequately stimulated or suppressed179
- tumor immune evasion.
Hyperthermia has a role in controlling the first three mechanisms. Inside a tumor mass, it exists at least partially a stressful hypoxic and acidic micro milieu which hampers antigen presentation or immune effectors function.26,180 On one hand, this kind of stressful environment can modify the response of lymphocytes [in vitro] to synthesis and release of mitogens and cytokines during HT treatment,101 on the other part it is the more suitable for killing tumor cells by heat, and for the generation of the “Danger Signal”.178
Danger Model
According to Matzinger180,181 for “Danger Signal” we must intend a tissue damage in general, that may provide a source for initiating protective immune response. Data by Feng et coll.53 indicate that heat stressed tumor cells are capable of providing the necessary danger signals, likely through increasing surface expression of heat shock proteins (HSPs),182,183 resulting in activation and for maturation of dendritic and Natural Killer cells.183,184 Heat stressed apoptotic 12B1-D1 cells, compared to not heat stressed cells, were more effective in stimulating dendritic cells to secrete interleukin-12 (IL-12) and in enhancing their immunostimulatory functions in mixed leukocyte reactions.53 Thus, DCs are able to distinguish between stressed and non stressed cells undergoing programmed cell death. In conclusion, a tumor tissue that undergoes a stress response (i.e., heat) and goes in apoptosis increases the synthesis of stress proteins on their surfaces or releases products during tissue damage that are recognized by tumor-infiltrating lymphocytes as not - self. This may generate potent antitumor T-cell responses.182
In contrast, non stressed apoptotic tumor cells are recognized by the immune system as a physiologic process, critical to normal development and able to elicit only a non-inflammatory or even tolerigenic bland danger signal (fig. 4).
Furthermore, HT stimulates DCs migration,185,186 lymphocyte homing136 and HSPs synthesis. 182,183 DCs emigration from peripheral organs to lymph organs, is crucial for their maturation and their shifting from an antigen-capturing mode to a T cell-sensitising mode.63 In this sense, Ostberg et coll183,186 have observed an enhancement of antigen-dependent immune responses at skin level and the stimulation and emigration of epidermal DCs to draining lymph nodes following WBHT. Concluding, cell death occurring with a concomitant production of HSPs, such as during HT treatment, is highly immunogenic. Tumor immunogenicity is also enhanced in cancer cells over- expressing HSP70 and these cells induce a marked TH1 type immune response compared to cells dying via apoptotic mechanism.187,188,189 In Figure 4, the various mechanisms of cell death and the consequences on tumor immunity are illustrated. It is important to note that apoptosis after macrophage phagocytosis induce the production of the immunosuppressive cytokine IL-10.189 This can permit tumor tolerance.
Innate Immunity Heat Shock Proteins Link
Hyperthermic treatment can induce and elicit a variety of innate immune responses.45 Innate immune responses have been shown to contribute to the control of tumor in mice and there is indirect evidence that it contributes to the control of cancer in humans too.9,179 This response is dependent on the stimulation of TLRs expressed on DCs surface as confirmed by recent studies by Hilf10 and Valubas.190 In fact they have demonstrated that the *ER-resident chaperone Gp96 (*ER: Endoplasmaticum Reticulum) is a potent tumor vaccine in animal models, and it induces both innate and specific immunity in a high efficient way interacting with TLRs pathway.10 Intriguingly, many other HSPs and substances from nonmicrobial sources have been recently shown to signal through TLRs. There are ten members of the TLR family identified to date,40,191 in particular TLR4 is involved in activation by HSP 60, TLR2/4 by HSP 70 and GP96, and TLR2 by necrotic cells.192 Furthermore, recent findings show that TLRs are expressed in a multitude of different cell types, such as APCs, macrophages and DCs. The best characterized regulator of TLR signalling is the NF-kB transcription factor, which controls the expression of many genes involved in the inflammatory response.193,194 This en- gagement triggers the induction of proinflammatory cytokines and chemokines which contribute to the maturation of DCs and the activation of naive T cells (see fig. 4).40 Between the cytokines and chemokines released IL-12, IL-18 and TNF-α have a crucial role in directing TH1 response.17 IL12 stimulates, also, NK cells that have been demonstrated to be required for obtaining an adequate antitumor activity by HSPs. Recent studies by Tournier194 et coll have confirmed that fever plays a role in activation of DCs through the release of different temperature sensitive cytokines. Another confirmation comes from a recent work by Basu et al195 that have demonstrated that elevated temperature in the range of 39.5°C-41°C causes immature DCs to mature, specifically through elevation of intracellular levels of HSP 90. The HSPs released from cells undergoing necrotic death cause translocation of NFkB into the nucleus and maturation of DCs. Concomitantly, the elevations of the aforementioned cytokines (IL-12 and TNFα) contributes further to DC-maturation. Additional study has outlined the importance of DCs maturation as an essential prerequisite for a successful vaccination.183 Studies by Wang et al120 have shown that fever, as wells as, HT combined with tumor derived HSP (HSPs 110 and 70) significantly enhanced the vaccine efficiency on mole-to-mole basis and reduced tumor volume. Okamoto196 and Schuller197 reported that LHT induced tumor-specific CTL response on colon carcinoma and necrosis of hepatocellular carcinoma cells.
Heat shocked activated DCs are more able to stimulate T cells than non heat shocked DCs, indicating that temperature elevation can be exploited to generate a powerful immune - activity. 40,45
Hyperthermia stimulates also neutrophils and macrophages recruitment.106,132 Two cells subset of innate immunity that have demonstrated to play an important role in host defence against tumors.198 As demonstrated by Gough et coll,199 macrophages can distinguish between tumor cells dying through classical apoptosis or cells engineered to die through non apoptotic mechanisms. In a certain sense, the immune system is able to read in which way tumor cells die and to react consequently. Additionally the presence of HSP 70 acts as one component of a bimodal alarm signal that activates macrophages, in the presence of stressful - immunogenic tumor cell killing to become activated in an antitumoral way. Different studies have demonstrated that tumor immunogenicity is enhanced in cancer cells over- expressing HSP70 and these cells induce a marked TH1 type immune response compared to cells dying via apoptotic mechanism (fig. 4).187,189 The danger signal and the TRL activation can work in concert and can augment the tumor immune response. In every case, this mechanisms are to be completely elucidated and clinical demonstrated.
Streptococcal Preparation OK-432 (Picibanil)-Corynebacterium Parvum
Following the abandonment of Coley's Toxin, different authors, unaware of the importance of innate immunity in tumor rejection, demonstrated that cell wall bacteria products such as streptococcal preparation OK432 (Picibanil) and Corynebacterium Parvum, can strengthen tumor lysis cells by lymphocytes when used with hyperthermia.200,201 OK-432 is a lyophilized streptococcal preparation made by penicillin treatment of the Su-strain of A-group streptococcus and it has been used in Japan since 1975 as cancer agent. Picibanil associated with hyperthermia has demonstrated in different animal strain to induce NK cell activity and to enhance tumor tissue response to heat.201,202 Corynebacterium Parvum is an aspecific immunotherapeutic agent constituted by bacterial cell wall glycoproteic and lipid fractions. In presence of hyperthermia it has demonstrated similar immune and thermal effects than OK-432.203-206 For Picibanil an involvement of TLR receptor 4 in its antitumor effect has been recently found demonstrating a link between innate immunity and anticancer activity.206
At the light of these studies, heat can be considered as an aspecific innate immunity booster. The use of bacterial product extracts increase further this stimulation.
Effects of Hyperthermia on Metastatic Process
Metastatic process is the most fearsome aspect of cancer. The formation of metastases is a complex multistep process influenced by the host and by selection and resistance induced by the different therapeutic approaches used.207,208 Shah outlined in animal experiments that a sublethal heating (≤ 42.5°C) of tumors with a non-complete destruction of all cancer cells, may enhance metastases formation, in a way not dissimilar to chemo- and radiotherapy.209 In 1974, studies by Dickson and Ellis210 opened a great controversy on the efficacy of hyperthermia treatment, in fact they reported an increased metastasization to the liver following heating of a Yoshida sarcoma in rats. Further studies reported by Shah209 showed an increased metastasization preferentially to lymph nodes and lungs following a non appropriate heating. A curative treatment with appropriate temperature (≥ 42.5 °C) was associated to regression of primary tumor and was accompanied by increased host immunocompetence. An increase on metastization has been reported by some Authors and attributed to a decreased activity of NK cells.6,7,106 Recent studies using WBHT in humans and animals211 did not found a decreased activity of NKs and CD4 cells in vivo, contradicting old studies in vitro.121 Urano,212 Dickson and Shah6 observed that WBHT may induce metastases at a frequency higher than LHT. Urano et al revealed that the size of the tumor and not its immunogenicity was critical for metastasization during WBHT.212 To reduce this incidence Oda et al213 suggest the use of anticancer drugs associated to WBHT. Dickson and Shah6 reported the abrogation of the effects on immune response generated by curative local heating providing that WBHT was followed or was associated with local hyperthermia. More recent clinical studies on animals with melanoma, treated with Radiation alone or combined with LHT, have demonstrated no significant difference in metastasization between the two groups, though local recurrence was a common event and was associated with metastases appearance.214,215 Similar phase III trial on human melanomas treated using similar design has shown an improved local control and a reduced metastasization with combined RT+LHT.216 A recent randomised study on soft tissue osteosarcoma has demonstrated that the rate of metastasization with thermoradiotherapy is similar to that seen with preoperative radiation therapy alone.215 Similar conclusions about dissemination of malignant cells during WBHT in combination with chemotherapy for epithelial malignancies have been reached by Hegewisch-Becker and collaborators.216
In order to obtain a maximum benefit from tumor heating a functioning host immune response seems necessary. In fact, studies conducted in the late 1990 by Pontiggia et al217 have clearly demonstrated that patients with higher values of inflammatory tests (α2 glycoprotein > 1.0 g/l; high sedimentation rate > 50/1h, and with a lymphocytes count < 500/mc, and a ratio CD4/CD8 < 1.2) failed to obtain remission. The same authors outlined that patients responsive to BRM (i.e., INF-α) show an increase in NK cell number and responders have a better prognosis as compared to non responders.217
Another important phenomena to take into consideration is the abscopal response. It consists in a regression of tumor at other anatomic sites following curative heating of primary tumor. This phenomena has been described in animals and humans.99,213 Disappearance of primary tumor and regression of distant metastases after hyperthermic limb perfusion in both sarcoma and melanoma has been ascribed to a non specific immune reaction, such as inflammatory reaction with an increased macrophage infiltrate.45,96,99,103,106 Clinical studies by Shah209 and Dickson210 have not demonstrated distant metastases regression after primary tumor treatment with LHT. Abscopal phenomena seems not pertinent to WBH treatment.
Conclusions and Comments
Tumor regression of some type of human tumors following HT has been reported. The evidence of an involvement of tumor immune response in this regression due to temperature elevation is increasing step by step.
The effects of hyperthermia on the immune system are pleiotropic.7 First of all, the compartment shifting and homing of immune competent cells (T-lymphocytes) and neutrophils to tumor area is increased whereas cytotoxicity is only transitorily affected.130 The increase of temperature correlates positively with an increased phagocytosis of leukocytes and macrophages within fever range temperatures.127,173 B-cells are activated by heat which brings about an increase of the production of immunoglobulins. On the contrary NK cells cytotoxicity is suppressed and their activity more affected in vitro.116,121,218-220 This suppression is however temporary. In the range of temperature beyond 39° after a period of stunning a complete recovery follows. Some authors have also demonstrated that recovery can be augmented or cytotoxicity partially spared by using interferons220,221 or antioxidants113 such as superoxide dismutase. A summary of the effects of LHT and WBH on LAK cell and NK cells cytotoxicity can be found in Table 8. From these studies some aspects become evident:
- Temperature is crucial.
- WBH affects less the cytoxicity activity of both immunocompetent cells than LHT.
- Times of exposure to heat in vitro studies are not clinically comparable.
Table 8
Effects of WBHT, LHT on cellular immune response.
In fact different parameters can influence lymphocyte reaction in vitro compared to in vivo situation. They are: a) treatment time that is too long (3-4h; 18h) or too short (1/2 h), b) the different lytic E:T ratio, c) the nonuniform distribution of heat easily obtainable in vitro but not in vivo and d) the pH of the medium. Studies by Skeen et al have tried to reproduce tumor pH microenvironment and its effect on lymphocytes. They have demonstrated that the pH of the medium (obtained adding lactic acid) had no effect at 37°C but showed a synergistic impairment with heat at 41,42 and 43°C.101
These examples indicate experiments are to be conducted with appropriate methods for assessing in vitro and in vivo cellular immune response. We also suggest to employ experimental conditions well defined, standardized and nearer the clinical situation for better understanding HT effect on immunity. In particular: pH of medium, Cytokine assay in situ, activation and impairment of cellular response by DCs use. Comprehensive clinical studies on WBHT are to our opinion those of Robins and Atanactovic.103,121,123,125,127
CD4+ / CD8+ lymphocytes ratio does not change in vivo, whereas activation and maturation of Dendritic Cells is positively affected.43 In fact, in animals experiments, febrile range temperature elevation (39.5°C-41°C) have demonstrated to elicit DCs maturation through HSPs induction. Furthermore, for this order of heating WBHT induces a panel of cytokines similar to that induced during fever;221,222 on the contrary LHT is not able to elicit any cytokines production.122 (see Table 6).
The induction of HSPs is rapid and occurs immediately following exposure to only a few degrees above normal physiological temperature stimulation.223 HSPs induction occurs during hyperthermia treatment and represents a simple method for stimulating innate immunity9,40 and for harness a highly tailored cancer immunotherapy eliciting the maturation of Dendritic cells and their recruitment into the tumor area.106,130,132,133 In fact, tumor antigens presentation by HSPs isolated from cancer cells has been shown to induce a specific and individualized protective anti tumor response.87 The tissue specificity of HSPs relies on the fact that they can bind antigenic peptides and improve endocytosis of the chaperoned peptides by APCs, enhancing their ability to stimulate peptide-specific T cells.224,225
Regarding the effects of hyperthermia on metastatic dissemination the conclusions are still controversial and many bias are linked to in vitro and in vivo assays for monitoring cancer immune response. However clinical studies on moderate (fever range) and extreme WBHT seem to provide the evidence for a non significant metastatic spread. Concluding, positive and negative effects on immunity are produced by HT treatment and are summarized in Table 9. A positive association between hyperthermia and the various therapeutic strategies has been demonstrated, suggesting a use in conjunction with a stimulation of a tumor immunity such as : cytokines, monoclonal antibodies, gene therapy, dendritic cells administration (see Tables 6, 7, 8). The recent recognized involvement in innate immune response, increases further the interest of hyperthermia, but increase the regret for the time missed abandoning Coley's Toxin and Mixed Bacterial Vaccine too.
Table 9
Positive and negative effects of hyperthermia on immunity.
References
- 1.
- Papac RJ. Spontaneous regression of cancer. Cancer Treatment Reviews. 1996;22:395–423. [PubMed: 9134002]
- 2.
- Abel U. Spontanremissionen und fieberhafte ErkrantungenIn: Heim ME, Schwarz R,eds.“Spontanremissionen in der Onkologie” 199868–75.
- 3.
- Hobohm U. Fever and cancer. Cancer Immunol Immunother. 2001;50:391–396. [PubMed: 11726133]
- 4.
- Bickels J. Coley toxin: Historical perspective. IMAJ. 2002;4:471–472. [PubMed: 12073431]
- 5.
- Coley-Nauts H, McLaren J. Coley toxins-the first century. “Consensus On Hyperthermia for 1990s” Adv Exp Med Biol. 1990;267:483–500. [PubMed: 2088067]
- 6.
- Dickson JA, Shah SA. Immunologic aspects of hyperthermiaIn: Storm FK, ed.“Hyperthermia in cancer therapy”Boston, MS: G K Hall Publishers,1983487–543.
- 7.
- Dickson JA, Shah SA. Hyperthermia and the immune response in cancer therapy. Cancer Immunol Immunother. 1980;9:1–10.
- 8.
- Dranoff G. Cytokines in cancer pathogenesis and cancer therapy. Nature Reviews Cancer. 2004;4:11–22. [PubMed: 14708024]
- 9.
- Diefenbach A, Raulet DH. The innate immune response to tumors and Ist role in the induction of T-cell immunity. Immunol Reviews. 2002;188:9–21. [PubMed: 12445277]
- 10.
- Hilf N, Singh-Jasuja H, Schild H. The heat shock protein Gp96 links innate and specific immunity. Int J Hyperthermia. 2002;18:521–533. [PubMed: 12537752]
- 11.
- Palucka K, Fay J, Banchereau J. Dendritic cells and tumour immunity. Curr Opin Oncol Endocr Metab Investig Drugs. 1999;1:282–290.
- 12.
- Bremes AJA, Parmiani G. Immunology and immunotherapy of human cancer: Present concepts and clinical developments. Crit Rev Oncol Hematol. 2000;34:1–25. [PubMed: 10781746]
- 13.
- Ben-Efraim S. One hundred years of cancer immunotherapy: A critical appraisal. Tumor Biol. 1999;20:1–24. [PubMed: 9858871]
- 14.
- Davis ID, Jefford M, Parente F. et al. Rational approaches to human cancer immunotherapy. J Leukoc Biol. 2003;73:3–29. [PubMed: 12525559]
- 15.
- Schirrmacher V, Freuerer M, Fournier P. et al. T-cell priming in bone marrow: The potential for long-lasting protective anti-tumor immunity. Trends Mol Med. 2003;9:526–534. [PubMed: 14659467]
- 16.
- Lord EM, Frelinger JC. Tumor immunotherapy: Cytokines and antigen presentation. Cancer Immunology Immunother. 1998;46:75–81. [PubMed: 9558002]
- 17.
- Shurin MR, Lu L, Kalinsky P. et al. TH1/TH2 in cancer, transplantation and pregnancy. Springer Semin Immunopathol. 1999;21:339–359. [PubMed: 10666777]
- 18.
- Nishimura T, Iwakabe K, Sekimoto M. et al. Distinct role of antigen specific T Helper type 1 (Th1) and Th2 cells in tumor eradication in vivo. J Exp Med. 1999;190:617–627. [PMC free article: PMC2195611] [PubMed: 10477547]
- 19.
- Titu LV, Monson JRT, Greenman J. The role of CD8+ T cells in immune responses to colorectal cancer. Cancer Immunology Immunother. 2002;51:235–247. [PubMed: 12070710]
- 20.
- Carlos TM. Leukocyte recruitment at sites of tumour: Dissonant orchestration. J Leukocyte Biol. 2001;70:171–184. [PubMed: 11493608]
- 21.
- Fauriat C, Marcenaro E, Sivori S. et al. Natural Killer Cell-Triggering receptors in patients with acute leukaemia. Leukemia Lymphoma. 2003;44:1683–1689. [PubMed: 14692519]
- 22.
- Hoos A, Levey DL. Vaccination with heat shock protein-peptide complexes: From basic science to clinical applications. Exp Rev Vaccines. 2003;2:369–379. [PubMed: 12903802]
- 23.
- O' ByrneKJ, Dalgeish AG, Browning MJ. et al. The relationship between angiogenesis and the immune response in carcinogenesis and progression of malignant disease. Eur J Cancer. 2000;36:151–169. [PubMed: 10741273]
- 24.
- Vaupel P, Kallinoski F, Okunieff P. Blood flow, oxygen and nutrients supply, and metabolic microenvironment of human tumors, a review. Cancer Res. 1989;49:6449–6465. [PubMed: 2684393]
- 25.
- Folkman J. Tumour angiogenesis: Therapeutic implications. N Engl J Med. 1971;285:1182–1186. [PubMed: 4938153]
- 26.
- Freitas I, Baronzio GF. Tumour hypoxia, reoxygenation and oxygenation strategies: Possible role in photodynamic therapy. J Photochem Photobiol B Biol. 1991;11:3–30. [PubMed: 1791492]
- 27.
- Berges G, Benjamin L. Tumorigenesis and the angiogenic switch. Nat Rev Cancer. 2002;3:401–410. [PubMed: 12778130]
- 28.
- Griffioen AW, Molena G. Angiogenesis: Potentials for pharmacological intervention in the treatment of cancer, cardiovascular diseases, and chronic inflammation. Pharmacol Rev. 2000;52:238–268. [PubMed: 10835101]
- 29.
- Griffioen AW, Selma C, Tromp SC. et al. Angiogenesis modulates the tumour immune response. Int J Exp Pathol. 1998;79:363–368. [PMC free article: PMC3220368] [PubMed: 10319018]
- 30.
- Papetti M, Herman I. Mechanisms of normal and tumour-derived angiogenesis. Am J Physiol Cell Physiol. 2002;282:c947–c970. [PubMed: 11940508]
- 31.
- Alexandroff AB, McIntyre CA, Porter JC. et al. Sticky and smelly issues: Lessons on tumour cell and leukocyte trafficking, gene and immunotherapy of cancer. British Journal of Cancer. 1998;77:1806–1811. [PMC free article: PMC2150337] [PubMed: 9667650]
- 32.
- Carlos TM, Harlan JM. Leukocyte-endothelial adhesion molecules. Blood. 1994;84:2068. [PubMed: 7522621]
- 33.
- Kitayama J, Nagawa H, Yasuhara H. et al. Suppressive effect of basic fibroblast growth factor on trans endothelial emigration of CD4(+) T-lymphocyte. Cancer Res. 1994;54:4729. [PubMed: 7520360]
- 34.
- Barleon B, Sozzani S, Zhou D. et al. Migration of human monocytes in response to Vascular endothelial growth factor (VEGF) is mediated via the VEGF receptor flt-1. Blood. 1996;87:3336–3343. [PubMed: 8605350]
- 35.
- Borgstrom PG, Hughes GK, Hansell P. et al. Leukocyte adhesion in angiogenic blood vessels. J Clin Invest. 1997;99:2246–2253. [PMC free article: PMC508056] [PubMed: 9151798]
- 36.
- Griffioen AW, Damen CA, Martinotti S. Endothelial ICAM-1 expression is suppressed in human malignancies; role of angiogenic factors. Cancer Res. 1996;56:1111. [PubMed: 8640769]
- 37.
- Mantovani A, Sozzani S, Locati M. et al. Macrophage polarization: Tumor associated macrophages as a paradigm for polarized M2 mononuclear phagocytes. Trends Immunol. 2002;23:549–555. [PubMed: 12401408]
- 38.
- Hightower LE. Heat shock, stress proteins, chaperones and proteotoxicity. Cell. 66:191–197. [PubMed: 1855252]
- 39.
- Smith DF, Whitesell L, Katsanis E. Molecular chaperones: Biology and prospects for pharmacological intervention. Pharmacol Reviews. 1998;50:494–513. [PubMed: 9860803]
- 40.
- Manjili MH, Wang XY, Park J. et al. Immunotherapy of cancer using Heat shock proteins. Frontiers Bioscience. 2002;7:d43–d52. [PubMed: 11779704]
- 41.
- Li GC, Mivechi NF, Weitzel G. Heat shock proteins, thermotolerance and their relevance to clinical hyperthermia. Int J Hyperthermia. 1995;11:459–488. [PubMed: 7594802]
- 42.
- Fortin A, Raybaud-Diogène H, Têtu B. et al. Overexpression of the 27 KDA heat shoch protein is associated with thermotolerance and chemoresistance but not with radioresistance. Int J Radiation Oncology Biol Phys. 2000;46:1259–1266. [PubMed: 10725639]
- 43.
- Wallin RPA, Lundqvist A, More SH. et al. Heat shock proteins as activators of the innate immune system. Trends Immunol. 2002;23:130–135. [PubMed: 11864840]
- 44.
- Przepiorka D, Srivastava PK. Heat shock protein—peptide complexes as immunotherapy for human cancer. Mol Med Today. 1998;4(11):478–84. [PubMed: 9857367]
- 45.
- Manjili MH, Wang XY, Park J. et al. Cancer immunotherapy: Stress proteins and hyperthermia. Int J Hyperthermia. 2002;18:506–520. [PubMed: 12537751]
- 46.
- Wells A, MalKovsky M. Heat shock proteins, tumor immunogenicity and antigen presentation: An integrated view. Immunology Today. 2001;21:129–132. [PubMed: 10689300]
- 47.
- Milani Y, Noessner E, Ghose S. et al. Heat shock protein 70 in antigen presentation and immune stimulation. Int J Hyperthermia. 2002;18:563–575. [PubMed: 12537755]
- 48.
- Flohe SB, Bruggemann J, Lendemans S. et al. Human heat shock protein 60 induces maturation of dendritic cells versus a TH1-promoting phenotype. J Immunol. 2003;170:2340–2348. [PubMed: 12594256]
- 49.
- Liu B, DeFilippo AM, Li Z. Overcoming immune tolerance to cancer by heat shock protein vaccines. Molecular Cancer Therapeutics. 2002;1:1147–1151. [PubMed: 12481439]
- 50.
- Tamura Y, Peng P, Lui K. et al. Immunotherapy of tumors with autologous tumor - derived heat shock protein preparations. Science. 1995;269:117–120. [PubMed: 9311915]
- 51.
- Srivastava P. Purification of heat shock protein-peptide complexes for use in vaccination against cancers and intracellular pathogens. Methods. 1997;12:165–171. [PubMed: 9184380]
- 52.
- Basu S, Binder RJ, Suto R. et al. Necrotic not apoptotic cell death release heat shock proteins, which deliver a partial maturation signal to dendritic cells and activate the NF-kB pathway. International Immunology. 2000;12:1539–1546. [PubMed: 11058573]
- 53.
- Feng H, Zeng Y, Whitesell L. et al. Stressed apoptotic tumor cells express heat shock proteins and elicit tumor specific immunity. Blood. 2001;197:3505–3512. [PubMed: 11369644]
- 54.
- Horizons in Cancer Therapeutics. From Bench to bedsides. 2001;2(n1)
- 55.
- Rosenberg SA. Progress in the development of immunotherapy for the treatment of patients with cancer. J Intern Med. 2001;250:462–475. [PMC free article: PMC2413437] [PubMed: 11902815]
- 56.
- Sahin U, Türeci Φ, Pfreudschuh M. Serological identification of human tumor antigens. Curr Opin Immunol. 1997;9:709–716. [PubMed: 9368781]
- 57.
- Bitton RJ, Guthmann MD, Gabri MR. et al. Cancer Vaccines: An update with special focuses on gangliosides antigens (Review). Oncology Rep. 2002;9:267–276. [PubMed: 11836591]
- 58.
- Ockert D, Schmitz M, Hampl M. et al. Advances in cancer immunity. Immunol Today. 1999;20:63–65. [PubMed: 10098323]
- 59.
- Espinoza-Delgado I: Cancervaccine. The Oncologist. 2002;7(suppl 3):s20–s33. [PubMed: 12165652]
- 60.
- Ribas A, Butterfield LH, Glaspy JA. et al. Current development in cancer vaccines and cellular immunotherapy. J Clin Oncol. 2003;21:2415–2432. [PubMed: 12805342]
- 61.
- Osanto S. Vaccine trials for the clinician: Prospects for tumor antigens. The Oncologist. 1997;2:284–299. [PubMed: 10388061]
- 62.
- Parmiani G, Pilla L, Castelli C. et al. Vaccination of patients with solid tumours. Annals of Oncology. 2003;14:817–824. [PubMed: 12796017]
- 63.
- Fernandez NC, Lozier A, Flament C. et al. Dendritic cells directly trigger NK cell functions: Cross-talk relevant in innate antitumor immune response. Nat Med. 1999;5:405–411. [PubMed: 10202929]
- 64.
- Celluzzi CM, Mayordromo JI, Storkus WJ. et al. Peptide pulsed dendritic Cells induce antigen - specific CTL-mediated protective antitumor activity. J Exp Med. 1996;183:283–287. [PMC free article: PMC2192396] [PubMed: 8551233]
- 65.
- Ribas A, Butterfield LH, Glaspy JA. et al. Cancer immunotherapy using gene- modified dendritic cells. Curr Gene Ther. 2002;2:57–78. [PubMed: 12108974]
- 66.
- Weber J, Fong L. Clinical trials of Dendritic Cells in cancerIn: Lotze M, Thomson AW, eds.“Dendritic Cells”San Diego, San Francisco: Academic Press,2001561–571.
- 67.
- Haupt K, Roggendorf M, Mann K. The potential of DNA vaccination against tumour-associated antigens for antitumor therapy. Exp Biol Med. 2002;227:227–237. [PubMed: 11910045]
- 68.
- Pawelec G, Rees R, Kiessling R. et al. Cells and Cytokines in immunotherapy and gene therapy of cancer. Crit Rev Oncogenesis. 1999;10:83–127. [PubMed: 10327211]
- 69.
- Baar J. Clinical applications of dendritic cell cancer vaccines. The Oncologist. 1999;4:140–144. [PubMed: 10337385]
- 70.
- Gabrilovich DI, Chen HL, Girgis KR. et al. Production of vascular endothelial factor by tumour inhibits the functional maturation of dendritic cells. Nat Med. 1996;2:1096–1103. [PubMed: 8837607]
- 71.
- Yamaguchi Y, Tsumura H, Miwa M. et al. Contrasting effects of TGF-β1 and TNF-α on the development og dendritic cells from progenitors in mouse bone marrow. Stem Cells. 1997;15:144–153. [PubMed: 9090791]
- 72.
- Sharma S, Stolina M, Yang SC. et al. Tumor cycloxygenase 2 -dependent suppression of dendritic cell function. Clin Cancer Res. 2003;9:961–968. [PubMed: 12631593]
- 73.
- Romano G. Gene transfer in experimental medecine. Drugs New Perspect. 2003;16:267–276. [PubMed: 12942157]
- 74.
- Peng K-W. Strategies for targeting therapeutic gene delivery. Molecular Medicine Today. 1999;5:448–453. [PubMed: 10498913]
- 75.
- Romano G, Pacilio C, Giordano A. Gene transfer technology in therapy. Current applications and future goals. Stem Cells. 1999;17:191–202. [PubMed: 10437982]
- 76.
- Navarro JG, Curiel DT, Douglas JT. Gene therapy of cancer. European Journal of Cancer. 1999;35:867–885. [PubMed: 10533466]
- 77.
- Kufe DW, Advani S, Weichselbaum R. Principles of gene therapyIn: Bast, Kufe, Pollock, Weichselbaum, Holland, Frei, eds.“Cancer Medicine”Hamilton, Ontario: BC Decker Publisher,2000876–890.
- 78.
- Rochlitz CF. Gene therapy of cancer. Swiss Med Wkly. 2001;131:4–9. [PubMed: 11205184]
- 79.
- Farzaneh F, Trefzer U, Sterry W. et al. Gene therapy of cancer. Immunology Today. 1998;19:294–296. [PubMed: 9666599]
- 80.
- Clark RP, Hersh EM. Cationic lipid-mediated gene transfer: Current concepts. Curr Opin Mol Ther. 1999;1:158–76. [PubMed: 11715940]
- 81.
- Davis ME. Non -viral gene delivery systems. Curr Opin Biothecnol. 2002;13:128–131. [PubMed: 11950563]
- 82.
- Maurer N, Fenske DB, Cullis PR. Development in liposomal drug delivery systems. Expert Opin Biol Ther. 2001;1:923–947. [PubMed: 11728226]
- 83.
- Boulikas T. Liposome DNA delivery and uptake by cells. Oncology Reports. 1996;3:989–005. [PubMed: 21594495]
- 84.
- Koshkina NV, Agoulnik I, Melton SL. et al. Biodistribution and pharmacokinetics of aerosol and intravenously administered DNA-polyethyleneimine complexes: Optimisation of pulmonary delivery and retention. Molecular Therapy. 2003;8:249–254. [PubMed: 12907147]
- 85.
- Densmore CL. The reemergence of aerosol gene delivery: A viable approach to lung cancer therapy. Current Cancer Drug Targets. 2003;3:273–284. [PubMed: 12871058]
- 86.
- Singh-Jasuja H, Hilf N, Scherer HU. et al. The heat shock protein gp96: A receptor-targeted cross-priming carrier and activator of dendritic cells. Cell Stress Chaperones. 2000;5(5):462–70. [PMC free article: PMC312878] [PubMed: 11189453]
- 87.
- Mazzaferro V, Coppa J, Carraba M. et al. Vaccination with autologous derived Heat shock protein gp96 after liver resection for metastatic colorectal cancer. Clin Cancer Res. 2003;9:3235–3245. [PubMed: 12960108]
- 88.
- Saper CB, Breder CD. The neurologic basis of fever. N Engl J Med. 1994;30:1880–1886. [PubMed: 7832832]
- 89.
- Dinarello CA. Cytokines as endogenous pyrogens. The Journal of Infectious Diseases. 1999;179(suppl 2):s294–s304. [PubMed: 10081499]
- 90.
- Netea MG, Kulberg BJ. Circulating cytokines as mediators of fever. The Journal of Infectious Diseases. 1999;179(suppl 2):s178–s184. [PubMed: 11113021]
- 91.
- Blatteis CM, Sehic E. Cytokines and fever. Ann NY Acad Sci. 1998;840:608–618. [PubMed: 9629288]
- 92.
- Ahlers O, Boehnke T, Kerner T. et al. Induced hyperthermia causes significant changes in lymphocytes. Crit Care. 1998;2(suppl 1):abstract p002.
- 93.
- Jampel HD, Duff GW, Gershon RK. et al. Fever and immuneregulation. J Exp Med. 1983;157:1229–1238. [PMC free article: PMC2186992] [PubMed: 6220108]
- 94.
- Hanson DF. Fever, temperature, and the immune response. Ann NY Acad Sci. 1997;813:453–464. [PubMed: 9100921]
- 95.
- Bell JF, Moore GF. Effects of high ambient temperature on various stage of rabies virus infection in mice. Infect Immun. 1974;10:510–515. [PMC free article: PMC422983] [PubMed: 4426698]
- 96.
- Hasday JD. The influence of temperature on host defensesIn: Mackowiak PA, ed.“Fever: Basic mechanisms and management”. 2nd edPhiladelphia: Lippincott-Raven Publishers,1977177–196.
- 97.
- Roberts NJ. Impact of temperature elevation on immunologic defences. RID. 1991;13:452–472. [PubMed: 1866550]
- 98.
- Lederman HM, Brill CR, Murphy PA. Interleukin-1 driven secretion of interleukin-2 is highly temperature dependent. J Immunol. 1987;138:3808–3811. [PubMed: 3495573]
- 99.
- Gautherie M. Hyperthermia and the immune systemIn: Gautherie M, ed.“Whole Body Hyperthermia: Biological and clinical aspects”Berlin-Heidelberg-New York: Springer- Verlag,19926–15.
- 100.
- Dickson JA, Shah SA. Hyperthermia: The immune response and Tumour metastasis. Natl Cancer Inst Monogr. 1982;61:183–192. [PubMed: 7177177]
- 101.
- Skeen MJ, McLaren JR, Olkowski ZL. Influences of hyperthermia on immunological functionsIn: Anghileri L, Robert J eds.“Hyperthermia in Cancer Treatment”CRC Press,198693–105.
- 102.
- Repasky E, Issels R. Physiological consequences of hyperthermia: Heat, heat shock proteins and the immune response. Int J Hyperthermia. 2002;18:486–488. [PubMed: 12537749]
- 103.
- Bull JM, Lees DE, Schuette WH. et al. Immunological and physiological responses to whole-body hyperthermia. Natl Cancer Inst Monogr. 1982;61:177–181. [PubMed: 7177176]
- 104.
- Roberts NJ, Lu ST, Michaelson SM. Hyperthermia and human leucocyte functions: DNA, RNA, and total protein synthesis after exposure to < 41° or >42.5° hyperthermia. Cancer Res. 1985;45:3076–3082. [PubMed: 2408744]
- 105.
- Knox JD, Mitchel RE, Brown DL. Effects of hyperthermia on microtubule organization and cytolytic activity of murine cytotoxic T lymphocytes. Exp Cell Res. 1991;194:275–283. [PubMed: 2026179]
- 106.
- Lamon EW, Parrish J, Marshall G. et al. Synergistic induction of thermotolerance in murine natural killer cells by interferon α and mild heat shock. Radiat Res. 1994;139:364–369. [PubMed: 8073121]
- 107.
- Kappel M, Stadeager C, Tvede N. et al. Effects of in vivo hyperthermia on natural killer cell activity, in vitro proliferative responses and blood mononuclear cell subpopulations. Clin Exp Immunol. 1991;84:175–180. [PMC free article: PMC1535372] [PubMed: 2015709]
- 108.
- Yang H, Mitchel RE. Hyperthermic inactivation, recovery and induced Thermotolerance of human natural killer cell lytic function. Int J Hyperthermia. 1991;7:35–49. [PubMed: 2051075]
- 109.
- Huang YH, Haegerstrand A, Frostergärd. Effects of in vitro hyperthermia on proliferative responses and lymphocyte activity. Clin Exp Immunol. 1996;103:61–66. [PMC free article: PMC2200318] [PubMed: 8565288]
- 110.
- Singh VK, Biswas S, Pandey CM. et al. Effect of elevated temperature on cytotoxic effector cells. Pathobiology. 1996;64:150–155. [PubMed: 8910924]
- 111.
- Azokar J, Yunis EJ, Essex M. Sensitivity of human natural killer cells to hyperthermia. Lancet. 1982;i:16–17. [PubMed: 6172681]
- 112.
- Kalland T, Dahlquist I. Effects of in vitro hyperthermia on human natural killer cells. Cancer Res. 1983;43:1842–1846. [PubMed: 6831421]
- 113.
- Kraybill WG, Olenki T, Evans S. et al. A phase 1 study of fever-range whole body hyperthermia (FR-WBH) in patients with advanced solid tumours: Correlation with mouse models. Int J Hyperthermia. 2002;18:253–266. [PubMed: 12028640]
- 114.
- Yoshioka A, Miyachi Y, Toda K. et al. Effects of local hyperthermia on natural killer activity in mice. Int J Hyperthermia. 1990;6:261–267. [PubMed: 2324568]
- 115.
- Fuggetta MP, Alvino E, Tricarico M. et al. In vitro effect of hyperthermia on natural cell-mediated cytotoxicity. Anticancer Research. 2000;20:1667–1672. [PubMed: 10928089]
- 116.
- Shen R-N, Li Lu, Young P. et al. Influence of elevated temperature on natural killer cell activity, lymphokine-activated killer cell activity and lectin-dependent cytotoxicity of human umbilical cord blood and adult blood cells. Int J Radiation Oncology Biol Phys. 1994;29:821–826. [PubMed: 8040029]
- 117.
- Evans SS, Bain MD, Wang WC. Fever -range hyperthermia stimulates α4β7 integrin-dependent lymphocyte-endothelial adhesion. Int J Hyperthermia. 200;16:45–59. [PubMed: 10669316]
- 118.
- Fritz KL, Koziol BS, Fabian DF. et al. Variable modulation of LAK cell mediated cytotoxicity and ICAM-1 expression by interferon-gamma and hyperthermia in human melanoma cell lines. Electronic Journal of Oncology. 1999;2:89–110.
- 119.
- Dinarello CA, Dempsey RA, Allegretta M. et al. Inhibitory effects of elevated temperature on human cytokine production and Natural Killer activity. Cancer Res. 1986;46:6236–6241. [PubMed: 2430693]
- 120.
- Wang X-Y, Kazim E.A, Repasky A. et al. Characterization of hsp 110 and glucose regulated protein 170 as cancer vaccine and the effect of fever -range hyperthermia on vaccine activity. J Immunol. 2001;166:490–497. [PubMed: 11123328]
- 121.
- Atanackovic D, Nierhaus A, Neumeier et al. 41.8 °C whole body hyperthermia as an adjunct to chemotherapy induces prolonged T cell activation in patients with various malignant diseases. Cancer Immunol Immunother. 2002;51:603–613. [PubMed: 12439605]
- 122.
- Schell SR, Wessels FJ, Abouhamze A. Pro-and Antinflammatory cytokine production after Radiofrequency ablation of unresectable tumors. J Am Coll Surg. 2002;195:774–781. [PubMed: 12495309]
- 123.
- Haveman J, Geerdink AG, Rodermond HM. Cytokine production after whole body and localized hyperthermia. Int J Hyperthermia. 1996;12:791–800. [PubMed: 8950159]
- 124.
- Robins HI, Kurz M, Wiedemann GJ. et al. Cytokine induction by 41.8 degree °C whole body hyperthermia. Cancer Lett. 1995;97:195–201. [PubMed: 7497463]
- 125.
- D'Oleire F, Schmitt CL, Robins HI. Cytokine induction in humans by 41,8 °C whole body hyperthermia. JNCI. 1993;85:833–8434. [PubMed: 7683730]
- 126.
- Robins HI, Grosen E, Katschinski DM. et al. Whole body hyperthermia induction of soluble tumor necrosis factor receptors: Implications for rheumatoid disease. J Rheumatol. 1999;26:2513–2516. [PubMed: 10606355]
- 127.
- Katschinski DM, Wiedemann GJ, Robins HI. et al. Whole body hyperthermia cytokine induction; a review and unifying hypothesis for myeloprotection in the setting of cytotoxic therapy. Cytokine and Growth factor Review. 1999;10:93–97. [PubMed: 10743501]
- 128.
- Alonso K. Cytokine levels in adult patients with solid tumors undergoing whole body hyperthermia (WBH). Int J Hyperthermia. 1997;13:559562. [PubMed: 9354940]
- 129.
- Morita M, Kuwano H, Arakri K. et al. Prognostic significance of lymphocyte infiltration following preoperative chemoradiotherapy and hyperthermia for esophageal cancer. Int J Radiation Oncology Biol Phys. 2001;49:1259–1266. [PubMed: 11286832]
- 130.
- Midis GP, Fabian DF, Lefor A. Lymphocyte migration to tumors after hyperthermia and immunotherapy. J Surgical Research. 1992;52:530–536. [PubMed: 1619922]
- 131.
- Lefor AT, Foster CE, Sartor W. et al. Hyperthermia increases intercellular adhesion molecule-1 expression and lymphocyte adhesion to endothelial cells. Surgery. 1994;116:214–221. [PubMed: 7914035]
- 132.
- Kappel M, Khazami A, Nielsen H. et al. Modulation of the counts and functions of neutrophils and monocytes under in vivo hyperthermia conditions. Int J Hyperthermia. 1994;10:165–173. [PubMed: 8064178]
- 133.
- Hasday JD, Garrison A, Singh IS. et al. Febrile-range hyperthermia augments pulmonary neutrophil recruitment and amplifies pulmonary oxygen toxicity. Am J Pathol. 2003;162:2005–2017. [PMC free article: PMC1868125] [PubMed: 12759256]
- 134.
- Ostberg JR, Repasky EA. Comparison of the effects of two different whole body hyperthermia protocols on the distribution of murine leukocyte populations. Int J Hyperthermia. 2000;16:29–43. [PubMed: 10669315]
- 135.
- Dong BW, Zhang J, Liang P. et al. Sequential pathological and immunologic analysis of percutaneous microwave coagulation therapy of hepatocellular carcinoma. Int J Hyperthermia. 2003;19:119–133. [PubMed: 12623635]
- 136.
- Shah A, Ungerb E, Bain MD. et al. Cytokines and adhesion molecule expression in primary human endothelial cells stimulated with fever - range hyperthermia. Int J Hyperthermia. 2002;18:534–551. [PubMed: 12537753]
- 137.
- Dickson JA, Jasiewicz ML, Simpson AC. Immunogenicity of ascites tumor cells following in vitro hyperthermia. Natl Cancer Inst Monogr. 1982;61:235–238.
- 138.
- Mondovì B, Santoro SA, Strom R. et al. Increased immunogenicity of Ehrlich Ascites cells after heat treatment. Cancer. 1972;30:885–888. [PubMed: 5079432]
- 139.
- Moricca G, Cavaliere R, Caputo A. Hyperthermic treatment of tumors: Experimental and clinical observationsIn: Rentchnick P et al, eds.Recent results in cancer researchBerlin, Heidelberg, New York: Springer,197759112–152. [PubMed: 268661]
- 140.
- Ito A, Masashige S, Honda H. et al. Augmentation of MHC class I antigen presentation via heat shock protein expression by hyperthermia. Cancer Immunol Immunother. 2001;50:515–522. [PubMed: 11776373]
- 141.
- Shen RN, Hornback NB, Shidnia H. et al. Whole-body hyperthermia decreases lung metastases in chimeric monoclonal antibody in a subcutaneous xenograft model. Clin Cancer Res. 1997;3:63.
- 142.
- Saga T, Sakahara H, Nakamoto Y. et al. Enhancement of the therapeutic outcome of radioimmunotherapy by combination with whole - body mild hyperthermia. Eur J Cancer. 2001;37:1429–1434. [PubMed: 11435076]
- 143.
- Hauck ML, Dewhirst MW, Bigner DD. et al. Local hyperthermia improves uptake of a chimeric monoclonal antibody in a subcutaneous Xenograft model. Clinical Cancer Res. 1997;3:63–70. [PubMed: 9815539]
- 144.
- Hauck ML, Zalutsky MR. The effects of local hyperthermia on the catabolism of a radioiodinated chimeric monoclonal antibody. Clinical Cancer Research. 1998;4:2071–2077. [PubMed: 9748121]
- 145.
- Kong G, Braun RD, Dewhirst MW. Characterization of the effect of hyperthermia on nanoparticle extravasation from tumor vasculature. Cancer Res. 2001;61:3027–3032. [PubMed: 11306483]
- 146.
- Hauck ML, Coffin DO, Dodge RK. et al. A local hyperthermia treatment which enhances antibody uptake in glioma xenograft model does not affec tumour interstitial fluid pressure. Int J Hyperthermia. 1997;13:307–316. [PubMed: 9222813]
- 147.
- Hauck ML, Dewhirst MW, Zalusky MR. The effects of clinically relevant hyperthermic temperatures on the kinetic binding parameters of a monoclonal antibody. Nucl Med Biol. 1996;23:551–557. [PubMed: 8832714]
- 148.
- Lohr F, Kang H, Huang Q. et al. Enhancement of radiotherapy by hyperthermia-regulated gene therapy. Int J Radiation Oncology Biol Phys. 2000;48:1513–1518. [PubMed: 11121657]
- 149.
- Huang Q, Hu JK, Lohr F. et al. Heat induced gene expression as a novel targeted cancer gene therapy strategy. Cancer Res. 2000;60:3435–3439. [PubMed: 10910053]
- 150.
- Asaumi JI, Higuchi Y, Murakami J. et al. Thermoradiotherapy combined with p53 therapy of human salivary gland adenocarcinoma cell line. Oncology Reports. 2003;10:71–74. [PubMed: 12469147]
- 151.
- Okamoto K, Shinoura N, Egawa N. et al. Adenovirus-mediated transfer of P53 augments hyperthermia-induced apoptosis in U251 glioma cells. Int J Radiation Oncology Biol Phys. 2001;50:525–531. [PubMed: 11380242]
- 152.
- Yanase M, Shinkai M, Honda H. et al. Antitumor immunity induction by intracellular hyperthermia using magnetite cationic liposomes. Jpn J Cancer Res. 1998;89:775–782. [PMC free article: PMC5921890] [PubMed: 9738985]
- 153.
- Mushiake H, Aoe M, Washio K. et al. Enhancement of gene transduction efficiency in cancer cells using cationic liposomes with hyperthermia. Acta Med Okayama. 2002;56:35–42. [PubMed: 11873943]
- 154.
- Yamauchi N, Watanabe N, Maeda M. et al. Mechanism of synergistic Cytotoxic effect between tumour necrosis factor and hyperthermia. Jpn J Cancer Res. 1992;83:540–545. [PMC free article: PMC5918849] [PubMed: 1319987]
- 155.
- Lin JC, Park J, Song W. Combined treatment of IL-1α and TNF- α potentiates the antitumor effect of hyperthermia. Int J Hyperthermia. 1996;12:335–344. [PubMed: 9044903]
- 156.
- Payne J. Mild hyperthermia modulates biological activity of interferons. Int J Hyperthermia. 2000;16:492–507. [PubMed: 11129261]
- 157.
- Takada Y, Sato EF, Nakajima T. et al. Granulocyte-colony stimulating factor enhances anti-tumor effect of hyperthermia. Int J Hyperthermia. 2000;16:275–286. [PubMed: 10830589]
- 158.
- Lans TE, Bartlett DL, Libutti SK. et al. Role of tumor necrosis factor on toxicity and cytokine production after isolated hepatic perfusion. Clin Cancer Res. 2001;7:784–790. [PubMed: 11309322]
- 159.
- Shen R-N, Lu L, Wu B. et al. Effects of interleukin 2 treatment combined with local hyperthermia in mice inoculated with lewis lung carcinoma cells. Cancer Res. 1990;50:5027–5030. [PubMed: 2379168]
- 160.
- Fritz KL, Koziol S, Dagmar FF. et al. Tumor necrosis factor α mediates the antitumor effect of combined Interleukin-2 and whole body hyperthermia. J Surgical Res. 1996;60:55–60. [PubMed: 8592432]
- 161.
- Geehan D, Fabian D, Lefor A. Immunotherapy and whole body hyperthermia as combined modality treatment of a subcutaneous murine sarcoma. J Surg Oncol. 1993;53:180–183. [PubMed: 8331940]
- 162.
- Van der Zee J, Van den Aardweg GJMJ, Van Rhoon GC. et al. Thermal enhancement of both tumor necrosis factor alpha-induced systemic toxicity and tumour cure in rats. Br J Cancer. 1995;71:1158–1162. [PMC free article: PMC2033841] [PubMed: 7779705]
- 163.
- Robins HI, Katschinski DM, Longo W. et al. A pilot study of melphalan, tumour necrosis-α and 41.8°C whole-body hyperthermia. Cancer Chemother Pharmacol. 1999;43:409–414. [PubMed: 10100597]
- 164.
- Robins HI, D'Oleire F, Kutz M. et al. Cytotoxic interactions of tumor necrosis factor, melphalan and 41.8°C hyperthermia. Cancer Letters. 1995;89:55–62. [PubMed: 7882302]
- 165.
- Eggermont AMM, Koops HS, Klausner JM. et al. Isolated limb perfusion with tumour necrosis factor alpha and chemotherapy for advanced extremity soft tissue sarcomas. Seminars in Oncology. 1997;24:547–555. [PubMed: 9344321]
- 166.
- Alexander HR, Bartlett DL, Libutti SK. Current status of isolated perfusion with or without necrosis factor for the treatment of unresectable cancers confined to liver. The Oncologist. 2000;5:416–424. [PubMed: 11040278]
- 167.
- Sumida M, Isawa E, Kobayashi K. et al. TNF-α and endotoxin levels in cancer patients undergoing intraperitoneal hyperthermic perfusion. Int J Hyperthermia. 1996;12:607–615. [PubMed: 8886888]
- 168.
- Strauch ED, Fabian DF, Turner J. et al. Combined hyperthermia and immunotherapy treatment of multiple pulmonary metastases in mice. Surg Oncol. 1994;3:45–52. [PubMed: 8186870]
- 169.
- Geehan D, Fabian D, Lefor A. Combined local hyperthermia and immunotherapy treatment of an experimental subcutaneous murine melanoma. J Surg Oncol. 1995;59:35–39. [PubMed: 7745975]
- 170.
- Klostergaard J, Tomasovic SP. Hyperthermia and biological response modifiersIn: Urano M, Douple E, eds.Hyperthermia and OncologyThe Netherlands: VSP,19944219–258.
- 171.
- Van Der Veen AH, Ten HagenTLM, DeWilt JHW. et al. An overview on the use of. Anticancer Research. TNF-α Our experience with regional administration and developments towards new opportunities for systemic application;2000;20:3467–3474. [PubMed: 11131649]
- 172.
- Sartor WM, Kyprianou N, Fabian DF. et al. Enhanced expression ICAM-1 in a murine fibrosarcoma reduces tumour growth rate. Journal of Surgical Research. 1995;59:66–74. [PubMed: 7630139]
- 173.
- Burd R, Dziedzic TS, Xu Y. et al. Tumour cell apoptosis, lymphocyte recruitment and tumor vascular changes are induced by low temperature, long duration (Fever -like) whole body hyperthermia. J Cell Physiol. 1998;177:137–147. [PubMed: 9731754]
- 174.
- Evans SS, Wang WC, Bain MD. et al. Fever range hyperthermia dynamically regulates lymphocyte delivery to high endothelial venules. Blood. 2001;97:2727–2733. [PubMed: 11313264]
- 175.
- Evans SS, Frey M, Scheider DM. et al. Regulation of leukocyte-endothelial cell interaction in tumor immunityIn: Mihich and Croce, eds.Biology of TumorsPlenum Press,1998(Ch 20)273–286.
- 176.
- Menoret A, Srivastava PK. The cancer microenvironment and its impact on immune response to cancerIn: Liu Y, ed.“Molecular approaches to tumor immunotherapy”Singapore, New jersey, London, Hong Kong: Word Scientific press,1998109–121.
- 177.
- Forsdyke DR. Heat shock proteins as mediators of aggregation-induced “danger” signals: Implications of the slow evolutionary fine-tuning of sequence for the antigenicity of cancer cells. Cell Stress and Chaperones. 1999;4:205–210. [PMC free article: PMC312935] [PubMed: 10590834]
- 178.
- Seya T, Akazawa T, Uehori J. et al. Role of Toll-like receptors and their adaptors in adjuvant immunotherapy for cancer. Anticancer Research. 2003;23:4369–4376. [PubMed: 14666723]
- 179.
- Wojtowicz-Praga S. Reversal of tumour -induced immunosuppression: A new approach to cancer therapy. J Immunother. 1997;20:165–177. [PubMed: 9181454]
- 180.
- Matzinger P. The danger model: A renewed sense of self. Science. 2002;296:301–305. [PubMed: 11951032]
- 181.
- Matzinger P. Essay 1: The danger model historical context. Scand J Immunol. 2001;54:4–9. [PubMed: 11439142]
- 182.
- Multhoff G, Boltzer C, Jennen L. et al. A stress inducible 72KDa heat shock protein (HSP72) is expressed on the surface of human tumor cells, but not on normal cells. Int J Cancer. 1995;61:272–279. [PubMed: 7705958]
- 183.
- Multhoff G. Activation of natural killer cells by heat shock protein 70. Int J Hyperthermia. 2002;18:576–583. [PubMed: 12537756]
- 184.
- de VriesLJM, Lesterhuis J, Scharenborg Nm. et al. Maturation of dendritic cells is a prerequisite for inducing immune response in advanced melanoma patients. Clinical Cancer Research. 2003;9:5091–5100. [PubMed: 14613986]
- 185.
- Ostberg JR, Patel R, Repasky EA. Regulation of immune activity by mild (fever-range) whole body hyperthermia: Effects on epidermal Langherans cells. Cell Stress and Chaperones. 2000;5:458–461. [PMC free article: PMC312877] [PubMed: 11189452]
- 186.
- Ostberg JR, Kabingu E, Repasky EA. Thermal regulation of dendritic cell activation and migration from skin explants. Int J Hyperthermia. 2003;19:520–533. [PubMed: 12944167]
- 187.
- Todryk SM, Gough MJ, Pockeley AG. Facets of heat shock protein 70 show immunotherapeutic potential. Immunology. 2003;110:1–9. [PMC free article: PMC1783014] [PubMed: 12941135]
- 188.
- Todryk SM, Melcher AA, Dalgleish AG. et al. Heat shock protein refine the danger theory. Immunology. 2000;99:334–337. [PMC free article: PMC2327171] [PubMed: 10712661]
- 189.
- Voll RE, Hermann M, Roth EA. et al. Immunosuppressive effects of apoptotic cells. Nature. 1997;350 [PubMed: 9389474]
- 190.
- Valubas RM, Braedel S, Hilf N. et al. The endoplasmatic reticulum-resident Heat Shock Protein Gp96 activates dendritic cells via the Toll Like Receptor 2/4 pathway. J Biol Chem. 2002;277:20847–20853. [PubMed: 11912201]
- 191.
- Medzhitov R. Toll-like receptors and Innate immunity. Nature Reviews. 2001;1:135–145. [PubMed: 11905821]
- 192.
- Beg AA. Endogenous ligands of Toll-like receptors: Implications for regulating inflammatory and immune responses. Trends in Immunology. 2002;23:509–512. [PubMed: 12401394]
- 193.
- Liwu L. Regulation of innate immunity signalling and its connection with human diseases. Current Drug Targets-Inflammation and Allergy. 2004;3:81–86. [PubMed: 15032644]
- 194.
- Tournier JN, Hellman AQ, Lesca G. et al. Fever-like thermal conditions regulate the activation of maturing dendritic cells. J Leukoc Biol. 2003;73:493–501. [PubMed: 12660224]
- 195.
- Basu S, Srivastava PK. Fever-like temperature induces maturation of dendritic cells through induction of hsp 90. Int Immunol. 2003;15:1053–1061. [PubMed: 12917257]
- 196.
- Okamoto M, Tazawa K, Kawagoshi M. et al. The combined effect against colon-26 cells of heat treatment and immunization with heat treated colon-26 tumor cell extract. Int J Hyperthermia. 2000;16:263–273. [PubMed: 10830588]
- 197.
- Schueller G, Paolini P, Friedl J. et al. Heat treatment of hepatocellular carcinoma cells: Increased levels of heat shock proteins 70 and 90 correlate with cellular necrosis. Anticancer Research. 2001;21:295–300. [PubMed: 11299751]
- 198.
- Di CarloE, Forni G, Lollini PL. et al. The intriguing role of polymorphonuclear neutrophils in antitumor reactions. Blood. 2001;97:339–345. [PubMed: 11154206]
- 199.
- Gough MJ, Melcher AA, Ahmed A. et al. Macrophages orchestrate the inmmune response to tumor cell death. Cancer Res. 2001;61:7240–7247. [PubMed: 11585761]
- 200.
- Urano M, Taradi M, Taradi SK. Enhancement of the thermal response of murine tumour and normal tissues by a streptococcal preparation, OK-432 (Picibanil). Int J Hyperthermia. 1991;7:113–23. [PubMed: 2051067]
- 201.
- Urano M, Yamashita T, Suit HD. et al. Enhancement of thermal response of normal and malignant tissues by Corynebacterium parvum. Cancer Research. 1984;44:2341–2347. [PubMed: 6722772]
- 202.
- Taradi M, Urano M, Taradi SK. et al. Augmentation of mouse natural killer cell activity by combined hyperthermia and streptococcal preparation, OK-432 (Picibanil) treatment. Int J Hyperthermia. 1991;7:653–65. [PubMed: 1919160]
- 203.
- Urano M, Overgaard M, Suit H. et al. Enhancement by Corynebacterium parvum of the normal and tumor tissue response to hyperthermia. Cancer Res. 1978;38:862–4. [PubMed: 626985]
- 204.
- Shah SA. Enhanced thermal response of a rat sarcoma by Corynebacterium parvum. Cancer Lett. 1985;26:235–40. [PubMed: 3978610]
- 205.
- Gridley DS, Nutter RL, Kettering JD. et al. Mouse neoplasia and immunity: Effects of radiation, hyperthermia, 2-deoxy-D-glucose, and Corynebacterium parvum. Oncology. 1985;42:391–8. [PubMed: 4069554]
- 206.
- Okamoto M, Oshikawa T, Tano T. et al. Involvement of toll-like receptor 4 signalling in interferon- γ production and antitumor effect by Streptococcal agent OK-432. J Natl Cancer Inst. 2003;19:316–326. [PubMed: 12591988]
- 207.
- Fidler IJ. The organ microenvironment and cancer metastasis. Differentiation. 2002;70:498–505. [PubMed: 12492492]
- 208.
- Heppner GH, Miller BE. Therapeutic implications of tumor heterogeneity. Seminars in Oncology. 1989;2:91–105. [PubMed: 2652316]
- 209.
- Shah S. Metastasis and hyperthermiaIn: Anghileri L, Robert J, eds.“Hyperthermia in Cancer Treatment”CRC Press,1986191–227.
- 210.
- Dickson JA, Ellis HA. Stimulation of tumor cell dissemination by raised temperature (42°C) in rats with transplanted Yoshida tumours. Nature. 1974;248:354. [PubMed: 4819644]
- 211.
- Shen R, Hornback NB, Shidnia H. et al. Whole body hyperthermia decreases lung metastases in lung tumor-bearing mice, possibly via a mechanism involving natural killer cells. J Clin Immunol. 1987;7:246–253. [PubMed: 3597715]
- 212.
- Urano M, Rice L, Epstein R. et al. Effect of whole -body hyperthermia on cell survival, metastases frequency, and host immunity in moderately and weakly immunogenic murine tumors. Cancer Res. 1983;43:1039–43. [PubMed: 6825078]
- 213.
- Oda M, Koga S, Maeta M. Effects of total-body hyperthermia on metastases from experimental mouse tumors. Cancer Res. 1985;45:1532–1535. [PubMed: 3919941]
- 214.
- Dewhirst MW, Sim Da, Forsyt K. et al. Local control and distant metastases in primary canine malignant melanomas treated with hyperthermia /or radiotherapy. Int J Hyperthermia. 1985;1:219–234. [PubMed: 3836269]
- 215.
- Prostnitz LR, Maguire P, Anderson JM. et al. The treatment of high grade soft tissue sarcomas with preoperative thermoradiotherapy. Int J Radiat Oncol Biol Phys. 1999;45:941–949. [PubMed: 10571201]
- 216.
- Hegewisch-Beecker S, Braun K, Otte M. et al. Effects of whole body hyperthermia (41.8°C) on the frequency of tumor cells in the peripheral blood of patients with advanced malignancies. Clinical Cancer Res. 2003;9:2079–2084. [PubMed: 12796371]
- 217.
- Pontiggia P, Mc LarenJR, Baronzio GF. et al. The biological response to heat. “Consensus On Hyperthermia for 1990s” Advances in Experimental Medecine and Biology. 1990;267:271–291. [PubMed: 2088044]
- 218.
- Sitnicka EW, Olszewski WL, Lukomska B. Influence of whole body hyperthermia on natural cytotoxicity of liver blood- borne sinusoidal cells. Int J Hyperthermia. 1993;9:731–743. [PubMed: 7504037]
- 219.
- Yang M, Lauzon W, Lemaire I. Effects of hyperthermia on natural killer cells: Inhibition of lytic function and microtubule organization. Int J Hyperthermia. 1992;8:87–97. [PubMed: 1545166]
- 220.
- Zanker KS, Lange J. Whole body hyperthermia and natural killer activity. Lancet. 1982;I:1079–1080. [PubMed: 6122886]
- 221.
- Kappel M, Tvede N, Hansen MB. et al. Cytokine production ex vivo: Effect of raised body temperature. Int J Hyperthermia. 1995;11:329–335. [PubMed: 7636320]
- 222.
- Quinn TD, Polk HC, Edwards MJ. Hyperthermic isolated limb perfusion increases circulating levels of inflammatory cytokines. Cancer Immunol Immunother. 1995;40:272–275. [PubMed: 7750126]
- 223.
- Ostberg JR, Kaplan KC, Repasky EA. Induction of stress proteins in a panel of mouse tissues by fever -range whole body hyperthermia. Int J Hyperthermia. 2002;18:552–562. [PubMed: 12537754]
- 224.
- Li Z, Menoret A, Srivastava P. Role of heat-shock proteins in antigen presentation and cross presentation. Current Opinion in Immunology. 2002;14:45–51. [PubMed: 11790532]
- 225.
- Kubista B, Trieb K, Blahovec H. et al. Hyperthermia increases the susceptibility of chondro-and osteosarcoma cells to natural killer cell-mediated lysis. Anticancer Res. 2002;22:789–792. [PubMed: 12014651]
- Introduction
- Tumor Immunity
- Therapeutic Modalities
- Effects of Thermal Component of Fever on Immune System
- Effects of Induced Thermal Elevation (Hyperthermia) on Immunity
- Specific Effects of Hyperthermia on Immune Therapeutic Modalities
- Effects of Hyperthermia on Lymphocyte Homing
- The Danger Model and Hyperthermia-Effects on Dendritic Cell Maturation and Stimulation of Innate-Adaptive Immunity
- Effects of Hyperthermia on Metastatic Process
- Conclusions and Comments
- References
- Effects of Local and Whole Body Hyperthermia on Immunity - Madame Curie Bioscien...Effects of Local and Whole Body Hyperthermia on Immunity - Madame Curie Bioscience Database
- ygbK putative 3-oxo-tetronate kinase YgbK [Escherichia coli str. K-12 substr. MG...ygbK putative 3-oxo-tetronate kinase YgbK [Escherichia coli str. K-12 substr. MG1655]Gene ID:947199Gene
- LOC339685 [Homo sapiens]LOC339685 [Homo sapiens]Gene ID:339685Gene
- Inpp4b inositol polyphosphate-4-phosphatase, type II [Mus musculus]Inpp4b inositol polyphosphate-4-phosphatase, type II [Mus musculus]Gene ID:234515Gene
- Mtx2 metaxin 2 [Mus musculus]Mtx2 metaxin 2 [Mus musculus]Gene ID:53375Gene
Your browsing activity is empty.
Activity recording is turned off.
See more...