NCBI Bookshelf. A service of the National Library of Medicine, National Institutes of Health.
Montmayeur JP, le Coutre J, editors. Fat Detection: Taste, Texture, and Post Ingestive Effects. Boca Raton (FL): CRC Press/Taylor & Francis; 2010.
11.1. INTRODUCTION
Fat plays a unique role in the human diet. In addition to being the most concentrated source of dietary energy, fat contributes to the texture, flavor, and aroma of a wide variety of foods. In general, the most palatable foods are those that are both energy-dense and high in fat content (Drewnowski, 1997a,b). The taste, smell, mouthfeel, and hedonic properties of fat all contribute to the popular concept of fat “taste” (Drewnowski, 1997a).
Fat is one reason why palatability and energy density of foods are closely intertwined (Drewnowski, 1997a,b). Energy density of foods is largely determined by their water and fat content (Drewnowski, 1998). By providing weight without energy, water has more impact on energy density of foods than does any macronutrient, even fat (Drewnowski, 1997a). Together, water and fat account for over 95% of the variance in the energy density of foods in the food supply.
Energy-dense foods and diets have been associated with higher energy intakes (Andrieu et al., 2006) and with higher prevalence of obesity and the metabolic syndrome (Mendoza et al., 2007). By all reports, the energy density of the Western diet is on the rise, as is the consumption of dietary fats (Drewnowski and Popkin, 1997; Popkin et al., 2001; Drewnowski, 2005). In the United Kingdom, dietary fat accounted for an average of 36% of dietary energy for men and 35% for women (Henderson et al., 2003). In France, dietary fat accounted for 37%–42% of dietary energy, with saturated fat providing 16% (Drewnowski et al., 1996; Perrin et al., 2002; Astorg et al., 2004). In the United States, dietary fat accounted for 33%–36% of dietary energy (Allred, 1995; CDC, 2004). Despite public health efforts to lower fat consumption, global consumption of both animal and vegetable fats continues to rise (CDC, 2004; Amuna and Zotor, 2008). Innate preferences for dietary fats seem to be tempered only by incomes.
As incomes rise, developing nations typically replace plant-based diets with more animal fats, vegetable oils, and caloric sweeteners, a phenomenon known as the “nutrition transition” (Drewnowski and Popkin, 1997). Paradoxically, developed nations recommend replacing fat-rich foods with water-laden grains, vegetables, and fruit in order to increase bulk and so reduce the energy density of the diet. Recommendations to reduce dietary energy density are one way to reduce energy intakes in an effort to address the global obesity epidemic (WHO Report, 2003).
The problem is that the palatability and enjoyment of foods are often tied to their energy density and therefore fat content. Energy-dense foods that are rich in fat are more palatable than are many low-energy-density vegetables and fruit (Drewnowski, 1998). High-fat foods, many containing sugar or salt, have an undeniable sensory appeal and are difficult to resist (Folkenberg and Martens, 2003). Energy-dense high-fat diets are consumed in preference to plant-based diets of grains, pulses, and legumes.
There are many explanations for why humans like fat (Drewnowski, 1997a,b). Several physiological mechanisms have been proposed, many of which are based on the strong links found between fat content, palatability, satiety, and energy density. The orosensory properties of fat or fat “taste” seem to be perceived through a combination of taste, texture, and olfaction (Drewnowski, 1997a; Schiffman et al., 1998). Fat is a concentrated source of energy with rewarding postingestive effects (Drewnowski, 1995). The learning of food preferences may be based on associating sensory attributes with the physiologic consequences of ingestion, such as satiety and well-being (Birch, 1999).
Parallels have been drawn between sugar and fat preferences. Sensory preference for sweet taste is present at birth, and the “sweet tooth” of early childhood helps to introduce new foods into the children’s diet (Birch, 1999). Children learn quickly to prefer flavors associated with high-energy content and begin to select high-fat foods early in life (Johnson et al., 1991; Birch, 1992). Genetic predisposition, metabolic needs, and behavioral or emotional factors can influence the liking for fats. Human preferences for fat-rich foods may also be influenced by economic factors and sociocultural values (Drewnowski, 1995; Drewnowski, 1997a; Tuorila and Pangborn, 1988).
This chapter will cover recent advances in the understanding of the genetic, physiological and behavioral factors related to the perception of and preference for fat in humans. As will be discussed further, human liking for fats may be a consequence of evolutionary pressures to select energy-dense foods to assure nutrition and survival (Drewnowski and Rock, 1995).
11.2. PERCEPTION OF FAT-RICH FOODS: TASTE, OLFACTION, AND TEXTURE
Sensory perception of foods involves the initial stage of chemosensation, which includes the detection of tastants, odorants, and textural attributes of foods, followed by the integration of sensory signals by the brain. Sensory processes begin with the placement of food in the mouth, the fracturing of the food by the teeth and its dilution with saliva, oral perception of temperature and texture, and the binding of taste and flavor molecules to receptors in the oral and nasal cavities. Activated receptors act on secondary messengers or other systems, sending a signal through the sensory nerves to the brain. Upon integration of sensory input, we become aware of the taste, aroma, and texture of foods (Chandrashekar et al., 2006; Engelen and Van der Bilt, 2008). The hedonic component of sensation, known as palatability or the “pleasantness” of food is an integral part of a complete sensory profile for a given food (Yeomans, 1998).
Fats are perceived by a variety of sensory mechanisms (Drewnowski 1989a; Mattes, 2005). The concept of food “taste” as understood by consumers includes the chemical sense of taste (gustation); smell/aroma (olfaction), and the perception of food texture in the mouth. The first sensory response tends to be the olfactory perception through the nose or mouth of fat-soluble volatile flavor molecules (Drewnowski et al., 1989; Drewnowski, 1990a). Texture, defined as the overall measure of oral sensations associated with placing food in the mouth (Drewnowski, 1997a), adds to the sensation of fatty taste (Drewnowski et al., 1989). The physical form that fat takes at different temperatures can also influence mouthfeel and contribute to the enjoyment of high-fat foods.
Traditionally, studies in sensory psychophysics have examined human taste acuity (detection and recognition thresholds) or taste sensitivity (intensity scaling), using aqueous solutions of sweet, salty, sour, or bitter compounds. However, such techniques have not been applied to the study of fats in foods. One reason is that the chemical structure of triglycerides makes them insoluble in saliva such that the delivery of fat molecules to receptors in aqueous solutions is not possible. Therefore, many early studies have focused on the texture of fat-containing foods in relation to food quality and food preference.
11.2.1. Perception and Preferences for Fat Taste
The first sensation of fat flavor is olfactory perception of volatile, fat-soluble molecules through the nose, also known as orthonasal olfaction. Olfactory perception of already-ingested foods that occurs in the oral cavity is referred to as retronasal olfaction and may employ distinct brain pathways (Heilmann and Hummel, 2004; Mattes, 2005). Food texture and mouthfeel, which may change as a result of chewing and mastication are also detected in the oral cavity (Drewnowski, 1987, 1991a, 1995).
Recent evidence indicates that the sensation of fat can be mediated through chemoreception and not just through mechanical perception of texture. Evidence that free fatty acids are perceived through chemoreception comes largely from animal studies. Rats may be able to detect free fatty acids in the oral cavity through fatty acid-sensitive receptors (Gilbertson et al., 1997, 2005) in a sex-dependent manner (Pittman et al., 2008). A specific fatty acid transporter (CD36) for linoleic acid has been described in mice (Laugerette et al., 2005) and a potential pathway for the detection of a “fatty taste” by the mouse has been described as well (Gaillard et al., 2008).
A variety of fat-digesting lipases may assist in the chemosensory process. Human lipases digest dietary fat (mostly triacylglycerides) into glycerol and fatty acids. Lipases are present in the digestive juices (lingual lipase, gastric lipase, and pancreatic lipase); inside cells (hormone-sensitive lipase and lysosomal acid lipase); and in endothelial cells (lipoprotein lipase and hepatic lipase) (Hamosh, 1990). In newborns, lingual lipase breaks down the fat globules in milk, releasing free fatty acids to begin the digestive process. In adults, the role of lingual lipase is less clear (Schiffman et al., 1998), since enzyme levels in adult humans are apparently too low to digest dietary triglycerides (Spielman et al., 1993). In any case, sensory studies have long demonstrated that free fatty acids are perceived as acrid, pungent, nauseous, and generally repulsive (Schiffman and Dackis., 1975). Free fatty acids are usually removed from processed food for health, safety, and stability reasons. Free fatty acids such as linoleic acid and other unpleasant fatty acids are involved in product deterioration (Hansen and Rose, 1996; Refsgaard et al., 2000) and are generally bitter.
Indeed, the ability to perceive free fatty acids ought to lead to product rejection rather than acceptance. For example, there is 0.6–1.5 mg of free fatty acid in 1 g of milk. Fatty acid levels above 3.5 mg/g or 5.6 mg/g in fresh milk were in fact rejected by a sensory panel (Nasser et al., 2001). A recent study (Chalé-Rush et al., 2007) evaluated the sensitivity to linoleic, oleic, and stearic acids of 22 healthy adults, using taste, orthonasal, and retronasal olfaction. Oral and nasal irritancy were also measured. Orthonasal perception had markedly lower thresholds, suggesting that being able to detect free fatty acids prior to ingestion (Heilmann and Hummel, 2004) may have had evolutionary importance in the detection of spoilt food by primates.
Human ability to detect bitter compounds may act as a warning signal, leading to the rejection of toxic plant compounds (Drewnowski, 2000), or spoiled food containing oxidized fat (Mattes, 2005). The detection systems for free fatty acids may reflect evolutionary adaptation against the ingestion of undesirable or toxic compounds. Even if humans do have one or more sensory mechanisms for detecting free fatty acids, such mechanisms appear more tied to avoidance and survival than to sensory pleasure and enjoyment.
The olfactory perception of fat-soluble flavor molecules may be more closely linked to hedonic value. Food odors may increase appetite and induce salivation and promote the release of gastric acid and insulin (Yeomans, 2006). Warwick et al. (1993) showed that combining smell and taste sensations (using vanilla and aspartame) enhanced subjects’ satiety after a high-fat meal, compared with a bland version of the same meal. The neuroanatomical overlap of brain structures processing taste, satiety, and emotion could explain why some fat-soluble volatile compounds can produce feelings of pleasure or disgust, without affecting our ability to detect fat content (Schiffman et al., 1998). Recent neuroimaging studies identified brain loci for sensory information on the mouthfeel, viscosity, odor, and even the pleasantness of fat. These regions are also accessed by satiety and physiological signals (De Araujo and Rolls, 2004; Rolls, 2004).
Both humans and rats are capable of detecting low concentrations of triglycerides in emulsions. Rats were able to discriminate a 0.78% corn oil emulsion from water (Mindell et al., 1990). Among humans, detection thresholds ranged from 5.3% (vol/vol) in young adults to 15.8% (vol/vol) in elderly subjects (Schiffman et al., 1998), depending on the type of emulsifier. Emulsifiers, naturally present in food, may modulate the interaction of the fat globules with the taste receptors (Schiffman et al., 1998). Young subjects (mean age 23 years) were able to discriminate the fat content of whole milk versus skim milk with difference thresholds ranging from 1.4% to 2.1%. These thresholds were not lowered by odor cues (Mela, 1988; Schiffman et al., 1998). Humans were better at detecting the fat content of liquids compared to solid foods (Mela and Christensen, 1987).
Recent studies suggest that oral exposure to fat per se, before ingestion, may influence metabolic processes related to digestion and energy storage, and may have implications for long-term health status (Mattes, 2002, 2005). Olfactory detection of pleasant, fatty flavors may also be linked with induction of preparatory digestive mechanisms, or satisfaction and satiety after consumption of a meal. Sensory perception of fats, whether mediated by taste, texture, olfaction, or by some combination of all three, seems to have important consequences for fat metabolism (Mattes, 2005).
11.2.2. Perception and Preference for Fat Texture
Fats endow foods with some key textural properties, including viscosity (thickness) and lubricity (slipperiness or oiliness) (Drewnowski and Greenwood, 1983; Mela, 1988; Schiffman et al., 1998). Food texture studies focused on the viscosity, elasticity, and orientation and elongation of food particles, often in relation to food acceptance. Texture studies showed, for example, that consumer preferences for yogurts and other dairy products were driven by perception of smooth or creamy textures that depended on fat content (Folkenberg and Martens, 2003).
The classic definition of food texture (Szczesniak, 1963) held that the perception of product texture arises from the dynamic interaction between the food and the consumer, occurring along the dimension of time. Multiple senses were involved. Whereas oral sensation or mouthfeel refer to the sense of touch, the perception of texture could also involve both sight and hearing, and the manner in which the food responds to the applied forces (Engelen and Van der Bilt, 2008). Another definition held texture to be a dynamic interaction with time between the food’s physical properties and the senses of touch, sight, and hearing (Engelen and Van der Bilt, 2008).
Mastication and swallowing played an essential role in the dynamic perception of texture in semisolid foods (Engelen and Van der Bilt, 2008). Semisolid is a texture represented by such foods as sauces, mayonnaise, cream, spread cheeses, ice creams, and margarines. The presence of particles, the size of particles, and their orientation are important, but only when combined with physiological processes taking place in the oral cavity, such as salivation (saliva flow, rate, and composition), tongue movements, and temperature exchanges between food and oral cavity (Engelen and Van der Bilt, 2008).
Because fats endow foods with a wide range of taste and texture properties, it is difficult to distinguish which particular oral sensations contribute to perception of fat content. Sensory evaluation of fats in solid foods is further complicated by the fact that the fat may be an integral part of the food (such as marbling in meat) or fats may be added during preparation. Fat globules in dairy products cannot be individually perceived in the oral cavity, but the substance may feel creamy (Cooper, 1987). Fat in frozen dairy products contributes to creaminess and smoothness by preventing the formation of large ice crystals. The principal sensory cue for fat content in milk or cream is stimulus thickness, smoothness, or viscosity (Drewnowski, 1987). The fat content of dairy products (Mela, 1988), peanut butter, margarine, and mayonnaise were also associated with thickness, smoothness, and viscosity (Kokini et al., 1977; Cussler et al., 1979). Temperature of the food, or temperature of the oral cavity does not seem to influence perception of fat content of oil-and-water emulsions (Mela et al., 1994a); but particle size (degree of emulsification) does, with smaller particle size slightly enhancing perception of fat content (Mela et al., 1994b).
Some of the terms used to characterize the perception of texture in the oral cavity, and particularly fat texture, and their definitions are summarized in Table 11.1. Table 11.2 shows how descriptive terms, classified into different categories may apply to characterizing the mouthfeel of beverages.
TABLE 11.1
Terms Used to Characterize the Oral Sensation of Dietary Fats
TABLE 11.2
Terms Used to Characterize the Mouthfeel of Beverages
The general foods (GF) Texture Profile was created to standardize evaluation criteria for product texture (Brandt et al., 1963; Szczesniak et al., 1963). This model evaluated sensory properties of foods over time, from placement in the mouth to complete mastication. It identified primary mechanical characteristics of food such as hardness, cohesiveness, adhesiveness, and viscosity; and secondary characteristics such as brittleness, chewiness, and gumminess. Further, geometrical characteristics were defined based on the orientation, shape and size of the food particles (gritty, grainy, and coarse), and mouthfeel characteristics were defined based on perception of moisture or fat (wet, oily, and greasy). The use of the texture profile entailed the introduction of a number of anchored rating scales (Szczesniak et al., 1963), such as for hardness, fracturability, chewiness, gumminess, adhesiveness, and viscosity. Each scale represented a wide range of textures, so that many different foods could be evaluated (reviewed in Drewnowski, 1991a). It is important to note that some of these descriptors are not hedonically neutral, i.e., while “creaminess” may be a desirable property, “greasiness” generally is not. Table 11.3 summarizes the GF Texture Profile scales for two attributes, viscosity, and adhesiveness, together with product anchors.
TABLE 11.3
Descriptors for Oral Sensations Associated with Fat and the Viscosity Scale from the General Foods Texture Profile
Different attribute scales reflect different aspects of fat texture perception. In one study, 25 normal weight men and women rated seven liquid dairy products along multiple 9-point attribute scales. Attribute scales included the standard texture and mouthfeel terms above as well as more abstract evaluations of the energy density of the sample (rich, fattening). There was a log-linear relation between attribute ratings and stimulus fat content, best seen when the graphs were plotted on a logarithmic scale. These data are summarized in Figure 11.1.
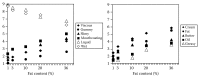
FIGURE 11.1
Relation between texture attributes and fat content of fluid dairy products. Sensory evaluation was carried out using 9-point category scales for each attribute, where 1, “not at all –” and 9, “extremely –”. Subjects (more...)
Fat can also affect the texture of cooked or processed food. Frying in fat brings the food to temperatures above the boiling point of water and contributes to crispiness or crunchiness in cakes, pastries, and cookies. Fat contributes to freshness and moisture by binding water molecules (Cooper, 1987). Appropriate terms for describing those texture attributes were also a part of the GF Texture Profile.
The reliance on texture cues means that an illusion of fat content can be created by making the stimulus viscous, either by gelling or by the addition of hydrocolloid thickeners. This becomes far more complex when the stimulus is solid as opposed to liquid. Drewnowski and Schwartz (1990) asked 50 young women to rate the sweetness and fat content of 15 stimuli resembling cake frostings. The samples were composed of sucrose (20%–77%, wt/wt), polydextrose (a bland, partly metabolizable starch), unsalted butter (15%–35%, wt/wt), and water.
Figure 11.2 (left panel) shows that the perception of sweetness was largely unaffected by fat content. Sweetness ratings accurately tracked increasing sweetness concentration. By contrast, the perception of fat content plunged as the sugar content increased (right panel). Perceived fatness was a combined function of fat and sugar contents that was heavily dependent on product texture. The addition of water caused a sharp decrease in fatness ratings, with the sweetest stimuli, being rated as lowest in fat content. The presence of sugar clearly distorted the ability to perceive the fat content of solid foods (reviewed in Drewnowski, 1991a).
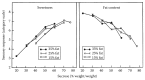
FIGURE 11.2
Ratings of perceived sweetness intensity (left panel) and fat content (right panel) as a function of stimulus sucrose and fat levels. (From Drewnowski, A. and Schwartz, M., Appetite 14, 203, 1990. With permission.)
However, preferences for fat may be independent of the conscious ability to detect or assess the fat content of solid foods. Another study compared the ability of 25 young men and women to rate the sweetness, creaminess, and fat content of solid and liquid foods of differing sugar and fat content (Drewnowski et al., 1989). Liquid dairy products included sweetened skim milk (<0.5% fat), whole milk (around 3.5% milk fat), half-and-half (12.5% milk fat), and heavy cream (36% milk fat). Solid foods consisted of cottage cheese, cream cheese, or the two blended together to produce mixtures of comparable fat content (0.1%–52%). The sweetened blends were spread over white bread and cut into small pieces to produce the solid food samples (Drewnowski et al., 1989). Subjects reliably estimated sweetness intensity for both solid and liquid samples, but they were unable to track the increasing fat content of the solid foods. Preferences were estimated using the 9-point hedonic preference scale (Peryam and Pilgim, 1957). Hedonic preferences for high-fat samples remained high despite difficulties in estimating stimulus fat content.
Despite impaired ability to track the fat content of solid foods, as opposed to liquids, subjects generally preferred stimuli with the higher fat content (Drewnowski, 1989a). Sensory assessment of fat content in foods can be a challenge, since it relies so much on the integration of multiple interrelated sensorial signals. Further, such assessment is system-specific, and may be affected by other product attributes, such as sugar content. Finally, given that the perception of food texture is driven by the interaction between the food and the consumer, it is not surprising that humans sometimes lack an accurate, conscious judgment of how much fat is contained in the food (Blundell and McDiarmid, 1997; Schiffman et al., 1998).
11.3. INTERACTIONS BETWEEN FAT, SUGAR, AND SALT
Fat content contributes to the food’s acceptability, palatability, and enjoyment. The creation of fat replacement products with a desirable and well-rounded sensory profile is a particular challenge for food chemists (Drewnowski, 1990b). Depending on the product, desired sensations may range from creaminess and smoothness (in dairy products), to tenderness and moisture (in cakes), to crispiness and crunchiness (in baked or fried foods). A particular hedonic synergy is obtained by pairing sugar and fat (Drewnowski and Greenwood, 1983; Drewnowski, 1991a, 1995; Schiffman et al., 1998).
When it comes to sweet liquids or solids, the hedonic response typically follows an inverted U-curve as preferences increase with added sweetness and then decline when the product is perceived as too sweet. The sensory optimum, usually reached at 8%–10% of sugar in aqueous solutions, is generally referred to as the “hedonic breakpoint.” Some individual variation is observed as hedonic responses to sugar may rise and then decline (type 1 response) or rise and plateau (type 2 response). In general, children prefer much sweeter solutions than do adults and show little evidence of a hedonic breakpoint. By contrast, hedonic response curves for older adults may actually decrease as a function of sugar concentration (Drewnowski, 1991a). The hedonic breakpoint for solids is found at a much higher sugar concentration than for liquids.
Sensory evaluations of mixtures of sweetened dairy products of variable sugar and fat concentration showed no evidence for a hedonic breakpoint for fat. In these studies, normal weight men and women were asked to taste and rate 20 mixtures of milk cream and sugar. Sugar content varied from 0% to 20%, whereas fat content varied from 0.1% to 36% (Drewnowski and Greenwood, 1983). Subjects were asked to rate sweetness, creaminess, perceived fat content, and preference (hedonic response) on a 9-point category scale. Later studies, based on identical design were conducted with massively obese patients, patients who had recently lost weight and normal weight controls (Drewnowski et al., 1985).
The sensory system was highly interactive when it came to hedonic preferences. Whereas hedonic ratings for sweetness peaked at 8%–10% sugar, depending on fat concentration, no sensory breakpoint for dairy fat was observed. There was also a synergy between sugar and fat: highest preference ratings were obtained for light cream with sugar (Drewnowski and Greenwood, 1983). Hedonic preference ratings for obese patients, as compared to normal weight controls, are shown in Figure 11.3. The graphs illustrate that obese patients disliked high concentrations of sucrose in skim milk, but showed increased acceptance when the sucrose was presented in heavy cream. For both normal weight and for obese subjects, hedonic preferences for taste stimuli were a joint function of sugar and fat content.
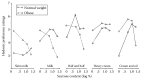
FIGURE 11.3
Hedonic preference ratings for dairy products sweetened with different amounts of sucrose. Ratings are for obese patients and normal weight controls. (Data from Drewnowski, A. et al., Am. J. Clin. Nutr. 61, 1206, 1995.)
Modeling hedonic responses to a two-dimensional sensory system required the use of a mathematical modeling technique, the response surface method (RSM) (Drewnowski and Greenwood, 1983). This analytical procedure can be used to predict and plot the response to multiple stimuli for any combination of ingredient levels. Used in food optimization studies, RSM can determine the combination of ingredients that yield the most preferred product and can graph hedonic optima in three dimensions (Drewnowski and Greenwood, 1983; Drewnowski et al., 1985, 1987b). A contour map, sometimes called “isobologram,” joins points representing the same level of response to variable stimulus levels of sugar and fat (Gessner, 1995). Drewnowski and Greenwood (1983) showed that the peak hedonic response was obtained at high, but not highest, fat and sugar content (8% sugar and 20% fat). There was a strong interaction between the sweet and the fat component. Whereas sweetened skim milk and unsweetened heavy cream were not highly preferred, highest hedonic ratings were obtained for sweetened light cream (reviewed in Drewnowski, 1995).
Figure 11.4 (top panels) shows the three-dimensional representation of the hedonic response surface for obese patients, reduced obese patients, and normal weight controls. Isopreference contours are shown directly below (bottom panels). The data show that reduced obese patients had the highest hedonic ratings, selecting high energy density stimuli that were highest in sugar and fat content (Drewnowski et al., 1985).

FIGURE 11.4
Hedonic preference ratings for sweetened dairy products of differing sugar and fat content. The data are for normal weight subjects, obese patients, and reduced obese patients. Data are presented as three-dimensional hedonic response surfaces (top panels) (more...)
Sensory responses to sugar/fat mixtures were further explored as a function of extremely low body weight (Drewnowski et al. 1987a,b). Those clinical studies investigated female patients with anorexia nervosa and bulimia nervosa, as compared to normal weight age-matched female controls. A negative relation (r = −0.43) between sugar/fat ratios and degree of overweight confirmed clinical reports of differential response to dietary fat by anorectic and obese women. Whereas anorectic patients tended to reject fat-rich foods, obese patients, on the contrary showed enhanced fat preferences. An association between high preference for fats and overweight had also been shown in other studies (Mela and Sacchetti, 1991; Drewnowski and Holden-Wiltse, 1992). Formerly obese patients maintaining weight loss showed enhanced sensory preferences for both sugar and fat (reviewed in Drewnowski, 1991a).
A majority of laboratory studies have supported the link between obesity and liking for higher fat foods (Drewnowski and Greenwood, 1983; Drewnowski et al., 1985; Mela and Rogers, 1998; Rissanen et al., 2002; De Graaf, 2005). However, the findings were not universal (Salbe et al., 2004). Obese persons tend to consume more energy-dense diets and formerly obese subjects show enhanced responsiveness to fat (Drewnowski et al., 1985; Cox et al., 1999; Rissanen et al., 2002). However, some cross-sectional and longitudinal studies failed to show a convincing link between BMI values and self-reported liking for high-fat foods.
Sensory studies on fat and salt mixtures are extremely limited. Warwick and Schiffman (1990) studied sensory responses for fat/sugar and fat/salt mixtures in young and elderly subjects of different body weights. The stimuli contained 0.5%–36% fat by weight, and 0%–20% sucrose, or 0%–0.6% NaCl. Younger subjects preferred sweeter stimuli, consistent with previous studies (Drewnowski, 1991a; Cox et al., 1999). Hedonic ratings of elderly subjects were not influenced by fat content. Younger subjects showed a link between preferences for fat/salt mixtures but the elderly did not.
11.4. MECHANISMS UNDERLYING PREFERENCES FOR FAT: PALATABILITY OR SATIETY?
There is no question that dietary sugars and fats are associated with overconsumption of energy. Whether this is due to excess palatability or deficient satiety is an unresolved issue. On one hand, fat and sugar mixtures are among the most preferred of foods (Drewnowski and Greenwood, 1983; Drewnowski, 1991a). The foods most preferred by young children are energy-dense, familiar, and sweet. Typically, such foods include chocolate, cookies, jellies, and candy (Drewnowski, 1989a). High-fat foods were not only the most preferred, but induced the least neophobia (Rankin and Mattes, 1996). Children quickly learned to select flavors associated with high-energy content, that is, foods rich in fat (Drewnowski, 1989a; Johnson et al., 1991; Birch, 1992).
Highly preferred foods can stimulate consumption independent of energy deficit or perceived hunger (Sørensen et al., 2003). Palatable foods can stimulate appetite and eating rate, leading to excessive food consumption (Yeomans et al., 1997, 2001, 2004). This overriding of hedonic responses over metabolic responses has been termed passive overconsumption (Blundell and Mcdiarmid, 1997), or more recently, nonhomeostatic eating (Mela, 2006).
Research has begun to integrate the homeostatic (energy) and the hedonic (pleasure) components of appetite control, assigning a role to weight status. Mela (2006) has noted that obesity seems to be linked to an increased “wanting” of highly palatable foods (motivation for eating) that is not necessarily linked to higher “liking” of those foods. Studies by Blundell et al. support the notion that wanting (how hard you are prepared to work for a food) and liking (the actual pleasure effects of eating the food) are two independent components of appetite, which can be measured separately (Blundell and Finlayson, 2004; Finlayson et al., 2007).
Studies on endogenous opioid peptides seem to support the hedonic or “liking” viewpoint (Reid, 1985; Cooper et al., 1988; Drewnowski et al., 1992a, 1995; Will et al., 2004; Ward and Dykstra, 2005). Endogenous opioid peptides are pleasure-enhancing molecules produced in the human brain. Early studies in rodents identified the link between endogenous opioid peptides and sugar and fat intake (Blass, 1987). Later clinical studies (Drewnowski et al., 1992a, 1995) examined sensory preferences for fat and sugar following administration of opioid peptide agonists (butorphanol) and antagonists (naloxone).
Those studies were conducted in normal weight and obese bulimic patients and nonbinging controls. Bulimic women consumed more sweet, high-fat foods under laboratory conditions than did nonbinging controls. Following naloxone administration, taste preferences for sugar/fat mixtures were suppressed by the opiate blockade. Food consumption of sweet high-fat foods (mostly chocolate) was also selectively suppressed, but only in bulimic females. The conclusion was that the opoid antagonist naloxone selectively affected the hedonic component of overeating: Stronger suppression was obtained for sweet- and fat-containing foods than for bland breadsticks and popcorn. Since sensory preferences were affected as well, the conclusion was that naloxone exerted its effects by reducing the hedonic appeal of palatable foods. By contrast, no differences in sensory or consumption responses were obtained between obese women and women of normal body weight. In retrospect, body weight was unrelated to the wanting of palatable foods, at least, under those particular laboratory conditions.
Butorphanol, an opiate agonist, administered in very small doses, had no impact on taste preferences or food selection. These clinical studies showed that sensory preferences for sweet and high-fat foods did involve opioid peptides that are implicated in food reward. Naloxone had less effect on foods that were high in either sugar or fat than on foods that contained both. The sweet high-fat foods, mostly chocolate, were the most preferred, most palatable combinations. In other studies also, naltrexone, another opioid antagonist, only had an effect on the consumption of most preferred foods (Yeomans and Gray, 1996).
Considering the growing body of research linking food reward with the endogenous opioid system in humans (Reid, 1985; Drewnowski et al., 1985, 1992a; De Araujo and Rolls, 2004; Davies et al., 2007) and animals (Will et al., 2004; Mizushige et al., 2006, 2007, Sclafani, 2007), it seems safe to conclude that both oral (including hedonics) and postingestive (including neuropeptides) stimuli are likely to play an interactive role and that hedonic factors are likely to override homeostatic controls on food intake at least under particular psychological conditions. In particular, dopamine, a neurotransmitter involved in the reward response, has been implicated in fat overconsumption and positive energy balance in humans (Davis et al., 2007). Low levels of brain dopamine have been shown to predict overeating and obesity in women (Goldfield et al., 2007). Dopamine has also been implicated in the concept of food addictions, generally involving sweet and high-fat foods (Drewnowski and Bellisle, 2007).
Additional information has come from recent neuroimaging studies. The anorectic peptide YY (PYY), associated with satiety, may downregulate preferences for high-fat foods in laboratory animals (Chandarama and Batterham, 2008). Functional brain imaging studies indicate that an exogenous analog to PYY is able to activate the orbitofrontal cortex; reduce motivation for seeking high-fat foods, and induce weight loss. These findings highlight the tightly interconnected roles for the hedonic and homeostatic pathways. In fact, recent studies in animals have shown that neurons in the homeostatic (“metabolic”) and reward (“cognitive”) brain areas are in close anatomic contact (Zheng et al., 2007).
Satiety is another mechanism advanced to explain human overconsumption of fats. Fats are also said to be less satiating than either carbohydrate or protein (Blundell et al., 1993; Rolls, 1995). The last point is something of a puzzle, since fats contain more energy per gram than does protein; slow down gastric emptying, and very effectively delay the onset of the next meal. Furthermore, palatability and satiety are generally measured in terms of food consumption. Higher intakes mean that a food is palatable; lower intakes mean that it is satiating. By definition then, palatability is the inverse of satiety, such that having a satiating yet palatable food is a contradiction in terms.
Distinguishing between satiety and satiation resolved the paradox. Whereas satiation refers to internal sensations responsible for terminating the current meal, satiety refers to processes that influence the time delay until the following meal and subsequent meal size. Fats were decreed to have an impact on satiety but not on satiation, neatly sidestepping the paradox. Of course, this makes it impossible to distinguish between high palatability and low satiation, especially since they are both measured in terms of food consumption and meal size.
The concept of sensory-specific satiety (SSS), first described by Rolls et al. (1981), integrates current pleasure and past experience. The current response may be influenced by postingestive consequences of recently consumed foods. SSS is defined as a decrease in pleasantness for foods just eaten, without a decrease in pleasantness for new foods (Rolls et al., 1981). The salient sensory mechanism may be taste, but flavor/aroma, texture, and even color may contribute as well (Johnson and Vickers, 1992; Guinard and Brun, 1998). Sensory satiety can be prolonged, lasting from up to 24 h (single exposure) to 2 weeks for repeated exposures (Kamphuis et al., 2001; Weenen et al., 2005). It is not clear if ingestion of calories is even necessary to induce sensory satiety. Drewnowski et al. (1982) had called a similar phenomenon flavor fatigue, as it applied to reduced preferences following repeated tasting without ingestion of a variety of soft drinks.
The evolutionary significance of sensory satiety may lie in variety seeking and the drive to secure a diet containing all essential nutrients (Rolls, 2007). Exposure to a wide variety of palatable foods, representing an array of tastes, flavors, and textures, has led to higher food intakes in rats (Rolls et al., 1983) and may reduce SSS in humans (Romer et al., 2006). However, studies have found that taste can have a stronger effect on SSS than fat content (Snoek et al., 2004) and texture (Guinard and Brun, 1998). A study of pleasantness ratings comparing ham sandwiches made with soft versus crusty bread and apples versus apple sauce showed that subjects could experience texture fatigue, independent of the taste fatigue. Another study of potato chips (Maier et al., 2007) showed that flavor was a strong component of SSS in salted high-fat foods; however different textures were not compared.
The distinction between palatability and satiety makes the argument that fats are highly palatable but have little impact on satiation (as distinct from satiety). However, in purely practical terms, palatability and satiation are inextricably linked since both are measured in terms of meal size and food consumption. Studies have failed to show differences in SSS profiles between obese subjects and lean controls (Snoek et al., 2004; Brondel et al., 2007).
The newer distinction between wanting and liking attempts to account for the fact that the obese are equally sensitive to taste sensations and like high-fat foods not more that lean controls, based on hedonic ratings and self-reports. Yet the obese appear more susceptible (or less resistant) to the drive to eat, that is, to the wanting component of appetite for energy-dense high-fat foods. Judging by published reports, this wanting seems to be unconscious and distinct from cognitive, conscious wanting, and may be related to the postingestive effects of eating pleasurable food itself, rather than oronsensory stimulation (Berridge, 2004; Mela, 2006). In addition to liking and wanting, it is evident that learned behaviors (Herman et al., 2005; Brunstrom, 2007), as well as external associations and cues (cultural background, time, and social environment for example) also modulate eating behavior.
11.5. FAT PREFERENCES AND AVERSIONS IN EATING DISORDERS, STRESS, AND OVERWEIGHT
11.5.1. Eating Disorders and Stress
Stress and eating disorders have been associated with food cravings and food aversions. Stress (Torres and Nowson, 2008), dietary restraint (Drewnowski et al., 1987b; Drewnowski, 1991b) and concerns with health, and body image or body weight (Bowen et al., 2003) all seem to have an impact on the selection of sweet or high-fat foods.
The most dramatic effects are observed in eating disorders. Studies in anorectic and bulimic patients showed how sugar and fat preference can be used as a biological marker to discriminate between clinical patient populations at extremes of body weight. Anorectic patients exhibit both carbohydrate and fat phobia. While giving high sensory preference ratings to intensely sweet solutions of sugar in water, anorectic patients viewed sweet foods as forbidden in their regular diets. By contrast, sensory preferences for fat were extremely low. Anorectic patients disliked mixtures of milk, cream, and sugar and avoided both meat and milk products in their diets. The avoidance of high-calorie foods was most likely linked to the avoidance of fat (Drewnowski et al., 1987b, 1988). Whether this fat phobia was physiologically or psychologically based was unclear.
Female patients suffering from bulimia nervosa showed a similar sensory and preference profile (Drewnowski, 1989b). When asked to rate the sweetness, creaminess, and preferences for mixtures of milk cream, and sugar, they showed the same ability to detect increasing sucrose concentration as controls and enhanced sensory response to fat. They too showed an aversion to sensory stimuli that were sweet and rich in fat (Drewnowski et al., 1987a; Sunday and Halmi, 1990). The application of the RSM technique (discussed earlier), showed that anorectic-restrictor and anorectic-bulimic patients liked sweet stimuli but disliked those with a high-fat content (Drewnowski et al., 1987a,b).
Dietary restraint that is concerned with weight or dieting, can also influence reported food preferences. Tuorila et al. (2001) showed that women with higher restraint scores chose fat-free versions of a high sugar/high-fat food (fudge). The choices were further modulated by the hedonic quality of the fudge and by the subjects’ perceived hunger. By contrast, obese patients with high restraint scores showed elevated preferences for the taste of fat (Elfhag and Erlanson-Albertsson, 2006). Drewnowski and Holden-Wiltse (1992) recruited 37 obese female weight-cyclers, and classified them as low-flux cyclers (weight changes between 0 and 5.5 kg over the last year) or high-flux cyclers (weight changes between 5.6 and 30 kg). The high-flux group showed higher preference ratings for nine ice creams of varying sugar and fat content than did the low-flux group and showed higher preference ratings for sweet desserts on a food preference questionnaire. By contrast, the two groups did not differ in their preference ratings for five sucrose solutions. The authors concluded that fat, not sugar, preferences were more closely tied to the weight-cycling syndrome.
Exploring the relationship between stress and eating behavior, Torres and Nowson (2008) identified sweet, high-fat foods as those that were most likely to be consumed under stressful conditions. Although the number of human studies was very limited, all five showed that both chronic and acute stressors induced the consumption of M&M candy, peanuts, chocolate, and ice cream by obese subjects. However, the effects of obesity and stress may have been confounded; stress was measured only with subjective rather than physiologic measures; and the studies were conducted in the laboratory or under free-living conditions.
Despite these limitations, there seems to be some agreement that acute and chronic stressors may have differential effects on food consumption. Only chronic stressors are associated with higher preference for energy-dense foods, which may be mediated by an increase in circulating cortisol (Torres and Nowson, 2008). Current studies in animals confirm that stress selectively elevates the intake of a preferred high-fat diet in mice (Teegarden and Bale, 2008). These findings prompt the question of whether a higher preference for fat would ensure better preparedness for the fight or fiight response under stress conditions in mammals, evolutionary enhancing their survival. Taken together, these data point out the strong influence of the reward system in food intake (dys)regulation.
11.5.2. Fat Preference in Smoking Cessation, Diabetes, and Pregnancy
Smoking cessation has been associated with increased appetite for sweet and high-fat foods and with weight gain. Perkins et al. (1990) showed that long-term exposure to nicotine suppressed hedonic ratings for sugar and fat. In that study, 10 smokers and 10 nonsmokers (all male) rated sweetened mixtures of milk, cream, and sugar containing between 0% and 20% sucrose and 0.1%–37.6% fat (Drewnowski and Greenwood, 1983). Acute nicotine exposure decreased perceived intensity of fat taste, but had no impact on sweet taste perception or on hedonic ratings for the stimuli. However, current smokers liked sweet/fat mixtures less regardless of nicotine exposure. The authors concluded that long-term exposure to nicotine seems to suppress sensory preferences for sugar and fat and may play a role in weight control.
Pregnancy has been associated with changes in taste perception, food cravings, and food aversions (Pope et al., 1992; Drewnowski, 1997a; Duffy et al., 1998; Tepper and Seldner, 1999; Bartoshuk et al., 2006;). Foods typically craved by pregnant women include sweet and salty foods, notably chocolate, sweets, desserts, fruits, fruit juices, ice cream; pizza; breads, noodles, and grains; pickles and salty snacks (Pope et al., 1992). On the other hand, aversions include coffee, meats, eggs, and pizza. Whereas cravings turn to the basic tastes, sugar, and salt, aversions are formed toward foods with a strong aromatic component. The aroma of coffee, meats, and other fat-soluble volatile molecules may be a warning system denoting potential dietary danger from foods that are bitter, spoiled, and potentially toxic. In other words, the aversions are to odors, many of which are emitted by fat-containing animal foods. The mechanisms by which these taste alterations happen during pregnancy are not fully understood, but they seem to be related to hormonal fluctuations and to the support of healthy pregnancy outcomes (Frye and Demolar, 1994; Duffy et al., 1998). Given that fat is more slowly emptied from the stomach compared with carbohydrate, high-fat foods may induce more feelings of fullness or nausea than carbohydrate-rich foods.
Alterations in sweet taste perception may also occur in type 1 and type 2 diabetes mellitus (DM) (Settle, 1991; Perros et al., 1996). Very limited studies have examined changes in fat perception or fat preference under these conditions. Laitinen et al. (1991) reported no changes in preference for fatty foods (milk and cheese) during the first 3 months of dietary therapy for patients newly diagnosed with type 2 DM. After this time, preferences for fat and sugar decreased accompanied by decreased intake of such foods. These patients were on a clinical diet, which may have accelerated taste changes due to imposed dietary restrictions. Diabetes may not affect preferences for dietary fats. Campbell et al. (1994) alternated 10 patients with diabetes mellitus between a high-monounsaturated fatty acid (MUFA) diet and a high-carbohydrate diet as advised for DM, for a period of 2 weeks each. Both diets were accepted equally well.
11.5.3. Fat Taste Response and Overweight
There is agreement that combinations of sugar and fat have unique hedonic properties and are able to activate the system of reward (Drewnowski, 1991a, 1997b). The question is whether hedonic qualities of such products are a direct cause of overeating and weight gain. Earlier studies on sensory properties of sugar and fat mixtures and the hedonic response showed that the sugar-to-fat preference ratios were tied to extremes of body weight. Massively obese women preferred fat to sugar, whereas anorectic women preferred sugar to fat, while showing reduced hedonic responses overall. However, preferences for dietary sugars and fats have not been directly linked to weight gain in any longitudinal cohort study. Rather, links between taste responsiveness, hedonic preferences, and body weight are still based on a series of small, clinical, cross-sectional studies.
Patients with BMI in the range of morbid obesity preferred stimuli richer in fat than in sugar, while anorectic patients preferred intensively sweet stimuli and reported aversion to dietary fat (Drewnowski et al., 1985, 1987a,b). Mela and Sacchetti (1991) further reported a direct association between percent fat in most preferred foods (mashed potato and scrambled eggs) and the participants’ own percent body fat. Furthermore, a retrospective history of weight cycling was associated with higher preferences for fatty, sweet foods such as ice cream and cakes (Drewnowski and Holden-Wiltse, 1992).
Subsequent studies attempted to identify fat-seeking phenotypes using clusters of characteristics as opposed to single traits (Cooling and Blundell, 1998; Blundell et al., 2005). Such clusters included a strong palatability and weak satiety response to fats; no suppression in liking for energy-dense foods after a meal, and high scores for hunger and disinhibition on the Three-Factor Eating Questionnaire (Blundell et al., 2005). The hypothesis was that the two phenotypes (high fat and low fat) would differ in their ability to distinguish between high- and low-fat foods and in their fat consumption (Cooling and Blundell, 1998). Data analyses showed that the high-fat or thrifty phenotypes had a long-term positive energy balance of approx. 0.5 MJ or 120 kcal/day.
However, the two phenotypes did not differ in their pleasantness ratings for fat or sugar, nor in their ability to estimate fat content based on ratings of creaminess (Cooling and Blundell, 2001). That study compared responses of the two groups of young males (n = 8 per group) to 18 solutions varying in sugar and fat content (Drewnowski et al., 1987b). Both groups correctly identified increasing levels of creaminess and sweetness with increasing fat and sugar concentrations, prompting the question of what is it that causes individuals with low-fat phenotypes to choose different foods from individuals with high-fat phenotypes, when their taste preferences do not seem to differ (Cooling and Blundell, 2001).
Obese men and women appear to differ in their food preferences (Drewnowski, 1997a). A clinical sample of 386 obese women and 93 obese men identified beef steaks and roasts, doughnuts, cookies, and cake as the foods they liked the most and had most trouble with when it came to weight control (Drewnowski et al., 1992b).
Whereas obese women typically preferred fat and sugar mixtures such as chocolate, cookies, doughnuts, and cakes, obese men tended to prefer fat and protein mixtures such as meats, fish, and eggs (Drewnowski et al., 1992b). These patterns of food preferences corresponded to the patterns of food consumption in the broader population (CDC, 2004; Serra-Majem et al., 2007). Data for United Kingdom in 2003 showed that men derived a higher proportion (25%) of their average daily total fat intake from meat and meat products than did women (20%); but similar amounts from spreads, cereals and cereal products, milk and milk products, and potatoes and savory snacks (Henderson et al., 2003). Within food groups, men consumed slightly more bacon, ham, sausages, meat pies, and pastries than women but the differences were small; by contrast, women tended to consume more chocolate, but again the differences were small (1% of total daily fat intake).
In short, differences in fat preference by men and women exist and are more prominent in the obese population. Overall, it seems that men of all sizes tend to derive more fat from savory sources of food; while women (more so if obese) tend to derive more fat from sugar/fat mixtures such as chocolates and desserts. As all preferred foods in the study with the obese population contained fat, a “fat tooth” rather than a “sweet tooth” may the characteristic feature of human obesity (Drewnowski, 1995).
11.6. CONCLUDING REMARKS
The aroma, texture, and mouthfeel of foods are often influenced by their fat content. Human preferences for fat in foods shape dietary choices and may affect energy balance and the long-term regulation of body weight. Understanding the mechanisms by which we detect, perceive, and enjoy fat-rich foods may help us predict food choices and their multiple influences on diets and health.
This chapter explored the perceptions and preferences for dietary fats, using findings from genetic, physiological, and behavioral research on food preference. Traditionally, studies on the sensory perception of fat have focused on diverse aspects of food texture; however, the response to fats also depends on taste and olfaction and on synergistic interactions between fats and other food components. Given that fat in foods can give rise to a variety of textures and flavors, it can be difficult to decide which particular percept is most closely associated with fat content. Fat perception is also influenced by physical form (liquid or solid) and by the presence of other gustatory cues, such as sweetness.
Genetic, developmental, attitudinal, and physiological factors also influence fat preferences. Fat preferences are shaped by postchildhood experiences, remain stable in adult life and do not decline with age. Hedonic preferences for fat-containing foods among adults are influenced by multiple sensory stimuli. In particular, studies have identified a hedonic synergy in sugar/fat mixtures. Mixtures of cream and sugar were universally better liked than sweetened skim milk or unsweetened heavy cream (Drewnowski and Greenwood, 1983). Although studies have generally failed to link sugar consumption to overweight, several cross-sectional studies have pointed to an association between overweight and excess consumption of dietary fats.
Human preferences for energy-dense sweet and high-fat foods may have evolved for reasons of survival. The relative contributions of hedonic (preference or “liking”) versus homeostatic (physiological or “wanting”) processes in determining preference and choice for high-fat foods have not been defined (Mela, 2006). The hedonic response to fat seems to be strongly linked to the endogenous opioid reward system. This reward system may be independent from motivation to eat at least in the obese population, contributing to increased energy intakes.
Our understanding of fat chemosensation has helped to model the development of a preference for dietary fats in humans. Physiological and gender variations in fat consumption need to be examined further in the context of food culture. The amount and type of fat consumed is strongly influenced by geography, culture, and cost (Perrin et al., 2002; Serra-Majem et al., 2007). Fat consumption is a classic example of how human innate preferences have been aligned with current trends in the food supply and the economics of food choice.
REFERENCES
- Allred JB. Too much of a good thing? J Am Diet Assoc. 1995;95:417–418. [PubMed: 7699181]
- Amuna P, Zotor FB. Epidemiological and nutrition transition in developing countries: impact on human health and development. Proc Nutr Soc. 2008;67:82–90. [PubMed: 18234135]
- Andrieu E, Darmon N, Drewnowski A. Low-cost diets: more energy, fewer nutrients. Eur J Clin Nutr. 2006;60:434–436. [PubMed: 16306928]
- Astorg P, Arnault N, Czernichow S, Noisette N, Galan P, Hercberg S. Dietary intakes and food sources of n-6 and n-3 PUFA in French adult men and women. Lipids. 2004;39:527–535. [PubMed: 15554151]
- Bartoshuk L, Duffy VB, Hayes JE, Moskowitz HR, Snyder DJ. Psychophysics of sweet and fat perception in obesity: Problems, solutions and new perspectives. Phil Trans R Soc. 2006;361:1137–1148. [PMC free article: PMC1642698] [PubMed: 16815797]
- Berridge KC. Motivation concepts in behavioral neuroscience. Physiol Behav. 2004;81:179–209. [PubMed: 15159167]
- Birch LL. Children’s preference for high-fat foods. Nutr Rev. 1992;50:249–255. [PubMed: 1461587]
- Birch LL. Development of food preferences. Ann Rev Nutr. 1999;19:41–62. [PubMed: 10448516]
- Blass EM. Opioids, sugar and the inherent taste of sweet: broad motivational implications. In: Dobbing J, editor. Sweetness ILSI-Nutrition Foundation Symposium. New York: Springer-Verlag; 1987. pp. 115–124.
- Blundell JE, Finlayson G. Is susceptibility to weight gain characterized by homeostatic or hedonic risk factors for overconsumption? Physiol Behav. 2004;82:21–25. [PubMed: 15234585]
- Blundell JE, Macdiarmid JI. Passive overconsumption. Fat intake and short-term energy balance. Ann NY Acad Sci. 1997;827:392–407. [PubMed: 9329770]
- Blundell JE, Burley VJ, Cotton JR, Lawton CE. Dietary fat and the control of energy intake: Evaluating the effects of fat on meal size and postmeal satiety. Am J Clin Nutr. 1993;57:772S–778S. [PubMed: 8475895]
- Blundell JE, Stubbs RJ, Golding C, et al. Resistance and susceptibility to weight gain: Individual variability in response to a high-fat diet. Physiol Behav. 2005;86:614–622. [PubMed: 16225895]
- Bowen D, Green P, Vizenor N, Vu C, Kreuter P, Rolls BJ. Effects of fat content on fat hedonics: Cognition or taste? Physiol Behav. 2003;78:247–253. [PubMed: 12576122]
- Brandt MS, Skinner EZ, Coleman JA. Texture profile method. J Food Sci. 1963;28:404–409.
- Brondel L, Romer M, Van Wymelbeke V, et al. Sensory-specific satiety with simple foods in humans: no influence on BMI? Int J Obes. 2007;31:987–995. [PubMed: 17160089]
- Brunstrom JM. Associative learning and control of human dietary behaviour. Appetite. 2007;49:268–271. [PubMed: 17197053]
- Campbell LV, Marmot PE, Dyer JA, Borkman M, Storlien LH. The high-monounsaturated fat diet as a practical alternative for NIDDM. Diabet Care. 1994;17:177–182. [PubMed: 8174444]
- Centers for Disease Control and Prevention. Trends in intake of energy and macronutrients—United States, 1971–2000. 2004. Accessed under: www
.cdc.gov/MMWR/preview /mmwrhtml/mm5304a3.htm. [PubMed: 14762332] - Chalé-Rush, Burgess JR, Mattes RD. Multiple routes of chemosensitivity to free fatty acids in humans. Am J Physiol Gastrointest Liver Physiol. 2007;292:G1206–G1212. [PubMed: 17234892]
- Chandarama K, Batterham R. Peptide YY. Curr Opin Endocrinol Diabetes Obes. 2008;15:65–72. [PubMed: 18185065]
- Chandrashekar J, Hoon MA, Ryba NJP, Zuker CS. The receptors and cells for mammalian taste. Nature. 2006;444:288–294. [PubMed: 17108952]
- Cooling J, Blundell JE. Are high-fat and low-fat consumers distinct phenotypes? Differences in the subjective and behavioral response to energy and nutrient challenges. Eur J Clin Nutr. 1998;52:193–201. [PubMed: 9537305]
- Cooling J, Blundell JE. High-fat versus low-fat phenotypes: Habitual eating of high- and low-fat foods not related to taste preference for fat. Eur J Clin Nutr. 2001;55:1016–1021. [PubMed: 11641752]
- Cooper HR. Texture in dairy products and its sensory evaluation. In: Moskowitz HR, editor. Food Texture. Vol. 1. New York: Marcel Dekker Inc; 1987. pp. 251–272.
- Cooper SJ, Jackson A, Kirkham TC, et al. Endorphins, opiates and food intake. In: Rogers RJ, Cooper SJ, editors. Endorphins, Opiates and Behavioral Processes. New York: Willey & Sons; 1988. pp. 143–186.
- Cox DN, Perry L, Moore PB, Vallis L, Mela DJ. Sensory and hedonic associations with macronutrient and energy intakes of lean and obese consumers. Int J Obes Relat Metab Disord. 1999;23:403–410. [PubMed: 10340819]
- Cussler EL, Kokini JL, Weinheimer RL, Moskowitz HR. Food texture in the mouth. Food Technol. 1979;33:89–92.
- Davis C, Patte K, Levitan R, Reid C, Tweed S, Curtis C. From motivation to behaviour: a model of reward sensitivity, overeating, and food preferences in the risk profile for obesity. Appetite. 2007;48:12–19. [PubMed: 16875757]
- De Araujo I, Rolls ET. Representation of the human brain of food texture and oral fat. J Neurosci. 2004;24:3086–3093. [PMC free article: PMC6729847] [PubMed: 15044548]
- De Graaf. Sensory responses, food intake and obesity. In: Mela D, editor. Food, Diet and Obesity. Cambridge: Woodhead Publishing Ltd; 2005. pp. 137–159.
- Drewnowski. Fats and food texture: sensory and hedonic evaluations. In: Moskowitz HR, editor. Food Texture. Vol. 1. New York: Marcel Dekker Inc; 1987. pp. 251–272.
- Drewnowski A. Sensory preferences for fat and sugar in adolescence and adult life. Ann NY Acad Sci. 1989a;561:243–250. [PubMed: 2735681]
- Drewnowski A. Taste responsiveness in eating disorders. Ann NY Acad Sci. 1989b;575:399–409. [PubMed: 2699201]
- Drewnowski A. Dietary fats: perceptions and preferences. J Am Coll Nutr. 1990a;9:431–435. [PubMed: 2212403]
- Drewnowski A. The new fat replacements. A strategy for reducing fat consumption. Postgrad Med. 1990b;87:111–121. [PubMed: 2186394]
- Drewnowski A. Fat and sugar: Sensory and hedonic aspects of sweet, high-fat foods. In: Friedman MI, Tordoff MG, Kare MR, editors. Chemical Senses. Vol. 4. New York: Marcel Dekker, Inc; pp: 1991a. pp. 69–83.
- Drewnowski A. Obesity and eating disorders: Cognitive aspects of food preference and food aversion. Bull Psychon Soc. 1991b;29:261–264.
- Drewnowski A. Energy intake and sensory properties of food. Am J Clin Nutr. 1995;62 (Suppl):1081S–1085S. [PubMed: 7484925]
- Drewnowski A. Taste preferences and food intake. Ann Rev Nutr. 1997a;17:237–253. [PubMed: 9240927]
- Drewnowski A. Why do we like fat? J Am Diet Assoc. 1997b;97(Suppl 7):S58–S62. [PubMed: 9216570]
- Drewnowski A. Energy density, palatability, and satiety: Implications for weight control. Nutr Rev. 1998;56:347–353. [PubMed: 9884582]
- Drewnowski A. Sensory control of energy density at different life stages. Proc Nutr Soc. 2000;59:239–244. [PubMed: 10946792]
- Drewnowski A. Concept of a nutritious food: Toward a nutrient density score. Am J Clin Nutr. 2005;82:721–732. [PubMed: 16210699]
- Drewnowski A, Bellisle F. Is sweetness addictive? Nutr Bull. 2007;32(Suppl 1):52–60.
- Drewnowski A, Greenwood MR. Cream and sugar: Human preferences for high-fat foods. Physiol Behav. 1983;30:629–633. [PubMed: 6878464]
- Drewnowski A, Holden-Wiltse J. Taste responses and food preferences in obese women: Effects of weight cycling. Int J Obes. 1992;16:639–648. [PubMed: 1328087]
- Drewnowski A, Popkin BM. The nutrition transition: new trends in the global diet. Nutr Rev. 1997;55:31043. [PubMed: 9155216]
- Drewnowski A, Rock CL. The influence of genetic taste markers on food acceptance. Am J Clin Nutr. 1995;62:506–511. [PubMed: 7661111]
- Drewnowski A, Schwartz M. Invisible fats: Sensory assessment of sugar/fat mixtures. Appetite. 1990;14:203–217. [PubMed: 2369116]
- Drewnowski A, Grinker JA, Hirsch J. Obesity and flavor perception: Multidimensional scaling of soft drinks. Appetite. 1982;3:361–368. [PubMed: 7168568]
- Drewnowski A, Brunzell JD, Sande K, Iverius PH, Greenwood MRC. Sweet tooth reconsidered: Taste responsiveness in human obesity. Physiol Behav. 1985;35:617–622. [PubMed: 4070436]
- Drewnowski A, Bellisle F, Aimez P, Remy B. Taste and bulimia. Physiol Behav. 1987a;41:621–626. [PubMed: 3441532]
- Drewnowski A, Halmi KA, Pierce B, Gibbs J, Smith GP. Taste and eating disorders. Am J Clin Nutr. 1987b;46:442–450. [PubMed: 3630963]
- Drewnowski A, Pierce B, Halmi KA. Fat aversion in eating disorders. Appetite. 1988;10:119–131. [PubMed: 3164990]
- Drewnowski A, Shrager EE, Lipsky C, Stellar E, Greenwood MRC. Sugar and fat: Sensory and hedonic evaluations of liquid and solid foods. Physiol Behav. 1989;45:177–183. [PubMed: 2727131]
- Drewnowski A, Krahn DD, Demitrack MA, et al. Taste responses and preferences for sweet high-fat foods: evidence for opioid involvement. Physiol Behav. 1992a;51:371–379. [PubMed: 1313591]
- Drewnowski A, Kurth C, Holden-Wiltse J, Saari J. Food preferences in human obesity: Carbohydrates versus fats. Appetite. 1992b;18:207–221. [PubMed: 1510463]
- Drewnowski A, Krahn DD, Demitrack MA, Nairn K, Gosnell BA. Naloxone, an opiate blocker, reduces the consumption of sweet high-fat foods in obese and lean female binge eaters. Am J Clin Nutr. 1995;61:1206–1212. [PubMed: 7762518]
- Drewnowski A, Henderson SA, Shore AB, Fischler C, Preziosi P, Hercberg S. Diet quality and dietary diversity in France: Implications for the French paradox. J Am Diet Assoc. 1996;96:663–669. [PubMed: 8675909]
- Duffy VB, Bartoshuk LM, Striegel-Moore R, Rodin J. Taste changes across pregnancy. Ann NY Acad Sci. 1998;855:805–809. [PubMed: 9929689]
- Elfhag K, Erlanson-Albertsson C. Sweet and fat taste preference in obesity have different associations with personality and eating behavior. Physiol Behav. 2006;88:61–66. [PubMed: 16624348]
- Engelen L, Van der Bilt A. Oral physiology and texture perception of semisolids. J Text Stud. 2008;39:83–113.
- Finlayson G, King N, Blundell JE. Is it possible to dissociate ‘liking’ and ‘wanting’ for foods in humans? A novel experimental procedure. Physiol Behav. 2007;90:36–42. [PubMed: 17052736]
- Folkenberg DM, Martens M. Sensory properties of low fat yoghurts. Part B: Hedonic evaluations of plain yoghurts by consumers correlated to fat content, sensory profile and consumer attitudes. Milchwissenschaft-Milk Sci Int. 2003;58:154–157.
- Frye CA, Demolar GL. Menstrual cycle and sex differences influence salt preference. Physiol Behav. 1994;55:193–197. [PubMed: 8140168]
- Gaillard D, Laugerette F, Darcel N, et al. The gustatory pathway is involved in CD36-mediated orosensory perception of long-chain fatty acids in the mouse. FASEB J. 2008;22:1458–1468. [PubMed: 18162488]
- Gessner PK. Isobolographic analysis of interactions: An update on applications and utility. Toxicology. 1995;105:161–179. [PubMed: 8571354]
- Gilbertson TA, Fontenot DT, Liu L, Zhang H, Monroe WT. Fatty acid modulation of K+ channels in taste receptor cells: Gustatory cues for dietary fat. Am J Physiol. 1997;27:C1203–C1210. [PubMed: 9142845]
- Gilbertson TA, Liu L, Kim I, Burks CA, Hansen DR. Fatty acid responses in taste cells from obesity-prone and -resistant rats. Physiol Behav. 2005;86:681–690. [PubMed: 16249010]
- Goldfield GS, Lorello C, Doucet E. Methylphenidate reduces energy intake and dietary fat intake in adults: A mechanism of reduced reinforcing value of food? Am J Clin Nutr. 2007;86:308–315. [PubMed: 17684199]
- Guinard J-X, Brun P. Sensory-specific satiety: Comparison of taste and texture effects. Appetite. 1998;31:141–157. [PubMed: 9792729]
- Hamosh M. Lingual and gastric lipases. Nutrition. 1990;6:421–428. [PubMed: 2134569]
- Hansen L, Rose MS. Sensory acceptability is inversely related to development of fat rancidity in bread made from stored flour. J Am Diet Assoc. 1996;96:792–793. [PubMed: 8683011]
- Heilmann S, Hummel TA. A new method for comparing orthonasal and retronasal olfaction. Behav Nuerosci. 2004;118:412–419. [PubMed: 15113268]
- Henderson L, Gregory J, Irving K. Energy, Protein, Carbohydrate, Fat and Alcohol Intake. Vol. 2. London: TSO; 2003. The National Diet and Nutrition Survey: Adults Aged 19–64 Years.
- Herman CP, Polivy J, Leone T. The psychology of overeating. In: Mela D, editor. Food, Diet and Obesity. Cambridge: Woodhead Publishing Ltd; 2005. pp. 114–136.
- Johnson J, Vickers Z. Factors influencing sensory-specific satiety. Appetite. 1992;19:15–31. [PubMed: 1416934]
- Johnson SL, McPhee L, Birch LL. Conditioned preferences: Young children prefer flavors associated with high dietary fat. Physiol Behav. 1991;50:1245–1251. [PubMed: 1798782]
- Kamphuis MMJW, Westerterp-Plantenga MS, Saris WHM. Fat-specific satiety in humans for fat high in linoleic acid vs. fat high in oleic acid. Eur J Clin Nutr. 2001;55:499–508. [PubMed: 11423927]
- Kokini KL, Kadane JB, Cussler EL. Liquid texture perceived in the mouth. J Text Stud. 1977;8:195–218.
- Laitinen JH, Tuorila HM, Uusitupa MI. Changes in hedonic responses to sweet and fat in recently diagnosed non-insulin-dependent diabetic patients during diet therapy. Eur J Clin Nutr. 1991;45:393–400. [PubMed: 1760999]
- Laugerette F, Passilly-Degrace, Patris B, et al. CD36 involvement in orosensory detection of dietary lipids, spontaneous fat preference, and digestive secretions. J Clin Invest. 2005;115:3177–3184. [PMC free article: PMC1265871] [PubMed: 16276419]
- Maier A, Vickers Z, Jeffrey Inman J. Sensory-specific satiety, its crossovers, and subsequent choice of potato chip flavors. Appetite. 2007;49:419–428. [PubMed: 17395335]
- Mattes RD. Oral fat exposure increases the first phase triacylglycerol concentration due to release of stored lipid in humans. J Nutr. 2002;132:3656–3662. [PubMed: 12468603]
- Mattes RD. Fat taste and lipid metabolism in humans. Physiol Behav. 2005;86:691–697. [PubMed: 16249011]
- Mela DJ. Sensory assessment of fat content in fluid dairy products. Appetite. 1988;10:37–44. [PubMed: 3355125]
- Mela DJ. Eating for pleasure of just wanting to eat? Reconsidering sensory hedonic responses as a driver of obesity. Appetite. 2006;47:10–17. [PubMed: 16647788]
- Mela DJ, Christensen CM. Sensory assessment of oiliness in a low moisture food. J Sensory Stud. 1987;2:273–281.
- Mela DJ, Rogers PJ. Food, Eating & Obesity: The Psychobiological Basis of Appetite and Weight Control. London: Chapman & Hall; 1998.
- Mela DJ, Sacchetti DA. Sensory preferences for fats: Relationships with diet and body composition. Am J Clin Nutr. 1991;53:908–915. [PubMed: 2008871]
- Mela DJ, Langley KR, Martin A. No effect of oral or sample temperature on sensory assessment of fat content. Physiol Behav. 1994a;56:655–658. [PubMed: 7800728]
- Mela DJ, Langley KR, Martin A. Sensory assessment of fat content: Effect of emulsion and subject characteristics. Appetite. 1994b;22:67–81. [PubMed: 8172491]
- Mendoza JA, Drewnowski A, Christakis DA. Dietary energy density is associated with obesity and the metabolic syndrome in U.S. adults. Diabet Care. 2007;30:974–979. [PubMed: 17229942]
- Mindell S, Smith GP, Greenberg D. Corn oil and mineral oil stimulate sham feeding in rats. Physiol Behav. 1990;48:283–287. [PubMed: 2255732]
- Mizushige T, Kawada T, Inoue K, Fushiki T. Involvement of β-endorphin in the formation of preference for dietary fat. Chem Senses. 2006;31:J17.
- Mizushige T, Inoue K, Fushiki T. Why is fat so tasty? Chemical reception of fatty acid on the tongue. J Nutr Sci Vitaminol. 2007;53:1–4. [PubMed: 17484372]
- Nasser JA, Kissleff HR, Boozer CN, Chou CJ, Pi-Sunyer FX. PROP taster status and oral fatty acid perception. Eating Behav. 2001;2:237–245. [PubMed: 15001033]
- Perkins KA, Epstein LH, Stiller RL, Fernstrom MH, Sexton JE, Jacob RG. Perception and hedonics of sweet and fat taste in smokers and nonsmokers following nicotine intake. Pharmacol Biochem Behav. 1990;35:671–676. [PubMed: 2339157]
- Perrin AE, Simon C, Hedelin G, Arveiler D, Schaffer P, Schlienger JL. Ten-year trends of dietary intake in middle-aged French population: relationship with educational level. Eur J Clin Nutr. 2002;56:393–401. [PubMed: 12001009]
- Perros P, MacFarlane TW, Counsell C, Frier BM. Altered taste sensation in newly diagnosed NIDDM. Diabet Care. 1996;19:768–770. [PubMed: 8799637]
- Peryam DR, Pilgrim PJ. Hedonic scale method for measuring food preferences. Food Technol. 1957;11:9–14.
- Pittman DW, Smith KR, Crawley ME, Corbin CH, Hansen DR, Watson KJ, Gilbertson TA. Orosensory detection of fatty acids by obesity-prone and obesity-resistant rats: Strain and sex differences. Chem Senses. 2008;33:449–460. [PubMed: 18372387]
- Pope JF, Skinner JD, Carruth BR. Cravings and aversions of pregnant adolescents. J Am Diet Assoc. 1992;92:1479–1482. [PubMed: 1452960]
- Popkin BM, Siega-Riz AM, Haines PS, Jahns L. Where is the fat? Trends in U.S. diets 1965–1996. Prev Med. 2001;32:245–254. [PubMed: 11277682]
- Rankin KM, Mattes RD. Role of food familiarity and taste quality in food preferences of individuals with Prader-Willi syndrome. Int J Obes. 1996;20:759–762. [PubMed: 8856400]
- Refsgaard HH, Brockhoff PM, Jensen B. Free polyunsaturated fatty acids cause taste deterioration of salmon during frozen storage. J Agric Food Chem. 2000;48:792–793. [PubMed: 10956102]
- Reid LD. Endogenous opioid peptides and regulation of drinking and feeding. Am J Clin Nutr. 1985;42:1099–1132. [PubMed: 2865892]
- Rissanen A, Hakala P, Lissner L, Mattlar CE, Koskenvuo M, Rönnemaa T. Acquired preference especially for dietary fat and obesity: A study of weight-discordant monozygotic twin pairs. Int J Obes. 2002;26:973–977. [PubMed: 12080452]
- Rolls BJ. Carbohydrates, fats and satiety. Am J Clin Nutr. 1995;61(Suppl):960S–967S. [PubMed: 7900695]
- Rolls ET. Smell, taste, texture and temperature multimodal representations in the brain, and their relevance to the control of appetite. Nutr Rev. 2004;62:S193–S204. [PubMed: 15630935]
- Rolls ET. Sensory processing in the brain related to the control of food intake. Proc Nutr Soc. 2007;66:96–112. [PubMed: 17343776]
- Rolls BJ, Rolls ET, Rowe EA, Sweeney K. Sensory-specific satiety in man. Physiol Behav. 1981;27:137–142. [PubMed: 7267792]
- Rolls BJ, van Duijenvoorde PM, Rowe EA. Variety in the diet enhances intake in a meal and contributes to the development of obesity in the rat. Phys Behav. 1983;31:21–27. [PubMed: 6634975]
- Romer M, Lehrner J, van Wymelbeke V, Jiang T, Deecke L, Brondel L. Does modification of olfactory-gustatory stimulation diminish sensory-specific satiety in humans? Physiol Behav. 2006;87:469–477. [PubMed: 16458336]
- Salbe AD, Del Parigi A, Pratley RE, Drewnowski A, Tataranni PA. Taste preferences and body weight changes in an obesity-prone population. Am J Clin Nutr. 2004;79:372–378. [PubMed: 14985209]
- Sclafani A. Oral and postoral determinants of food reward. Physiol Behav. 2007;81:773–779. [PubMed: 15234183]
- Schiffman SS, Dackis C. Taste of nutrients: Amino acids, vitamins, and fatty acids. Percept Psychophys. 1975;17:140–146.
- Schiffman SS, Graham BG, Sattely-Miller EA, Warwick ZS. Orosensory perception of dietary fat. Curr Dir Psychol Sci. 1998;7:137–143.
- Serra-Majem L, Ribas-Barba L, Salvador G, et al. Trends in energy and nutrient intake and risk of inadequate intakes in Catalonia, Spain (1992–2003). Public Health Nutr. 2007;10(11A):1354–1367. [PubMed: 17988407]
- Settle RG. The chemical senses in diabetes mellitus. In: Getchell TV, Doty RL, Bartoshuk LM, Snow JB JR, editors. Smell and Taste in Health and Disease. New York: Raven Press; 1991. pp. 829–844.
- Snoek H, Huntjens L, van Gemert LJ, de Graaf C, Weenen H. Sensory-specific satiety in obese and normal-weight women. Am J Clin Nutr. 2004;80:823–831. [PubMed: 15447886]
- Sørensen LB, Møller P, Flint A, Martens M, Raben A. Effect of sensory perception of foods on appetite and food intake: a review of studies on humans. Int J Obes. 2003;27:1152–1166. [PubMed: 14513063]
- Spielman AI, D’Abundo S, Field RB, Schmale H. Protein analysis of human von Ebner saliva and a method for its collection from the foliate papillae. J Dent Res. 1993;72:1331–1335. [PubMed: 7689601]
- Sunday SR, Halmi KA. Taste perceptions and hedonics in eating disorders. Physiol Behav. 1990;48:587–594. [PubMed: 2082356]
- Szczesniak AS. Classification of textural characteristics. J Food Sci. 1963;28:385–389.
- Szczesniak AS. Consumer awareness of texture and other food attributes: II. J Texture Stud. 1971;2:196–206. [PubMed: 28371983]
- Szczesniak AS, Brandt MA, Friedman HH. Development of standard rating scales for mechanical parameters of texture and correlations between the objective and the sensory methods of texture evaluation. J Food Sci. 1963;28:397–403.
- Teegarden S, Bale TL. Effects of stress on dietary preference and intake are dependent on access and stress sensitivity. Physiol Behav. 2008;93:713–723. [PMC free article: PMC2483328] [PubMed: 18155095]
- Tepper BJ, Seldner AC. Sweet taste and intake of sweet foods in normal pregnancy and pregnancy complicated by gestational diabetes mellitus. Am J Clin Nutr. 1999;70:277–284. [PubMed: 10426706]
- Torres SJ, Nowson C. Relationship between stress, eating behaviour, and obesity. Nutrition. 2008;23:887–894. [PubMed: 17869482]
- Tuorila H, Pangborn RM. Prediction of reported consumption of selected fat-containing foods. Appetite. 1988;11:81–95. [PubMed: 3239966]
- Tuorila H, Kramer FM, Engell D. The choice of fat-free vs. regular-fat fudge: the effects of liking for the alternative and the restrain status. Appetite. 2001;37:27–32. [PubMed: 11562155]
- Ward SJ, Dykstra LA. The role of CB1 receptors in sweet versus fat reinforcement: Effect of CB1 receptor deletion, CB1 receptor antagonism (SR141716A) and CB1 receptor agonism (CP-55940). Behav Pharmacol. 2005;16:381–388. [PubMed: 16148442]
- WHO. World Health Organ Tech Rep Ser. Vol. 916. Geneva: World Health Organization; 2003. 2003. Diet, nutrition and the prevention of excess weight gain and obesity Report of a joint WHO/FAO expert consultation.
- Warwick ZS, Schiffman SS. Sensory evaluation of fat-sucrose and fat-salt mixtures: Relationship to age and weight status. Physiol Behav. 1990;48:633–636. [PubMed: 2082362]
- Warwick ZS, Hall WG, Pappas TN, Schiffman SS. Taste and smell sensations enhance the satiating effect of both a high-carbohydrate and a high-fat meal in humans. Physiol Behav. 1993;53:553–563. [PubMed: 8451323]
- Weenen H, Satfleu A, de Graaf C. Dynamic aspects of liking: Post-prandial persistence of sensory specific satiety. Food Qual Pref. 2005;16:528–535.
- Will M, Franzblau E, Kelly AE. The amygdala is critical for opioid-mediated binge eating of fat. Neurosci Res. 2004;15:1857–1860. [PubMed: 15305124]
- Yeomans M. Taste, palatability, and the control of appetite. Proc Nutr Soc. 1998;57:609–615. [PubMed: 10096124]
- Yeomans MR. Olfactory influences on appetite and satiety in humans. Physiol Behav. 2006;89:10–14. [PubMed: 16712883]
- Yeomans MR, Gray RW. Selective effects of naltrexone on food pleasantness and intake. Physiol Behav. 1996;60:439–446. [PubMed: 8840904]
- Yeomans MR, Gray RW, Mitchell CJ, True S. Independent effects of palatability and within-meal pauses on intake and appetite ratings in human volunteers. Appetite. 1997;29:61–76. [PubMed: 9268426]
- Yeomans MR, Lee MD, Gray RW, French SJ. Effects of test-meal palatability on compensatory eating following disguised fat and carbohydrate preloads. Int J Obes. 2001;25:1215–1224. [PubMed: 11477507]
- Yeomans MR, Blundell JE, Leshem M. Palatability: Response to nutritional need or need-free stimulation of appetite? Brit J Nutr. 2004;92(Suppl 1):S3–S14. [PubMed: 15384315]
- Yoshikawa S, Nishumaru S, Yoshida M. Collection and classification of words for description in food texture, III: Classification by multivariate analysis. J Texture Stud. 1970;1:452–463. [PubMed: 28370014]
- Zheng H, Patterson LM, Berthoud HR. Orexin signalling in the ventral tegmental area is required for high-fat appetite induced by opioid stimulation of the nucleus accumbens. J Neurosci. 2007;27:11075–11082. [PMC free article: PMC6672863] [PubMed: 17928449]
- Review Preference for High-Fat Food in Animals.[Fat Detection: Taste, Texture,...]Review Preference for High-Fat Food in Animals.Manabe Y, Matsumura S, Fushiki T. Fat Detection: Taste, Texture, and Post Ingestive Effects. 2010
- Review Heritable Variation in Fat Preference.[Fat Detection: Taste, Texture,...]Review Heritable Variation in Fat Preference.Reed DR. Fat Detection: Taste, Texture, and Post Ingestive Effects. 2010
- Review Why do we like fat?[J Am Diet Assoc. 1997]Review Why do we like fat?Drewnowski A. J Am Diet Assoc. 1997 Jul; 97(7 Suppl):S58-62.
- Low-energy-density diets are associated with high diet quality in adults in the United States.[J Am Diet Assoc. 2006]Low-energy-density diets are associated with high diet quality in adults in the United States.Ledikwe JH, Blanck HM, Khan LK, Serdula MK, Seymour JD, Tohill BC, Rolls BJ. J Am Diet Assoc. 2006 Aug; 106(8):1172-80.
- Review Role of the Gustatory System in Fatty Acid Detection in Rats.[Fat Detection: Taste, Texture,...]Review Role of the Gustatory System in Fatty Acid Detection in Rats.Pittman DW. Fat Detection: Taste, Texture, and Post Ingestive Effects. 2010
- Human Perceptions and Preferences for Fat-Rich Foods - Fat DetectionHuman Perceptions and Preferences for Fat-Rich Foods - Fat Detection
- Wnt/β-Catenin Signaling in Vertebrate Posterior Neural DevelopmentWnt/β-Catenin Signaling in Vertebrate Posterior Neural Development
- Arterial, Venous, and Microvascular Hemostasis/Thrombosis - Platelet-Vessel Wall...Arterial, Venous, and Microvascular Hemostasis/Thrombosis - Platelet-Vessel Wall Interactions in Hemostasis and Thrombosis
- Platelet Aggregation - Platelet-Vessel Wall Interactions in Hemostasis and Throm...Platelet Aggregation - Platelet-Vessel Wall Interactions in Hemostasis and Thrombosis
- How Tobacco Smoke Causes Disease: The Biology and Behavioral Basis for Smoking-A...How Tobacco Smoke Causes Disease: The Biology and Behavioral Basis for Smoking-Attributable Disease
Your browsing activity is empty.
Activity recording is turned off.
See more...