By agreement with the publisher, this book is accessible by the search feature, but cannot be browsed.
NCBI Bookshelf. A service of the National Library of Medicine, National Institutes of Health.
Alberts B, Johnson A, Lewis J, et al. Molecular Biology of the Cell. 4th edition. New York: Garland Science; 2002.
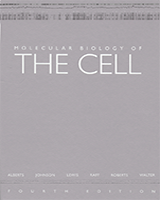
Molecular Biology of the Cell. 4th edition.
Show detailsThe linkage of the extracellular matrix to the cell requires transmembrane cell adhesion proteins that act as matrix receptors and tie the matrix to the cell's cytoskeleton. Although we have seen that some transmembrane proteoglycans function as co-receptors for matrix components, the principal receptors on animal cells for binding most extracellular matrix proteins—including collagens, fibronectin, and laminins—are the integrins. These constitute a large family of homologous transmembrane, cell-matrix adhesion receptors. In blood cells, as we have seen, integrins also serve as cell-cell adhesion molecules, helping the cells bind to other cells, as well as to the extracellular matrix.
Integrins, like other cell adhesion molecules, differ from cell-surface receptors for hormones and for other extracellular soluble signal molecules in that they usually bind their ligand with lower affinity and are usually present at about tenfold to a hundredfold higher concentration on the cell surface. If the binding were too tight, cells would presumably become irreversibly glued to the matrix and would be unable to move—a problem that does not arise if attachment depends on large numbers of weak adhesions. This is an example of the “Velcro principle” mentioned earlier. Like other transmembrane cell adhesion proteins, however, integrins do more than just attach a cell to its surroundings. They also activate intracellular signaling pathways that communicate to the cell the character of the extracellular matrix that is bound.
Integrins Are Transmembrane Heterodimers
Integrins are crucially important because they are the main receptor proteins that cells use to both bind to and respond to the extracellular matrix. An integrin molecule is composed of two noncovalently associated transmembrane glycoprotein subunits called α and β (Figure 19-64; see also Figure 19-12B). Because the same integrin molecule in different cell types can have different ligand-binding specificities, it seems that additional cell-type-specific factors can interact with integrins to modulate their binding activity.
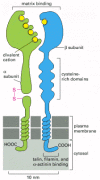
Figure 19-64
The subunit structure of an integrin cell-surface matrix receptor. Electron micrographs of isolated receptors suggest that the molecule has approximately the shape shown here, with the globular head projecting more than 20 nm from the lipid bilayer. By (more...)
The binding of integrins to their ligands depends on extracellular divalent cations (Ca2+ or Mg2+, depending on the integrin), reflecting the presence of divalent-cation-binding domains in the extracellular part of the α and β subunits. The type of divalent cation can influence both the affinity and the specificity of the binding of an integrin to its ligands.
Many matrix proteins in vertebrates are recognized by multiple integrins. At least 8 integrins bind fibronectin, for example, and at least 5 bind laminin. A variety of human integrin heterodimers are formed from 9 types of β subunits and 24 types of α subunits. This diversity is further increased by alternative splicing of some integrin RNAs. Some of the best-studied integrins are listed in Table 19-6.
Table 19-6
Some Types of Integrins.
β1 subunits form dimers with at least 12 distinct α subunits. They are found on almost all vertebrate cells: α5β1, for example, is a fibronectin receptor and α6β1 a laminin receptor on many types of cells. Mutant mice that cannot make any β1 integrins die at implantation, whereas mice that are only unable to make the α7 subunit (the partner for β1 in muscle) survive but develop muscular dystrophy (as do mice that cannot make the laminin ligand for the α7β1 integrin).
The β2 subunits form dimers with at least four types of α subunit. They are expressed exclusively on the surface of white blood cells, where they have an essential role in enabling these cells to fight infection. The β2 integrins mainly mediate cell-cell rather than cell-matrix interactions, binding to specific ligands on another cell, such as an endothelial cell. The ligands, sometimes referred to as counterreceptors, are members of the Ig superfamily of cell-cell adhesion molecules discussed earlier. The β2 integrins enable white blood cells, for example, to attach firmly to endothelial cells at sites of infection and migrate out of the bloodstream into the infected site (see Figure 19-30B). Humans with the genetic disease called leucocyte adhesion deficiency are unable to synthesize β2 subunits. As a consequence, their white blood cells lack the entire family of β2 receptors, and they suffer repeated bacterial infections.
The β3 integrins are found on a variety of cells, including blood platelets. They bind several matrix proteins, including fibrinogen. Platelets interact with fibrinogen during blood clotting, and humans with Glanzmann's disease, who are genetically deficient in β3 integrins, bleed excessively.
Integrins Must Interact with the Cytoskeleton to Bind Cells to the Extracellular Matrix
Integrins function as transmembrane linkers (or “integrators”), mediating the interactions between the cytoskeleton and the extracellular matrix that are required for cells to grip the matrix. Most integrins are connected to bundles of actin filaments. The α6β4 integrin found in hemidesmosomes is an exception: it is connected to intermediate filaments. After the binding of a typical integrin to its ligand in the matrix, the cytoplasmic tail of the β subunit binds to several intracellular anchor proteins, including talin, α-actinin, and filamin. These anchor proteins can bind directly to actin or to other anchor proteins such as vinculin, thereby linking the integrin to actin filaments in the cell cortex. Given the right conditions, this linkage leads to a clustering of the integrins and the formation of focal adhesions between the cell and the extracellular matrix, as discussed earlier.
If the cytoplasmic domain of the β subunit is deleted using recombinant DNA techniques, the shortened integrins still bind to their ligands, but they no longer mediate robust adhesion, and they fail to cluster at focal adhesions. It seems that integrins must interact with the cytoskeleton to bind cells strongly to the matrix, just as cadherins must interact with the cytoskeleton to hold cells together efficiently. The cytoskeletal attachment may help cluster the integrins, providing a stronger aggregate bond; it may also lock the integrin in a conformation that allows the integrin to bind its ligand more tightly.
Just as cadherins can promote cell-cell adhesion without forming mature adherens junctions, integrins can mediate cell-matrix adhesion without forming mature focal adhesions. In both cases, however, the transmembrane adhesion proteins may still bind to the cytoskeleton. For integrins, this kind of adhesion occurs when cells are spreading or migrating, and it results in the formation of focal complexes. For such focal complexes to mature into the focal adhesions that are typical of many well-spread cells, the activation of the small GTPase Rho is required. The activation of Rho leads to the recruitment of more actin filaments and integrins to the contact site (discussed in Chapter 16).
Cells Can Regulate the Activity of Their Integrins
We discuss below how integrin clustering activates intracellular signaling pathways. But signaling also operates in the opposite direction: signals generated inside the cell can either enhance or inhibit the ability of integrins to bind to their ligand outside the cell (Figure 19-65). This regulation is poorly understood, but it may involve the phosphorylation of the cytoplasmic tails of the integrins, the association of the tails with activating cytoplasmic proteins, or both.
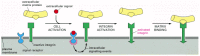
Figure 19-65
The regulation of the extracellular binding activity of a cell's integrins from within. In this example, an extracellular signal activates an intracellular signaling cascade that alters the integrin so that its extracellular binding site can now mediate (more...)
The ability of a cell to control integrin-ligand interactions from within is termed inside-out signaling. It is particularly important in platelets and white blood cells, where integrins usually have to be activated before they can mediate adhesion. In most other cells, integrins are usually maintained in an adhesion-competent state. Regulated adhesion allows white blood cells to circulate unimpeded until they are activated by an appropriate stimulus. Because the integrins do not need to be synthesized de novo, the signaled adhesion response can be rapid. Platelets, for example, are activated either by contact with a damaged blood vessel or by various soluble signal molecules. In either case, the stimulus triggers intracellular signaling pathways that rapidly activate a β3 integrin in the platelet membrane. This induces a conformational change in the extracellular domain of the integrin that enables the protein to bind the blood-clotting protein fibrinogen with high affinity. The fibrinogen links platelets together to form a platelet plug, which helps stop bleeding.
Similarly, the weak binding of a T lymphocyte to its specific antigen on the surface of an antigen-presenting cell (discussed in Chapter 24) triggers intracellular signaling pathways in the T cell that activate its β2 integrins. The activated integrins then enable the T cell to adhere strongly to the antigen-presenting cell so that it remains in contact long enough to become stimulated fully. The integrins may then return to an inactive state, allowing the T cell to disengage.
There are occasions, especially during development, when cells other than blood cells also regulate the activity of their integrins. If a constitutively active integrin (made by deleting the cytoplasmic tail of the α subunit) is expressed in a developing Drosophila embryo, for example, it disrupts normal muscle development. The muscle precursor cells expressing the activated integrin cannot disengage from the extracellular matrix and therefore cannot migrate normally.
Integrins Activate Intracellular Signaling Pathways
We have already discussed how integrins function as transmembrane linkers that connect extracellular matrix molecules to actin filaments in the cell cortex and thereby regulate the shape, orientation, and movement of cells. But the clustering of integrins at the sites of contact with the matrix (or with another cell) can also activate intracellular signaling pathways. Signaling is initiated by the assembly of signaling complexes at the cytoplasmic face of the plasma membrane, much as in signaling by conventional signaling receptors (discussed in Chapter 15).
Whereas activated integrins, like activated conventional signaling receptors, can induce global cell responses, often including changes in gene expression, activated integrins are especially adept at stimulating localized changes in the cytoplasm close to the cell-matrix contact. This may be a fundamental feature of signaling by transmembrane cell adhesion proteins in general. In the developing nervous system, for example, the growing tip of an axon is guided mainly by its responses to local adhesive (and repellent) cues in the environment that are recognized by transmembrane cell adhesion proteins. The primary effects of the adhesion proteins are thought to result from the activation of intracellular signaling pathways that act locally in the axon tip, rather than through cell-cell adhesion itself or signals conveyed to the cell body.
Many of the signaling functions of integrins depend on a cytoplasmic protein tyrosine kinase called focal adhesion kinase (FAK). Focal adhesions are often the most prominent sites of tyrosine phosphorylation in cells in culture (see Figure 17-50), and FAK is one of the major tyrosine-phosphorylated proteins found in focal adhesions (although it can also associate with conventional signaling receptors). When integrins cluster at sites of cell-matrix contact, FAK is recruited to focal adhesions by intracellular anchor proteins such as talin, which binds to the integrin β subunit, or paxillin, which binds to one type of integrin α subunit. The clustered FAK molecules cross-phosphorylate each other on a specific tyrosine, creating a phosphotyrosine docking site for members of the Src family of cytoplasmic tyrosine kinases. These kinases then phosphorylate FAK on additional tyrosines, creating docking sites for a variety of intracellular signaling proteins; they also phosphorylate other proteins at focal adhesions. In this way, the signal is relayed into the cell (as discussed in Chapter 15).
One way to analyze the function of FAK is to examine focal adhesions in cells from mutant mice that lack the protein. FAK-deficient fibroblasts still adhere to fibronectin and form focal adhesions. Suprisingly, they form too many focal adhesions rather than too few; as a result, cell spreading and migration are slowed (Figure 19-66). This unexpected finding suggests that FAK normally helps disassemble focal adhesions and that this loss of adhesions is required for normal cell migration. By interacting with both conventional signaling receptors and focal adhesions, FAK can couple migratory signals to changes in cell adhesion. Many cancer cells have elevated levels of FAK, which may help explain why they are often more motile than their normal counterparts.

Figure 19-66
Excessive numbers of focal adhesions in FAK-deficient fibroblasts. Normal and FAK-deficient fibroblasts were stained with antibodies against vinculin to reveal the location of focal adhesions (see Figure 19-12). (A) The normal fibroblasts have fewer focal (more...)
Integrins and conventional signaling receptors can work together in several ways. The signaling pathways activated by conventional signaling receptors can increase the expression of integrins or extracellular matrix molecules, while those activated by integrins can increase the expression of conventional signaling receptors or the ligands that bind to them. The intracellular signaling pathways themselves can also interact and reinforce one another. While some conventional signaling receptors and integrins activate the Ras/MAP kinase pathway (see Figure 15-56) independently, for example, they often act together to sustain the activation of this pathway long enough to induce cell proliferation. Integrins and conventional signaling receptors cooperate to stimulate many types of cell response. Many cells in culture, for example, will not grow or proliferate in response to extracellular growth factors unless the cells are attached via integrins to extracellular matrix molecules. For some cell types, including epithelial, endothelial, and muscle cells, even cell survival depends on signaling through integrins. When these cells lose contact with the extracellular matrix, they undergo programmed cell death, or apoptosis. This dependence on attachment to the extracellular matrix for survival and proliferation may help ensure that the cells survive and proliferate only when they are in their appropriate location, which may protect animals against the spread of cancer cells. Attachment-dependent cell survival is exploited for special purposes in embryonic development, as shown in Figure 19-67. The signaling pathways that integrins activate to promote cell survival are similar to those activated by conventional signaling receptors, as discussed in Chapters 15 and 17.

Figure 19-67
Matrix-dependent cell survival in the formation of the proamniotic cavity. Endoderm cells are thought to produce a signal (indicated by the red arrows) that causes ectoderm cells to die by apoptosis. The ectoderm cells in contact with the basal lamina, (more...)
The cell adhesion molecules discussed in this chapter are summarized in Table 19-7.
Table 19-7
Cell Adhesion Molecule Families.
Summary
Integrins are the principal receptors used by animal cells to bind to the extracellular matrix. They are heterodimers and function as transmembrane linkers between the extracellular matrix and the actin cytoskeleton. A cell can regulate the adhesive activity of its integrins from within. Integrins also function as signal transducers, activating various intracellular signaling pathways when activated by matrix binding. Integrins and conventional signaling receptors often cooperate to promote cell growth, cell survival, and cell proliferation.
- Integrins - Molecular Biology of the CellIntegrins - Molecular Biology of the Cell
- centisome 49 region of E.coli K12 BHB2600centisome 49 region of E.coli K12 BHB2600gi|453985|gb|U00008.1|ECOHU49Nucleotide
- PCAT1 prostate cancer associated transcript 1 [Homo sapiens]PCAT1 prostate cancer associated transcript 1 [Homo sapiens]Gene ID:100750225Gene
- MIR496 microRNA 496 [Homo sapiens]MIR496 microRNA 496 [Homo sapiens]Gene ID:574454Gene
- Mir125b-1 microRNA 125b-1 [Mus musculus]Mir125b-1 microRNA 125b-1 [Mus musculus]Gene ID:387236Gene
Your browsing activity is empty.
Activity recording is turned off.
See more...