NCBI Bookshelf. A service of the National Library of Medicine, National Institutes of Health.
Institute of Medicine (US) Committee on Military Nutrition Research; Marriott BM, Carlson SJ, editors. Nutritional Needs In Cold And In High-Altitude Environments: Applications for Military Personnel in Field Operations. Washington (DC): National Academies Press (US); 1996.

Nutritional Needs In Cold And In High-Altitude Environments: Applications for Military Personnel in Field Operations.
Show detailsAndrew J. Young 1 Michael N. Sawka and Kent B. Pandolf
INTRODUCTION
Understanding and ameliorating the effects of cold is an important military concern. Throughout history, there are many examples of the terrible effects experienced by soldiers during military operations conducted during cold weather. Over 90,000 U.S. Army and Army Air Force casualties during World War II were attributable to cold injury. German Army cold-injury casualties were at least as high. Another 10,000 casualties resulting from cold injury occurred during the Korean War. Currently, cold injury prevention is an area of major command emphasis for Army units operating in cold climates.
Humans tend to rely on behavioral thermoregulation to protect themselves against the cold. That is, they wear clothing, remain in shelters, and use various heat-generating devices. However, when behavioral strategies are inadequate to defend body temperature homeostasis, physiological responses are elicited. Besides protecting against cold effects and playing a role in the etiology of cold injuries, these physiological responses may alter the metabolism of persons living and working in cold climates. This chapter reviews the human physiological responses elicited by cold exposure and then considers some factors accounting for differences in response among individuals. The purpose is to provide a basis for considering how physiological responses influence performance and nutritional requirements of soldiers exposed to cold.
HUMAN HEAT BALANCE IN THE COLD
Biophysical Factors
Body temperature reflects the summated effects of internal heart production and heat transfers between the body and ambient environment. The heat balance equation describes the relationship:

where M represents metabolic heat production, and Wk represents energy leaving (positive for concentric work) or entering (negative for eccentric work) the body as external work.2 Heat exchange between the body and environment occurs via evaporation (E), radiation (R), convection (C), and conduction (K), with W/m2 being watts per square meter. The sum of these processes is heat storage (S), which represents heat gain by the body if positive or heat loss from the body if negative. The biophysics of human thermal balance is considered in detail elsewhere (Santee and Gonzalez, 1988).
In humans exposed to environments colder than body temperature, heat flows from the body core toward the environment, primarily via dry (i.e., conductive and convective) heat-loss mechanisms. Wind increases convective heat loss from the body surface (Santee and Gonzalez, 1988), thus providing the basis for the concept of wind chill (Siple and Passel, 1945). Because water has a much higher thermal capacity than air, convective heat transfer is greater (perhaps 70-fold) during immersion in water than in air of the same temperature (Gonzalez, 1988). Clothing provides insulation between the body and the environment, thus limiting convective and conductive heat loss, but wet clothing provides considerably less insulation than dry. Thus, environmental characteristics besides temperature influence the potential for heat loss and the resulting physiological strain of defending body temperature.
Physiological Responses
Humans have two general types of responses to cold. Vasomotor responses reduce dry heat loss to the environment. Metabolic responses act to replace heat lost to the environment.
Vasomotor Responses
Peripheral vasoconstriction is one important physiological response exhibited by humans exposed to cold. Blood flow decreases as water temperature becomes colder, as shown in Figure 7-1, which depicts blood flow in the hand decreasing in response to immersion in water of decreasing temperature. During whole-body cold exposure, the vasoconstrictor response is not limited to the hands, but is widespread throughout the peripheral shell. The decrease in peripheral blood flow reduces convective heat transfer between the body's core and shell (skin, subcutaneous fat, and skeletal muscle) and increases insulation. Heat is lost from the body surface faster than it is replaced. As a result, whole-body cold exposure causes skin temperature over the entire body surface to decline (Figure 7-2). Insulation begins to increase when skin temperature falls below about 95°F (35°C), and becomes maximal when skin temperature is about 89°F (31°C) or less (Veicsteinas et al., 1982). Thus, during cold exposure, central core temperature defense occurs at the expense of a decline in skin temperature.

FIGURE 7-1
Steady-state blood flow (mean ± SE) to the hand of male Inuit and caucasian control subjects during immersion of the hand in water of various temperatures. SOURCE: Adapted from data of Brown and Page (1952).
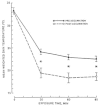
FIGURE 7-2
Mean weighted skin temperature before and during a 90-min resting cold-air exposure. Values are means ± SE of measurements in seven young caucasian men. *, Significant (P 8 0.01) difference between pre- and postacclimation. SOURCE: Young et al. (more...)
The reduction in blood flow and consequent fall in skin temperature contribute to the etiology of cold injuries (Purdue and Hunt, 1986). The hands and fingers are particularly susceptible to cold injury (Boswick et al., 1979) and to a loss of manual dexterity due to cold-induced vasoconstriction (Gaydos, 1958). In these areas of the body, another vasomotor response to cold, cold-induced vasodilation, modulates the effects of vasoconstriction. Figure 7-3 illustrates this response, first described by Lewis (1930), who termed the response the hunting reaction. Periodic oscillations (rise and fall) of skin temperature follow the initial decline in skin temperature during prolonged cold exposure. These skin temperature oscillations are the result of transient increases in blood flow to the cooled finger. Originally thought to be a local effect of cooling (Burton and Edholm, 1955), recent evidence suggests the hunting reaction may involve a centrally-mediated mechanism (Lindblad et al., 1990). A similar cold-induced vasodilation occurs in the forearm (Clarke et al., 1957; Ducharme et al., 1991). This effect may reflect the operation of a different physiological mechanism, since the forearm response appears to be the result of vasodilation in muscle vasculature rather than in skin (Ducharme et al., 1991).

FIGURE 7-3
Finger skin temperature measurements from young and older men immersing their hands in 39°F (4°C) water. SOURCE: Adapted from Mathew et al. (1986).
Metabolic Responses
In addition to those mechanisms that limit heat loss, humans employ other means to defend body temperature. Metabolic heat production can increase in order to replace heat lost during cold exposure. Muscle is generally considered the source of the increased metabolic heat production. Besides generating external force, muscle contractions also result in the liberation of considerable heat (approximately 70 percent of total energy expended). Thus, voluntary physical activity during work or exercise increases metabolic heat production (exercise in the cold will be considered later in the chapter). In the absence of an increase in voluntary muscle activity, shivering begins. Certain animals respond to cold exposure with an increase in metabolic heat production by noncontracting tissue, a process referred to as nonshivering thermogenesis (LeBlanc et al., 1967). However, there is no clear evidence that humans share this mechanism (Toner and McArdle, 1988).
Shivering is an involuntary pattern of repetitive, rhythmic muscle contractions. Horvath (1981) referred to shivering as a ''quasiexercising" state, since the muscles contract but do no external work. Shivering may begin immediately or within several minutes after the onset of cold exposure, usually in torso muscles, followed by a spread to the limbs (Horvath, 1981). The electromyographic measurement in individual shivering muscles can be analyzed to quantify shivering activity (Muza et al., 1986). More commonly, however, shivering thermogenesis is quantified by measuring the increase in whole-body oxygen uptake (o2). By assuming that the respiratory exchange ratio represents a nonprotein respiratory quotient, calculation of the thermal equivalent (i.e., metabolic heat production) of the
o2 is possible (McArdle et al., 1991).
The increased o2 associated with the onset of shivering in the cold requires an increased systemic oxygen transport. Cardiac output increases with cold exposure. Figure 7-4 depicts this increase in terms of heart rate, stroke volume, and cardiac output for men resting in thermoneutral and cold air. The cardiac output increases primarily because of an increase in stroke volume, with little change in resting heart rate during cold exposure (Muza et al., 1988).
![FIGURE 7-4. Resting heart rate, stroke volume, and cardiac output (mean ± SE) of seven young caucasian men before and during 90-min exposure to cold air (41°F [5°C]).](/books/NBK232852/bin/img00006.gif)
FIGURE 7-4
Resting heart rate, stroke volume, and cardiac output (mean ± SE) of seven young caucasian men before and during 90-min exposure to cold air (41°F [5°C]). SOURCE: Muza et al. (1988), used with permission.
As shivering intensity increases and more muscles become involved, the o2 increases. For example,
o2 of young men resting in 41°F (5°C) air with a 1 m/s wind averaged 600 to 700 ml/min, which corresponded to about 15 percent of their
o2 max (Young et al., 1986). Immersion in cold water can elicit even more intense shivering, as reflected by higher
o2. Inactive men immersed in 64°F (18°C) water exhibited
o2 of about 1 liter/min, which corresponded to 25 to 30 percent of their
o2 max (Young et al., 1989). Investigators have attempted to define maximal shivering capacity in terms of
o2. Iampietro et al. (1960) observed
o2 to be about 1,500 ml/min in inactive men exposed nude to -1°F (-18°C) with a 4.5 m/s wind. The highest reported
o2 during shivering in cold water is 2.2 liter/min in 54°F (12°C) water (Golden et al., 1979) corresponding to 46 percent
o2 max. Thus, shivering intensity varies with the severity of cold stress.
Energy Substrate Utilization
Shivering, like all muscular activity, depends on an adequate supply of substrate for the metabolic processes producing energy for the contractions. Metabolic rate can increase two- to fivefold (Horvath, 1981; Toner and McArdle, 1988; Young, 1990), depending on intensity of shivering, as discussed above. This increase has nutritional implications for persons who live and work in cold conditions. Persons adequately clothed or sheltered from the environment do not shiver much, and thus nutritional requirements are not significantly affected. Those who are not adequately protected from the cold by clothing and shelter will shiver, and their nutritional energy requirements will be greater than in warmer climates. While it is obvious that the increment in nutritional energy requirement will be proportional to the duration and severity of cold exposure, accurate predictions of individual requirements are difficult.
Attempts have been made to determine whether the increased metabolic rate of shivering muscle causes preferential use of a particular substrate. Vallerand and Jacobs (1989) used indirect calorimetry to quantify the relative contribution of carbohydrate and fat metabolism to the total energy requirements of inactive men shivering for 2 hours in cold air. In that study, shivering metabolism increased to about 2.5 times the resting metabolic rate measured in thermoneutral conditions (Vallerand and Jacobs, 1989). The increased metabolism caused almost a sevenfold increase (588 percent) in carbohydrate oxidation while fat oxidation rose less than twofold (63 percent) compared to resting in thermoneutral conditions (Vallerand and Jacobs, 1989). Further, carbohydrate and fat oxidation provided 18 percent and 59 percent respectively of the total energy expenditure in the neutral condition compared to 51 percent and 39 percent in the cold condition (Vallerand and Jacobs, 1989). These findings indicate that both fat and carbohydrate metabolism sustain shivering, but that carbohydrate is the dominant energy source.
Either blood glucose, muscle glycogen stores, or both may provide the source of carbohydrate for shivering thermogenesis. The importance of maintaining adequate blood glucose concentrations to sustain shivering activity is clear. Young men exposed to cold air stopped shivering, and their metabolic rate and core temperature declined when blood glucose concentration dropped below 2.5 mmol/liter (Gale et al., 1981). However, intravenous glucose infusion restored shivering in both an arterially occluded and an unoccluded leg (Gale et al., 1981). This finding suggests that glucose exerts a centrally-mediated effect on shivering; however, a role for blood glucose as a substrate for shivering muscle is not precluded, particularly since cold exposure enhances insulin-stimulated glucose uptake in peripheral tissues (Vallerand et al., 1988).
The importance of muscle glycogen for shivering thermogenesis remains controversial. Young et al. (1989) attempted to determine whether shivering depletes muscle glycogen stores and whether muscle glycogen depletion limits shivering or compromises thermoregulation in the cold. Changes in muscle glycogen concentration and core temperature were measured in eight young men during 1 to 3 hours of immersion in 64°F (18°C) water preceded either by 3 days of heavy exercise and a low-carbohydrate diet or by 3 days of rest and a high-carbohydrate diet. The exercise and low carbohydrate diet resulted in very low preimmersion muscle glycogen levels, while rest and a high-carbohydrate diet produced very high glycogen levels; blood glucose concentrations were not significantly different between trials. Despite different preimmersion muscle glycogen levels, there were no significant differences in metabolic rate or in the fall in core temperature during immersion (Young et al., 1989). As shown in Figure 7-5, no significant change in muscle glycogen levels occurred during either trial immersion (Young et al., 1989). This result suggests that shivering does not deplete muscle glycogen, perhaps because of availability of blood glucose. Furthermore, muscle glycogen depletion does not compromise metabolic heat production or core temperature defense during cold exposure.

FIGURE 7-5
Effect of 1- to 3-h immersion in 65°F (18°C) water on muscle glycogen concentration. Dashed line represents line of identity (no change). Individual data from trials in which initial glycogen levels were high (triangles) or low (circles) (more...)
Using a similar experimental design, also using eight young male subjects, but with shorter immersions, Martineau and Jacobs (1989) arrived at a different conclusion. Figure 7-5 compares their data with the findings of Young et al. (1989). Martineau and Jacobs (1989) reported that muscle glycogen levels decreased during a high-glycogen immersion trial but not during a low-glycogen trial. Initial metabolic rate was significantly lower in the low muscle glycogen trial, although eventually it achieved the level of the high-glycogen trial. Furthermore, body temperature declined slightly faster during the low-glycogen trial (34.5°F/h [1.5°C/h]) than during the high-glycogen trial (34.25°F [1.25°C/h]) (Martineau and Jacobs, 1989). Martineau and Jacobs (1989) concluded that muscle glycogen served as a substrate during shivering and that muscle glycogen depletion impaired thermoregulation in the cold.
The discrepancies between the findings of the two studies are not readily explained. The subjects studied by Martineau and Jacobs (1989) were extremely lean compared to those studied by Young et al. (1989). However, the leaner subjects did not shiver more intensely than the fatter subjects. In both studies, metabolic rates were similar, corresponding to about 25 to 30 percent o2 max. Steady-state exercise at such a low intensity would not deplete muscle glycogen. Furthermore, the fatter subjects in the study of Young et al. (1989) were immersed and shivered longer (2 to 3 hours versus 1 hour), yet they did not exhibit muscle glycogen depletion. Lastly, the changes in muscle glycogen that Martineau and Jacobs (1989) observed during immersion (see Figure 7-5), and the effect of low muscle glycogen on body cooling were small. Thus, muscle glycogen is probably not an obligatory substrate for shivering, at least at sea level. When alternate substrates, such as blood glucose, are available, muscle glycogen can be spared or resynthesized at a rate equal to its use.
At high altitudes, muscle glycogen may be an important substrate for sustaining shivering in the cold. Ascent to high altitude decreases o2 max. A
o2 corresponding to 25 to 30 percent of
o2 max at sea level would require 60 to 70 percent
o2 max at 5,000 m. Exercise at that intensity would significantly deplete muscle glycogen, and muscle glycogenolysis during exercise is faster at high altitude than at sea level (Young, 1990). Whether altitude affects muscle glycogenolysis the same during shivering as during exercise remains to be determined experimentally.
Effects of Exercise on Thermoregulation in the Cold
Voluntary physical activity can increase metabolic heat production more than shivering. Whereas maximal shivering can elevate o2 to about 2 liter/min, exercise can increase
o2 to 5 liter/min or even higher. However, the effect of exercise on thermal balance depends on a complex interaction among factors related to exercise intensity, environmental conditions, and mode of activity. While exercise increases metabolic heat production, it also facilitates heat loss from the body by increasing blood flow to the skin and active muscles. This flow enhances convective heat transfer from the central core to peripheral shell. Thus, while metabolic heat production increases progressively as exercise intensity increases, so too does heat loss due to increasing blood flow to muscle and skin. Also, limb movement increases convective heat loss from the body surface by disrupting the stationary boundary layer of air or water that develops at the skin surface in a still environment.
The arms have a greater surface area-to-mass ratio and a thinner subcutaneous fat layer than the legs (Toner and McArdle, 1988). Thus the increased blood flow to the muscles and skin of the arms resulting from upper body exercise has a greater effect on convective heat transfer than does that which results from lower body exercise. In fact, Toner et al. (1984) observed that heat loss is more pronounced and core temperature falls more during arm exercise than during leg exercise at the same absolute metabolic rate. These effects are magnified by the greater convective heat transfer coefficient of water as compared to air. In cold air, metabolic heat production during exercise can be high enough to compensate for increased heat loss and allow core temperature to be maintained even when ambient temperature is extremely cold (Toner and McArdle, 1988). In contrast, increased heat loss during exercise in cold water can be so great that metabolic heat production, even during intense exercise, is insufficient to defend core temperature (Toner and McArdle, 1988).
During submaximal exercise in the cold, o2 can be higher than, or the same as in temperate conditions, depending on the exercise intensity (Young, 1990). Figure 7-6 schematically depicts the effect of cold exposure on
o2 during exercise over a range of submaximal intensities. At low intensities,
o2 is higher in cold than in temperate conditions, since metabolic heat production during low-intensity exercise is insufficient to maintain core and skin temperatures high enough to prevent the afferent stimulus for shivering. Thus, the increased
o2 represents the added oxygen requirement for shivering activity. As metabolic heat production rises with increasing exercise intensity, the afferent stimulus for shivering declines, and at some point, exercise metabolism is high enough to prevent shivering completely. At this intensity and higher,
o2 during exercise is the same in cold and temperate conditions. The exercise intensity at which metabolic heat production is sufficient to prevent shivering will depend on the severity of cold stress. Furthermore, that intensity will not necessarily be the same for all persons exposed to the same cold stress, because of individual characteristics that will be discussed later.

FIGURE 7-6
Effect of cold on o2 during steady-state exercise at different intensities.
Cold exposure can reduce maximal oxygen uptake, but may not always do so (Young, 1990). Exposure conditions must be severe enough to reduce core or muscle temperature markedly (> 0.5°C [> 0.9°F]) before Vo2max is reduced (Bergh and Ekblom, 1979; Fortney and Senay, 1979; Horvath, 1981; McArdle et al., 1976). Exposure to (cold) conditions that lower core temperature 0.5°C (0.9°F) or less does not significantly reduce Vo2max (Schmidt and Bruck, 1981). Potential mechanisms explaining how cold exposure could reduce Vo2max include that a low body temperature may impair myocardial contractility (Bergh and Ekblom, 1979) and limit maximal heart rate (Bergh and Ekblom, 1979; Fortney and Senay, 1979; Horvath, 1981; McArdle et al., 1976) sufficiently to limit maximal cardiac output.
Cardiovascular Responses to Exercise in the Cold
Cold exposure can also affect cardiovascular responses to submaximal exercise. Obviously, cardiac output must increase to satisfy the requirement for increased systemic oxygen transport when cold exposure stimulates shivering during low-intensity exercise in the cold. For a given o2, cardiac output is the same during exercise in cold and temperate conditions (McArdle et al., 1976). However, cold exposure can alter the way that cardiac output is achieved. Heart rate is usually lower and stroke volume higher during exercise in cold air or cold water compared to exercise at the same
o2 in temperate conditions (Doubt, 1991; McArdle et al., 1976). This phenomenon probably reflects the effect of increased cardiac preload due to the increased central blood volume that is associated with cold-induced peripheral vasoconstriction.
Influence of Cold on Muscle Energy Metabolism
Cold exposure may affect muscle energy metabolism during exercise. Some, but not all, investigators have observed an increase in blood lactate concentration during exercise in cold over that observed in temperate conditions (Young, 1990). When core temperature and o2 are similar during exercise in cold and temperate conditions, blood lactate is unaffected by cold. Studies in which cold exposure increased blood lactate concentrations during exercise also recorded lower core temperatures and higher
o2 during exercise in cold than in temperate conditions (Young, 1990). Furthermore, animal experiments employing radioactively labeled lactate infusions to measure lactate turnover rates during exercise show that cold exposure can increase both the appearance and removal of blood lactate compared to neutral conditions with no net increase in concentration (Minaire et al., 1971).
The effects of cold exposure on lactate metabolism during exercise raise the possibility that cold exposure may accelerate muscle glycolysis during exercise. During steady-state exercise at higher intensities, muscle glycogen utilization is the same in cold and temperate conditions (Jacobs et al., 1985; Young et al., 1995). However, during low-intensity steady-state exercise, glycogen use in the active muscle is more pronounced in cold than in temperate conditions (Jacobs et al., 1985). The increased glycogen use during low-intensity exercise has been attributed to the added metabolic cost of shivering, but in fact o2 was the same during exercise in cold and temperate conditions, which suggests that shivering may not explain the increased use of glycogen (Jacobs et al., 1985). Blomstrand and Essen-Gustavson (1987) and Blomstrand et al. (1986) demonstrated that dramatically lowering muscle temperature (to about 82°F [28°C]) accelerates muscle glycolysis during short, very intense exercise. No clear experimental explanation for that observation is available, but decreased muscle temperature may reduce mechanical or biochemical efficiency of the muscle (Young, 1990). Whatever the mechanism, it seems that reduced muscle and core temperatures, rather than cold exposure, are responsible for alterations in muscle energy metabolism during exercise.
INDIVIDUAL CHARACTERISTICS MODIFYING HUMAN HEAT BALANCE IN THE COLD
Anthropometry
Differences in body size, configuration, and composition can explain much of the variability between individuals in their capability to defend body temperature during cold exposure (Toner and McArdle, 1988). These body characteristics modify the stress of a given environmental condition. Therefore, different persons exposed to the same environment do not experience the same stress or exhibit responses of the same magnitude. Effects attributed to acclimatization, aging, gender, and physical fitness on thermoregulatory response to cold are in large part due to differences in anthropometric factors that co-vary with those other factors (LeBlanc et al., 1978; Young, 1991; Young et al., 1995).
Body shape and mass contribute significantly to an individual's tendency to lose heat in cold environments. Because the principal heat loss vector in humans exposed to cold is convective heat transfer at the skin surface, a large surface area favors greater heat loss than a smaller surface area. In contrast, a large body mass favors maintenance of a constant temperature by virtue of a greater heat content when compared to a small body mass. Gonzalez (1988) explains the biophysical basis for the interaction between the two factors in detail elsewhere. Here, it suffices to point out that it is the ratio of surface area to body mass that influences heat loss. All other factors being equal (which is rarely the case), persons with a large surface area-to-mass ratio experience greater declines in body temperature during cold exposure than those with smaller surface area-to-mass ratios (Burton and Edholm, 1955; Toner and McArdle, 1988). This concept also applies when considering regional heat loss patterns. Thus, as discussed earlier, Toner et al. (1984) observed that heat loss and the decline in body temperature in cold water were greater during arm than during leg exercise at the same metabolic rate because of the greater surface area-to-mass ratio of the arms.
Body fat is one of the most important characteristics modifying the stress of cold exposure. All body tissues provide thermal resistance to heat conduction from within the body, but thermal resistivity of fat is greater than that of either skin or muscle (Toner and McArdle, 1988). Subcutaneous fat provides significant insulation against heat loss in the cold. Figure 7-7 depicts whole-body heat loss measured in young male Inuits (Native Americans residing in the Arctic) and caucasians residing in temperate regions of North America. Heat loss was measured under conditions in which peripheral blood flow was minimal (immersion in water cool enough to induce maximal vasoconstriction without eliciting shivering). These conditions minimize heat convection so that heat flow reflects thermal conductance reasonably accurately. As shown, thermal conductance—or its inverse relationship, insulation—is closely correlated with subcutaneous fat thickness. Thus, thermal conductance decreases and insulation increases as the layer of subcutaneous fat thickens. As a result, as many studies have confirmed, fat persons shiver less and experience smaller declines in body temperature during cold exposure than do lean persons (Toner and McArdle, 1988). Nutritional strategies during cold weather should aim to prevent body fat loss in soldiers, especially during long-duration operations.
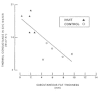
FIGURE 7-7
Relationship between subcutaneous body fat and thermal conductance measured under conditions that elicited maximal peripheral vasoconstriction without causing shivering or increased metabolism. Under these conditions, convective heat flux is minimized, (more...)
Physical Fitness
There is no consensus concerning the influence of physical fitness, particularly aerobic capacity, on thermoregulatory response to cold. Some investigations have employed a cross-sectional experimental design to evaluate aerobic fitness effects on responses to cold. Bittel et al. (1988) reported that fit persons maintained warmer skin temperatures than did less fit persons during rest in cold air. However, the effect appeared to be due to thinner subcutaneous fat thickness and higher metabolic heat production in fit compared to less fit subjects, rather than to a fitness effect, per se, on vasoconstriction (Bittel et al., 1988). Budd et al. (1991) found no relationship between o2 max and skin temperature during rest in cold air but conceded that their subjects'
o2 max encompassed a range too narrow to evaluate fitness effects effectively.
Endurance training effects are not addressed well by cross-sectional studies since factors in addition to training contribute to a high o2 max. Longitudinal studies indicate that endurance training strengthens cutaneous vasoconstrictor response to cold. Young et al. (1995) reported that after 8 weeks of endurance training, which increased
o2 max by 13 percent, subjects exhibited a faster decline in skin temperature during exercise in cold water than before training. Similarly, Kollias and Buskirk (1972), reported that after 9 weeks of aerobic training, there was a faster decline in skin temperature during resting exposure to cold air than before training. Therefore, endurance training provides a thermoregulatory advantage for persons exposed to cold.
Age and Gender
Aging is widely though to compromise body temperature defense during cold exposure. A recent review of the relevant scientific literature (Young, 1991), however, suggests that this belief may not be entirely justified. The incidence of hypothermia on admission to hospitals appears greater for older (60 years or more) than for younger persons (LeBlanc et al., 1978). However, the overall incidence of hypothermia admission is low compared to other ailments resulting in hospital admission, and coexisting conditions such as injury, illness, and alcohol or drug intoxication may confound these data (Coleshaw et al., 1986; Keatinge, 1986). Epidemiological surveys of body temperature of older persons taken while in their own homes do not indicate a large incidence of hypothermia (Collins et al., 1977; Fox et al., 1973). Nevertheless, controlled laboratory comparisons show that older men may be less able than younger men to defend core temperature during cold exposures. The cutaneous vasoconstrictor response to cold may be slower and cold-induced vasodilation may be blunted (see Figure 7-3) in older as compared to younger men (Mathew et al., 1986). Shivering thermogenesis may also be less in older than younger men (Young, 1991). The latter effect is probably the result of a loss of muscle mass, rather than an effect of aging on thermoregulation (Mathew et al., 1986). These aging effects begin to be apparent after about 45 years of age in men (Young, 1991). Data from one study, however, indicated that older women defend core temperature during cold exposure as well as, or better than, younger women (Wagner and Horvath, 1985). Here again, body composition changes with aging (the older women were much fatter than the younger women) probably accounted for the difference attributed to aging. It is possible that preventable changes in body composition and physical fitness rather than aging may account for impaired (as well as improved) thermoregulatory responses to cold.
Gender-related differences in body size, body shape and composition, and hormonal effects associated with the menstrual cycle affect heat balance and thermoregulatory response to cold (Stephenson and Kolka, 1993). These differences contribute to a disparity in cold tolerance between men and women, which is particularly apparent in cold water. Most women have greater fat content and subcutaneous fat thickness than men of comparable age. A thicker subcutaneous fat layer accounts for the greater maximal tissue insulation and lower critical water temperature (coldest water tolerated without shivering) observed in women as compared with men (Rennie et al., 1962a). Despite this difference, however, greater fat content may not provide women with a thermoregulatory advantage over men.
When women and men of equivalent subcutaneous fat thickness are compared, the women have a greater surface area and smaller total body mass. Although insulation is equivalent, women's total heat loss is greater due to the larger surface area for convective heat flux. Because of their smaller body mass, body heat content is less in the women. Therefore body temperature falls more rapidly for any given thermal gradient and metabolic rate. The mathematical basis for this concept is explained elsewhere (Gonzalez, 1988). The findings of McArdle et al. (1984a, b) provide experimental demonstration of the concept. They reported that women's core temperatures fall more rapidly during cold-water immersion with resting than those of men with equal subcutaneous fat thickness (McArdle et al., 1984a). When men and women of equivalent subcutaneous fat thickness exercised in cold water at the same metabolic rate per unit surface area, both experienced similar core temperature changes (McArdle et al., 1984b).
Comparison of men and women with equivalent total body masses shows that women still seem to be at a disadvantage in the cold. In this case, women's greater fat content enhances insulation, and surface area differences between the genders are not as pronounced. Nevertheless, a smaller lean body mass, the source of metabolic heat production, limits women's capacity for heat production, compared to men of comparable total body mass. This disparity may be inconsequential under conditions where metabolism is low and does not differ much between men and women (i.e., when resting in mildly cool conditions such as those used to assess maximal tissue insulation) (Rennie et al., 1962a). However, under colder conditions that stimulate shivering—especially maximal shivering—the limited thermogenic capacity of women will result in a more rapid decline in their core temperature than in men of equivalent total body mass.
Acclimatization
Persons chronically exposed to cold experience adjustments in thermoregulation (Young, 1988). Habituation is, by far, the most commonly observed adjustment to chronic cold exposure. Blunting of both shivering and cold-induced vasoconstriction are the hallmarks of habituation (Young, 1988). These adjustments enable skin to be kept warmer during cold exposure, but they can contribute to a greater heat loss and more pronounced fall in core temperature.
Besides habituation, cold acclimatization and cold acclimation can heighten responses to cold or induce responses not apparent in the unacclimatized state. These adjustments follow two patterns. First, metabolic acclimatization-acclimation is characterized by a more pronounced thermogenic response to cold (Young, 1988). An exaggerated shivering response may develop because of chronic cold exposure, and the possibility that humans develop a nonshivering thermogenesis cannot be completely ruled out. In contrast, enhanced heat conservation mechanisms characterize the insulative acclimatization-acclimation pattern (Young, 1988). More rapid cutaneous vasoconstriction develops in some chronically cold-exposed persons, an adjustment that may reflect an enhanced sympathetic nervous response (Young, 1988).
Compared to chronic heat stress, physiological adjustments to chronic cold exposure appear less practical in terms of relieving thermal strain, defending body temperature, and preventing thermal illness and injury. Nonetheless, changes in shivering response to cold resulting from habituation or metabolic acclimatization may have some nutritional implications.
AUTHORS' CONCLUSIONS AND RECOMMENDATIONS
Humans exhibit two major physiological responses to cold exposure. Peripheral vasoconstriction limits heat loss. Shivering, physical activity, or both increase heat production. Thus, heat balance in the cold and the requirement for shivering are dependent on the severity of environmental stress and the effectiveness of the vasoconstriction for conserving heat, as well as the intensity and mode of activity or exercise. There are nutritional implications of the physiological responses, particularly the thermogenic response. Increasing metabolic heat production requires increased energy intake. Carbohydrate metabolism may contribute more to total energy metabolism in cold than in temperate environments. Gender, aging, and acclimatization all affect thermoregulatory responses to cold, but these effects probably have little nutritional significance. Body composition is probably the most important physiological determinant of thermoregulatory tolerance in cold environments. Behavioral responses, such as taking shelter from the cold and wearing adequate protective clothing, can greatly reduce the physiological strain of cold exposure and obviate the need for nutritional interventions.
Some general recommendations can be made:
- Research should resolve discrepant findings concerning effects of muscle glycogen depletion on thermogenesis and heat balance in the cold, with emphasis on effects of body fat differences.
- The effects of hypoxia on cold-induced thermogenesis and substrate utilization should be studied.
- Novel techniques for stimulating thermogenesis should be developed, particularly for emergency or rescue situations in cold weather.
- The possibility that physiological responses to an acute cold challenge might be used reliably to predict susceptibility to cold injury should be studied.
- The possibility that age- and gender-related differences in heat balance and thermoregulatory responses to cold can be minimized by physical training and nutritional strategies should be investigated.
REFERENCES
- Bergh, U., and B. Ekblom 1979. Physical performance and peak aerobic power at different body temperatures. J. Appl. Physiol. 46:885–889. [PubMed: 468604]
- Bittel, J.H.M., C. Nonott-Varly, G.H. Livecchi-Gonnot, G.L.M.J. Savourey, and A.M. Hanniquet 1988. Physical fitness and thermoregulatory reactions in a cold environment in men. J. Appl. Physiol. 65:1984–1989. [PubMed: 3209545]
- Blomstrand, E., and B. Essen-Gustavson 1987. Influence of reduced muscle temperature on metabolism in type I and type II human muscle fibres during intensive exercise. Acta Physiol. Scand. 131:569–574. [PubMed: 3442242]
- Blomstrand, E., L. Kaijser, A. Martinsson, U. Bergh, and B. Ekblom 1986. Temperature-induced changes in metabolic and hormonal responses to intensive dynamic exercise. Acta Physiol. Scand. 127:477–484. [PubMed: 3751634]
- Boswick, J.A., J.D. Thompson, and R.A. Jonas 1979. The epidemiology of cold injuries. Surg. Gynecol. Obstet. 149:326–332. [PubMed: 472990]
- Brown, G.M., and J. Page 1952. The effect of chronic exposure to cold on temperature and blood flow of the hand. J. Appl. Physiol. 5:220–227. [PubMed: 12999657]
- Budd, G.M., J.R. Brotherhood, A.L. Hendrie, and S.E. Jeffery 1991. Effects of fitness, fatness, and age on men's responses to whole body cooling in air. J. Appl. Physiol. 71:2387–2393. [PubMed: 1778937]
- Burton, A.C., and O.G. Edholm 1955. Vascular reactions to cold. Pp. 129–147 in Man in a Cold Environment, L.E. Bayliss, editor; , W. Feldberg, editor; , and A.L. Hodgkin, editor. , eds. London: Edward Arnold Publishers, Ltd.
- Clarke, R.S.J., F. Hellon, and A.R. Lind 1957. Cold vasodilation in the human forearm. J. Physiol. 137:84–85.
- Coleshaw, S.R.K., J.C. Easton, W.R. Keatinge, M.A. Floyer, and J. Garrard 1986. Hypothermia in emergency admissions in cold weather [abstract]. Clin. Sci. 70:93.
- Collins, K.J., C. Dore, A.N. Exton-Smith, R.H. Fox, and I.C. Macdonald 1977. Accidental hypothermia and impaired temperature homeostasis in the elderly. Br. Med. J. 1:353–356. [PMC free article: PMC1604514] [PubMed: 837095]
- Ducharme, M.B., W.P. VanHelder, and M.W. Radomski 1991. Cyclic intramuscular temperature fluctuations in the human forearm during cold-water immersion. Eur. J. Appl. Physiol. 63:188–193. [PubMed: 1761006]
- Fortney, S.M., and L.C. Senay 1979. Effect of training and heat acclimation on exercise responses of sedentary females. J. Appl. Physiol. 47:978–984. [PubMed: 511724]
- Fox, R.H., P.M. Woodward, A.N. Exton-Smith, M.F. Green, D.V. Donnison, and M.H. Wicks 1973. Body temperatures in the elderly: A national study of physiological, social, and environmental conditions. Br. Med. J. 1:200–206. [PMC free article: PMC1588152] [PubMed: 4686555]
- Gale, E.A.M., T. Bennett, J.H. Green, and I.A. Macdonald 1981. Hypoglycaemia, hypothermia and shivering in man. Clin. Sci. 61:463–469. [PubMed: 7026128]
- Gaydos, H.F. 1958. Effect on complex manual performance of cooling the body while maintaining the hands at normal temperatures. J. Appl. Physiol. 12:373–376. [PubMed: 13525295]
- Golden, F.S.C., I.F.G. Hampton, G.R. Hervery, and A.V. Knibbs 1979. Shivering intensity in humans during immersion in cold water [abstract]. J. Physiol. (Lond.) 277:48p. [PubMed: 469790]
- Gonzalez, R.R. 1988. Biophysics of heat transfer and clothing considerations. Pp. 45–95 in Human Performance Physiology and Environmental Medicine at Terrestrial Extremes, K.B. Pandolf, editor; , M.N. Sawka, editor; , and R.R. Gonzalez, editor. , eds. Indianapolis, Ind.: Benchmark Press.
- Horvath, S.M. 1981. Exercise in a cold environment. Exerc. Sports Sci. Rev. 9:221–263. [PubMed: 6749522]
- Iampietro, P.F., J.A. Vaughan, R.F. Goldman, M.B. Krieder, F. Masucci, and D.E. Bass 1960. Heat production from shivering. J. Appl. Physiol. 15:632–634. [PubMed: 14405791]
- Jacobs, I., T.T. Romet, and D. Kerrigan-Brown 1985. Muscle glycogen depletion during exercise at 9 degrees C and 21 degrees C. Eur. J. Appl. Physiol. 54:35–39. [PubMed: 4018052]
- Keatinge, W. 1986. Medical problems of cold weather. J. R. Coll. Physicians Lond. 20:283–287. [PMC free article: PMC5371043] [PubMed: 3772849]
- Kollias, R.B., and E.R. Buskirk 1972. Effects of physical condition in man on thermal responses to cold air. Int. J. Biometeorol. 16:389–402. [PubMed: 4657908]
- LeBlanc, J., D. Robinson, D.F. Sharman, and P. Tousignant 1967. Catecholamines and short-term adaptation to cold in mice. Am. J. Physiol. 213:1419–1422. [PubMed: 6075743]
- LeBlanc, J., J. Cote, S. Dulac, and F. Dulong-Turcot 1978. Effects of age, sex and physical fitness on responses to local cooling. J. Appl. Physiol. 44:813–817. [PubMed: 649481]
- Lewis, T. 1930. Observations upon the reactions of the human skin to cold. Heart 15:177–181.
- Lindblad, L.E., L. Ekenvall, and C. Klingstedt 1990. Neural regulation of vascular tone and cold induced vasoconstriction in human finger skin. J. Auton. Nerv. Syst. 30:169–174. [PubMed: 2370420]
- Martineau, L., and I. Jacobs 1989. Muscle glycogen availability and temperature regulation in humans. J. Appl. Physiol. 66:72–78. [PubMed: 2917958]
- Mathew, L., S.S. Purkayastha, R. Singh, and J.S. Gupta 1986. Influence of aging in the thermoregulatory efficiency of man. Int. J. Biometeorol. 30:137–145. [PubMed: 3721612]
- McArdle, W.D., J.R. Magel, G.R. Lesmes, and G.S. Pechar 1976. Metabolic and cardiovascular adjustment to work in air and water at 18, 25 and 33°C. J. Appl. Physiol. 40:85–90. [PubMed: 1248988]
- McArdle, W.D., J.R. Magel, T.J. Gergley, R.J. Spina, and M.M. Toner 1984. a Thermal adjustment to cold-water exposure in resting men and women. J. Appl. Physiol. 56:1565–1571. [PubMed: 6735815]
- McArdle, W.D., J.R. Magel, R.J. Spina, T.J. Gergley, and M.M. Toner 1984. b Thermal adjustment to cold-water exposure in exercising men and women. J. Appl. Physiol. 56:1572–1577. [PubMed: 6735816]
- McArdle, W.D., F.I. Katch, and V.L. Katch 1991. Measurement of human energy expenditure. Pp. 145–157 in Exercise Physiology. Philadelphia, Pa.: Lea & Febiger.
- Minaire, Y., A. Pernod, M.J. Jomain, and M. Mottaz 1971. Lactate turnover and oxidation in normal and adrenal-demedulated dogs during cold exposure. Can. J. Physiol. Pharmacol. 49:1063–1070. [PubMed: 5142311]
- Muza, S.R., A.J. Young, M.N. Sawka, K.B. Pandolf, and J.E. Bogart 1986. Power spectral analysis of the surface electromyogram during shivering. Aviat. Space Environ. Med. 57:1150–1153. [PubMed: 3800814]
- Muza, S.R., A.J. Young, M.N. Sawka, J.E. Bogart, and K.B. Pandolf 1988. Respiratory and cardiovascular responses to cold stress following repeated cold water immersion. Undersea Biomed. Res. 15:165–178. [PubMed: 3388627]
- Purdue, G.F., and J.L. Hunt 1986. Cold injury: A collective review. J. Burn Cancer Res. 7:331–341. [PubMed: 2889738]
- Rennie, D.W., B.G. Covino, M.R. Blair, and K. Rodahl 1962. a Physical regulation of temperature in Eskimos. J. Appl. Physiol. 17:326–332. [PubMed: 14491492]
- Rennie, D.W., B.G. Covino, B.J. Howell, S.H. Song, B.S. Kang, and S.K. Hong 1962. b Physical insulation of Korean diving women. J. Appl. Physiol. 17:961–966. [PubMed: 13981679]
- Santee, W.R., and R.R. Gonzalez 1988. Characteristics of the thermal environment. Pp. 1–43 in Human Performance Physiology and Environmental Medicine at Terrestrial Extremes, K.B. Pandolf, editor; , M.N. Sawka, editor; , and R.R. Gonzalez, editor. , eds. Indianapolis, Ind.: Benchmark Press.
- Schmidt, V., and K. Bruck 1981. Effect of a precooling maneuver on body temperature and exercise performance. J. Appl. Physiol. 50:772–778. [PubMed: 7263359]
- Siple, P.A., and C.R. Passel 1945. Measurements of dry atmospheric cooling in sub freezing temperatures. Proc. Am. Philos. Soc. 89:177–199.
- Stephenson, L.A., and M.A. Kolka 1993. Thermoregulation in women. Exerc. Sports Sci. Rev. 21:231–262. [PubMed: 8504843]
- Toner, M.M., and W.D. McArdle 1988. Physiological adjustments of man to the cold. Pp. 361–399 in Human Performance Physiology and Environmental Medicine at Terrestrial Extremes, K.B. Pandolf, editor; , M.N. Sawka, editor; , and R.R. Gonzalez, editor. , eds. Indianapolis, Ind.: Benchmark Press.
- Toner, M.M., M.N. Sawka, and K.B. Pandolf 1984. Thermal responses during arm and leg and combined arm-leg exercise in water. J. Appl. Physiol. 56:1355–1360. [PubMed: 6725090]
- Vallerand, A.L., and I. Jacobs 1989. Rates of energy substrates utilization during human cold exposure. Eur. J. Appl. Physiol. 58:873–878. [PubMed: 2767069]
- Vallerand, A.L., J. Frim, and M.F. Kavanagh 1988. Plasma glucose and insulin responses to oral and intravenous glucose in cold-exposed humans. J. Appl. Physiol. 65:2395–2399. [PubMed: 3063707]
- Veicsteinas, A., G. Ferretti, and D.W. Rennie 1982. Superficial shell insulation in resting and exercising men in cold water. J. Appl. Physiol. 52:1557–1564. [PubMed: 7107465]
- Wagner, J.A., and S.M. Horvath 1985. Influences of age and gender on human thermoregulatory responses to cold exposures. J. Appl. Physiol. 58:180–186. [PubMed: 3968009]
- Young, A.J. 1988. Human adaptation to cold. Pp. 401–434 in Human Performance Physiology and Environmental Medicine at Terrestrial Extremes, K.B. Pandolf, editor; , M.N. Sawka, editor; , and R.R. Gonzalez, editor. , eds. Indianapolis, Ind.: Benchmark.
- 1990. Energy substrate utilization during exercise in extreme environments. Exerc. Sports Sci. Rev. 18:65–117. [PubMed: 2192901]
- Young, A.J., S.R. Muza, M.N. Sawka, R.R. Gonzalez, and K.B. Pandolf 1986. Human thermoregulatory responses to cold air are altered by repeated cold water immersion. J. Appl. Physiol. 60:1542–1548. [PubMed: 3710973]
- Young, A.J., M.N. Sawka, P.D. Neufer, S.R. Muza, E.W. Askew, and K.B. Pandolf 1989. Thermoregulation during cold water immersion is unimpaired by low muscle glycogen levels. J. Appl. Physiol. 66:1809–1816. [PubMed: 2732173]
- Young, A.J., M.N. Sawka, L. Levine, P.W. Burgoon, W.A. Latzka, R.R. Gonzalez, and K.B. Pandolf 1995. Metabolic and thermal adaptations form endurance training in hot or cold water. J. Appl. Physiol. 78:793–901. [PubMed: 7775320]
Footnotes
- 1
Andrew J. Young, Thermal Physiology and Medicine Division, Environmental Physiology and Medicine Directorate, U.S. Army Research Institute of Environmental Medicine, Natick, MA 01760-5007
- 2
During concentric work, the muscle shortens as it develops tension; during eccentric work, the muscle lengthens as it develops tension.
- Physiology of Cold Exposure - Nutritional Needs In Cold And In High-Altitude Env...Physiology of Cold Exposure - Nutritional Needs In Cold And In High-Altitude Environments
- H1f11-ps H1.11 linker histone, pseudogene [Mus musculus]H1f11-ps H1.11 linker histone, pseudogene [Mus musculus]Gene ID:629389Gene
- OR52I1 olfactory receptor family 52 subfamily I member 1 [Homo sapiens]OR52I1 olfactory receptor family 52 subfamily I member 1 [Homo sapiens]Gene ID:390037Gene
- ZACN zinc activated ion channel [Homo sapiens]ZACN zinc activated ion channel [Homo sapiens]Gene ID:353174Gene
- SHROOM1 shroom family member 1 [Homo sapiens]SHROOM1 shroom family member 1 [Homo sapiens]Gene ID:134549Gene
Your browsing activity is empty.
Activity recording is turned off.
See more...