NCBI Bookshelf. A service of the National Library of Medicine, National Institutes of Health.
Institute of Medicine (US) Committee on Military Nutrition Research. The Role of Protein and Amino Acids in Sustaining and Enhancing Performance. Washington (DC): National Academies Press (US); 1999.

The Role of Protein and Amino Acids in Sustaining and Enhancing Performance.
Show detailsDennis M. Bier1
Origin of the Word "Protein"
How protein got its name is an interesting story (Hartley, 1951). The traditional credit for coining the word "protein" goes to the Dutch chemist Gerardus Johannes Mulder, who in an article published in the Bulletin des Sciences Physiques et Naturelles en Neerlande on July 30, 1838, stated (in French) that this material was the essential general principle of all of the constituents of the animal body and defined it by the Greek word "proteus," which he translated in Latin to "primarius," that is, the primary constituent of the body (Hartley, 1951). The interesting part of the story is that Mulder appears to have taken the term directly from Swedish chemist Sac Berzelius, who, on July 10, sent Mulder a letter in which Berzelius suggested the name "protein."
Besides the apparent use of the new term without proper attribution was the situation of a Dutch chemist, writing in a Dutch journal, defining a new word in French that was derived from Greek, and then qualifying its meaning in Latin! Further, considering that this episode took place 160 years ago, not only was the mail a lot faster, but also the publication time was substantially shorter than it is today, since the entire story took place in a period of about 3 weeks!
Admittedly, Berzelius and Mulder were right: Protein is the essential general principle of the constituents of the animal body. Thus, one might briefly summarize the physiological roles of protein in metabolism as "responsible for just about everything." But, this is neither particularly helpful nor informative.
Functions of Body Proteins and Amino Acids
Table 5-1 lists a variety of the functions of body proteins and amine acids. From the practical standpoint of integrative human physiology helpful to the military, the major roles of body proteins are (1) those relating to protein synthesis and protein breakdown in the context of maintaining lean body mass, (2) efficient operation of regulatory proteins required for conduct and optimization of body functions, and (3) the energy costs of the above, including the cost of oxidation and excretion of protein metabolites resulting from the metabolic reactions constituting these events. The net physiological results that are useful to military personnel include increased strength, improved endurance, optimization of "fight or flight" reactions (preferably the former), efficient blood coagulation and wound healing, enhanced immunological functions with improved disease resistance, and peak mental alertness and memory.
TABLE 5-1
Some Functions of Amino Acids and Their Products.
What is often forgotten in this context is that protein turnover is an energy-requiring process. Further, the energy cost of protein metabolism is higher than conventionally estimated. These observations are important because one of the principal lessons learned from prior Committee on Military Nutrition Research reports, such as the Ranger studies, is that biological energy demands often far exceed dietary energy intakes in combat field circumstances. Thus, while strength and endurance might immediately be identified with protein metabolism, the energy costs of (1) the protein enzymatic reactions involved in fight or flight reactions, (2) the synthesis of host defense proteins, or (3) memory storage might not be so readily apparent.
Protein Metabolism as an Energy-Requiring Process
In 1989, Waterlow and Millward calculated the daily energy cost of protein turnover as approximately 18 kJ (4.3 kcal)/kg body weight, or about 20 percent of the basal metabolic rate. This estimate, made from the best information available at the time, tallied the energy costs of protein breakdown and regulation as "probably negligible" and did not include the energy costs of posttranslational modifications. Today, however, it is known that these costs are not negligible. Using another approach relating protein (ATP) utilization to total ATP synthesis, Reeds et al. (1985) came to roughly similar conclusions but, after addressing the potential additional costs of all the cellular regulatory reactions, estimated that these control reactions and other, unaccounted-for, costs would increase the true energy cost of protein metabolism significantly.
Young and Yu (1996) have listed some of these energy-dependent processes (Table 5-2). In addition to the costs of protein synthesis, there is an energy-dependent cost of protein degradation via the ubiquitin pathway.
TABLE 5-2
Some Energy-Dependent Processes Associated with Protein Metabolism.
Similarly, there are a large number of energy-dependent processes involved in the regulation of protein metabolism, as expanded on below, and there are other assorted costs attached to the body's nitrogen metabolism cycles. The latter cycles are addressed by others in this volume.
Energy Costs of Protein Synthesis and Regulation
Of first concern are the energy costs of cell replication. Before the cost of protein synthesis itself can be calculated, one must recognize that the proteolytic degradation of cyclins regulates the movement from one phase of the cell cycle to the next through energy-dependent ubiquitin pathways (King et al., 1996; Martin-Castellanos and Moreno, 1997). Of second concern is that mitotic events, including spindle microtubular growth, and spindle elongation during anaphase require hydrolysis of GTP or ATP.
Protein synthesis itself has a higher cost than previously estimated. While transcription of the amine acid mRNA codon requires six ATP per amine acid and activation to amine acyl-tRNA requires another two ATP, there is disagreement about the total energy cost of mRNA translation. In addition to the one ATP per peptide required for capping the 5-prime end of the peptide, at least one GTP per peptide bond is required for initiation, two GTP per bond for elongation, and one GTP per peptide for termination. However, recent evidence suggests that one additional molecule of GTP is required for chain elongation (Schimmel, 1993), and hydrolysis of an additional GTP might be required during initiation and/or at the termination step as well. If hydrolysis of these additional high-energy bonds is proven correct, the net energy cost of protein synthesis alone will increase significantly from estimates made a decade ago.
In addition, there are a variety of other costs that are difficult to estimate. All of the additional sequences that are involved in, for example, synthesizing ''pre-proteins" and "pre-pro-proteins" and the costs of alternate splicing are not easy to quantify. In a sense, synthesizing and then removing these unused peptide sequences is wasted energy unless some as-yet-unknown energy advantage is discovered for this process. Similarly, the cost of synthesizing nonessential amino acids that are required for protein synthesis and the costs of posttranslational modifications are not known with certainty.
Further, the folding (Hartl, 1996) and the movement of the synthesized proteins to their sites of action (Rothman and Wieland, 1996) are highly energy-dependent processes. ATP-dependent mechanisms are required for polypeptide chain folding by heat-shock protein 70 and the chaperonin families of molecular chaperones (Hartl, 1996). Translocation across the membrane of the rough endoplasmic reticulum is an energy-dependent process, as is each transport step to the cis, medial, and trans Golgi compartments (Rothman and Wieland, 1996).
Table 5-3 shows some of the protein targeting costs for secretory and nonsecretory proteins. The net actual costs of these events to the whole organism are difficult to estimate with any accuracy or precision, but they are likely to be significant.
TABLE 5-3
Protein Targeting Costs.
In addressing the potential energy costs of regulatory proteins (Table 5-4), one is struck by the fact that, although these proteins do not represent much in the way of mass compared with structural proteins, their turnover rates are very high. Therefore, they may represent a significant energy drain. It is now known, for example, that an immense number of processes are controlled by reversible enzymatic phosphorylation/dephosphorylation reactions. This central mechanism of regulatory control occurs in every cell at an unimaginable number of times per minute. What the actual net energy cost of these regulatory events is to the whole body is only speculative, but likely significant.
TABLE 5-4
Energy-Dependent Processes in the Turnover of Regulatory Proteins.
Signal transduction processes, including those mediated by the more than 100 known members of the protein kinase family, and the energy-dependent costs of the second messenger families, for example the phosphatidyl and inositol kinases, are additional energy costs of regulatory protein metabolism. Furthermore, the energy costs of posttranslational modifications such as protein glycosylation are additional. The addition of each nucleotide sugar costs the hydrolysis of one uridine triphosphate bond. Approximately 10 percent of proteins are glycosylated. Each protein averages between 2 and 5 sugar chains, and each sugar chain averages about 12 sugars. Precisely how to tally this cost in the overall sum of the total daily energy cost of protein turnover, is not known, but the cost is potentially large.
Energy Costs of Protein Breakdown
Finally, it is important to address the potential energy costs of protein breakdown. There are two principal routes of protein degradation (Figure 5-1) (Mitch and Goldberg, 1996; Young and Yu, 1996). Most cytosolic, nuclear, and mitochondrial proteins are degraded through energy-dependent pathways requiring hydrolysis of ATP (Gottesman et al., 1997). Extracellular and membrane proteins are degraded through the lysosomal pathway. Although the latter route is not an energy-dependent process in itself, energy is required for proton movement into and maintenance of the acid environment within the lysosome. In Table 5-5, Mitch and Goldberg (1996) have summarized some of the conditions that alter muscle protein degradation through the energy-dependent ubiquitin-proteosome pathway. It is clear from this table that the pathophysiological regulation of this pathway might affect energy demands in a wide variety of clinical circumstances.
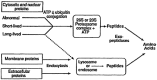
Figure 5-1
Major Pathways of Protein Degradation. Source: Young, V.R. and Y.M. Yu, 1996. Protein and amino acid metabolism. Pp. 159-200 in Nutrition and Metabolism in the Surgical Patient, 2nd Edition, Josef E. Fischer, ed. Boston: Little, Brown and Company.
TABLE 5-5
Conditions That Alter Muscle Protein Degradation Through the Ubiquitin-Proteosome Pathway.
This thesis is not merely an academic one. Price et al. (1996) demonstrated that the accelerated proteolysis observed in insulinopenic, streptozocin-diabetic rats was the consequence of activation of the energy-dependent ubiquitin-proteosome pathway. Similarly, Tiao et al. (1997) have demonstrated an increase in the ubiquitin and 20S proteosome subunit HC3 mRNA levels in rectus abdominis muscle obtained from septic patients.
Conclusion
The energy-dependent processes of maintaining the turnover of body proteins, including synthesis, folding, targeting, regulatory processes, and protein breakdown, have an overall cost to body energy homeostasis that is significantly higher than previously appreciated. The work of Tiao et al. (1997) confirms the human implications of animal studies like those of Price et al. (1996), and strongly suggests that circumstances that increase energy-dependent proteolytic mechanisms have practical clinical consequences for whole body energy homeostasis. It is not known yet how to approach a precise quantification of these costs at the whole body level. Nonetheless, investigators should give more thought to this question; there are important clinical circumstances where the energy costs of protein metabolism are likely to result in disproportionately increased energy demands. The high physical exertion levels of military personnel in the field may be one of these circumstances.
References
- Gottesman, S., M.R. Maurizi, and S. Wickner. 1997. Regulatory subunits of energy-dependent proteases. Cell 91: 435-438. [PubMed: 9390551]
- Hartl, F. U. 1966. Molecular chaperones in cellular protein folding. Nature 381: 571-580. [PubMed: 8637592]
- King, R.W., R.J. Deshaies, J.-M. Peters, and M.W. Kirschner. 1996. How proteolysis drives the cell cycle. Science: 274: 1652-1659. [PubMed: 8939846]
- Martin-Castellanos, C., and S. Moreno. 1997. Recent advances on cyclins, CDKs and CDK inhibitors. Trends in Cell Biol. 7: 95-98. [PubMed: 17708915]
- Mitch, W.E., and A.L. Goldberg. 1996. Mechanisms of muscle wasting. N. Engl. J. Med. 335: 1897-1905. [PubMed: 8948566]
- Price, S.R., J.L. Bailey, X. Wang, C. Jurkovitz, B.K. England, X. Ding, L. S. Phillips, and W.E. Mitch. 1996. Muscle wasting in insulinopenic rats results from activation of the ATP-dependent, ubiquitin-proteosome proteolytic pathway by a mechanism including gene transcription. J. Clin. Invest. 98: 1703-1708. [PMC free article: PMC507607] [PubMed: 8878419]
- Reeds, P.J., M.F. Fuller, and B.A. Nicholson. 1985. Metabolic basis of energy expenditure with particular reference to protein. Pp. 46-47 in Substrate and Energy Metabolism in Man, J. S. Garrow, editor; and D. Halliday, editor. , eds. London: John Libby.
- Rothman, J. E., and F. T. Wieland. 1996. Protein sorting by transport vesicles. Science 272: 227-234. [PubMed: 8602507]
- Schimmel, P. 1993. GTP hydrolysis in protein synthesis: two for tu? Science 259: 1264-1265. [PubMed: 8446896]
- Tiao, G., S. Hobler, J.J. Wang, T.A. Meyer, F.A. Luchette, J.E. Fischer, and P-0. Hasselgren. 1997. Sepsis is associated with increased mRNAs of ubiquitin-proteosome proteolytic pathway in human skeletal muscle. J. Clin. Invest. 99:163-168. [PMC free article: PMC507782] [PubMed: 9005983]
- Waterlow, J. C., and Millward, D. J. 10. 1989. Energy cost of turnover of protein and other cellular constituents. Pp. 277-282 in Energy Transformations in Cells and Organisms, W. Wieser, editor; and E. Gnaiger, editor. , eds. Stuttgart: Georg Thieme Verlag.
- Young, V.R. and Y.M. Yu. 1996. Protein and amino acid metabolism. Pp. 159-200 in Nutrition and Metabolism in the Surgical Patient, 2nd Edition, Josef E. Fischer, editor. , ed. Boston: Little, Brown and Company.
Discussion
DR. NESHEIM: Questions?
DR. MILLWARD: If I can just speak briefly in defense of John Waterlow's original interpretation.
DR. BIER: By the way, do not take this as a criticism of John. He worked with the data available.
DR. MILLWARD: I would just like to make the comment that one of the difficulties with these sorts of arguments is definition of what you are talking about when you are talking about protein turnover. You made the initial statement that proteins are involved in everything that goes on in the cell. You talked about a wide range of protein-related functions that are energy-dependent. There is no question about that. Therefore, it is possible to subsume ultimately all energy expenditure under the heading of protein-related functions if you are not careful in the way in which you have defined the process that you are talking about.
DR. BIER: I agree with that. That is, in the end, since you cannot live without the proteins required for life, then essentially all energy generation is dependent on protein turnover. I think that is a very good point, Joe, and you are correct in the sense of defining the limits of the argument.
I guess what I was trying to do was to move us from what I would call the traditional nutritional thinking on this issue, that is, that the costs are related to the net synthesis and oxidation of protein, the protein turnover of macromolecules, and the maintenance of lean mass, and move us to appreciate further that there are additional costs that many physiologists have not spent a lot of time thinking about.
DR. LIEBERMAN: Under conditions of relatively mild undernutrition, similar to what we see in your study, do you think there might be some better markers to tell us that protein balance is not where we would like it to be?
DR. BIER: I am sure there are, since use of all available probes of a very complicated system should give you more information. But is there any individual marker? I do not know. I think that some of the new things, like ubiquitin mRNA and the like, may give us an indication.
DR. LIEBERMAN: It would be nicer if we had something that was more fine grained.
LTC FRIEDL: I would not dismiss the turnover rate. We see that in undernutrition. That is a good example of where all these processes can be reduced. What are the consequences in the big picture? How much leeway do we have in adjusting the rate of protein turnover.
DR. BIER: Well, I am not sure you can turn it down in the circumstance of active physical exertion, for example. That is, during the period of exercise itself, protein turnover may "turn down, "but there is a period where you have to then "turn it up". I do not think in the context of continued strenuous activity you can significantly reduce the overall cost.
LTC FRIEDL: Maybe there is a compromise between the demands of physical activity and deficient energy intake. Undernutrition seems to favor the process of turning down the overall turnover.
DR. BIER: Yes, that is in a circumstance where the person is generally lying in bed or voluntarily reducing exertion overall.
LTC FRIEDL: It is not a suboptimal performance.
DR. BIER: Michael Rennie is going to talk about physical exercise later, and I will defer this to him.
DR. NESHEIM: Yes.
DR. HOYT: Colonel Friedl, Jim Delany, and I did a study of Norwegian Rangers who did not eat or sleep for a week, and we looked at their body composition with dual X-ray Absorptiometry and their energy expenditure with doubly-labeled water. You can look at the contribution of fat and carbohydrate and protein to that negative energy balance. Would you expect anything other than a 4 kcal/g contribution from the protein loss reflecting the savings associated with not supporting some of the synthetic costs?
DR. BIER: That is a net protein loss. The actual cost is higher. But I take Joe's argument that, in the end, one can attribute all the energy cost somehow or other to protein. I guess, then, this goes back to the issue of whether we can change the efficiency or improve the net energy balance cost by adding protein in that sort of circumstance? I am not sure that I know the answer.
DR. NESHEIM: Thank you. We need to move on. This is a very good stage setting for a lot of discussion that will come along. Now, we want to move to a discussion of the regulation of muscle mass and functions by Dr. Nair from the Mayo Clinic
Protein and Amino Acids, 1999
Pp. 121-136. Washington, D.C.
National Academy Press
Footnotes
- 1
Dennis M. Bier, Children's Nutrition Research Center, Baylor College of Medicine, Houston, TX 77030-2600.
- The Energy Costs of Protein Metabolism: Lean and Mean on Uncle Sam's Team - The ...The Energy Costs of Protein Metabolism: Lean and Mean on Uncle Sam's Team - The Role of Protein and Amino Acids in Sustaining and Enhancing Performance
- MIR34C [Pan troglodytes]MIR34C [Pan troglodytes]Gene ID:100316149Gene
- ORF1ab [SARS coronavirus Tor2]ORF1ab [SARS coronavirus Tor2]Gene ID:1489680Gene
- RAD21-AS1 RAD21 antisense RNA 1 [Homo sapiens]RAD21-AS1 RAD21 antisense RNA 1 [Homo sapiens]Gene ID:644660Gene
- DDR1-DT DDR1 divergent transcript [Homo sapiens]DDR1-DT DDR1 divergent transcript [Homo sapiens]Gene ID:414771Gene
Your browsing activity is empty.
Activity recording is turned off.
See more...