NCBI Bookshelf. A service of the National Library of Medicine, National Institutes of Health.
National Research Council (US) Committee on Technological Options to Improve the Nutritional Attributes of Animal Products. Designing Foods: Animal Product Options in the Marketplace. Washington (DC): National Academies Press (US); 1988.
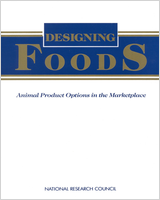
Designing Foods: Animal Product Options in the Marketplace.
Show detailsJ. G. LINN
Milk composition is economically important to milk producers and processors and nutritionally important to consumers. It has been known for years that variations in milk composition occur; however, the composition of milk marketed nationally has been rather constant over the last 15 years, averaging 3.6 percent fat, 3.2 percent protein, and 4.7 percent lactose (Young et al., 1986). This is probably partly because of the prominence of the Holstein breed and the pricing of milk based on fat concentration. The introduction of milk pricing on a component basis and the perception by consumers that animal fats are unhealthy have created new interest in how milk components can be altered to accommodate these emerging markets. The paper starts with a brief overview of the biosynthesis of milk components since changes in these reflect changes in the mammary gland synthesis or secretion of the component. Factors affecting milk composition such as breed, genetic variation within breed, health, environment, management practices, and diet are then reviewed.
Lipids
Biosynthesis
The synthesis of milk fat has been recently reviewed (Dils, 1983, 1986; Larson, 1985; Book and Thomas, 1980). The following discussion highlights relevant stages of biosynthesis where fat composition can be altered.
The predominant fat in milk is triacylglycerol, which contains fatty acids of short- (C4-C10), intermediate- (C12-C16), or long-chain (C18) length. The short-chain acids are synthesized within the mammary. gland from acetate and beta-hydroxybutyrate; long-chain acids are almost exclusively derived from blood plasma fatty acids of dietary origin; and intermediate-chain acids arise from both sources. In broad terms, about 50 percent of the fatty acids in milk are synthesized in the mammary gland and the other 50 percent are derived directly from blood.
Fatty acids are synthesized in the mammary gland via the malonyl coenzyme A (malonyl-CoA) pathway. Blood beta-hydroxybutyrate is primarily used for the first four carbons initiating fatty acid synthesis. Chain length occurs two carbons at a time, with acetate being the major carbon donor. Once formed, fatty acyl-CoAs may combine with glycerol or diacylglycerol or monoacylglycerol to form triacylglycerol. Placement of the fatty acids on the glycerol molecule is not random. Short-chain fatty acids are essentially in the 3 position, and C18 acids are on either the 1 or 3 position.
Triacylglycerols are synthesized in the endoplasmic reticulum. As they are formed, they are rapidly incorporated into lipid-covered droplets. The droplets then migrate toward the apical membrane of the secretory cell, where they become encapsulated by the membrane, pinched off, and released into the lumen.
Factors Affecting Milk Fat Content
Breed/Genetics
Between and within breeds, fat varies the most and lactose the least (Woodford et al., 1986). Gaunt (1980) reported cattle in the United States tend to have the lowest percentage of milk fat. This may be partly because of environmental factors, but some genetic variation within a breed in different countries must exist.
The repeatability from one lactation to another for the percentage of constituents in milk is quite high, an average of 0.67 (Gaunt, 1980). Repeatability of milk fat percentage for Holsteins is 0.76. Other breeds appear to have a similar repeatability.
Jerseys have the highest heritability for milk fat percentage (0.71), with other breeds ranging from 0.51 to 0.57. The small variation between ratios of one milk constituent to another, particularly fat to protein, suggests little hope for drastic changes in milk yield and milk composition (Gaunt, 1973; Wilcox, 1978). Heritabilities of solids-not-fat (SNF) to fat and protein to fat ratios are highest for Ayrshire followed by Jersey, Guernsey, Brown Swiss, and Holstein. Differences in heritabilities of breeds other than Holstein may be overestimated because of a small sample population.
Genetic correlations between milk composition percentages are high and positive, averaging 0.74. However, milk yield and composition percentages are negatively correlated, -0.3 for milk yield and fat percentage (Gaunt, 1980). Thus, it is very difficult to improve milk yield and milk percentage composition simultaneously.
Selection in Holstein cattle for the single trait of milk fat percentage would decrease milk yield by 287 pounds but increase fat percentage by 0.19 percent per generation. Selection for milk yield only increases milk yield by 607 pounds and decreases fat percentage by 0.036 percent. Selection for milk fat yield is the most effective method for increasing fat percentage (+ 0.058 percent) and milk yield (+443 pounds) (Gaunt, 1980).
Environment/Management
A decrease in milk fat percentage of 0.2 percent over five lactations has been reported by Rogers and Stewart (1982). Fat yields would be expected to increase, since the increase in milk yields with age more than offsets the drop in fat percentage.
Milk fat percentages vary with stage of lactation. The highest percentages are usually found in colostrum, followed by a decline during the first 2 months of lactation, then a slow increase as lactation progresses. Davies et al. (1983) reported distinct changes in the fatty acid content of milk over the lactation cycle. During the first half, the proportions of short- and intermediate-chain fatty acids increase, and the proportion of long-chain fatty acids decreases. No further changes occur during the last half of lactation. Some of these changes are influenced by environment, diet, and rates of fatty acid synthesis in the mammary gland.
Seasonal variations in milk fat percentages are well recognized, with summer months averaging 0.4 percentage units less than winter months (Jenness, 1985). The higher environmental temperatures during the summer also affect milk fatty acid composition. Milk fat in the summer tends to be lower in palmitic acid relative to stearic and octadecanoic acids than milk fat from the same cows during the winter (Christie, 1979). Some of the changes in milk fat percentage and composition with temperature change can be related to changes in blood plasma lipids, but these observations are also confounded by dietary changes. Milam et al. (1986) observed no change in milk fat percentage when heat-stressed cows were given water at 10 or 28ºC.
The fat percentage of milk increases continuously during the milking process, with the lowest fat milk drawn first and the highest fat milk drawn last. The increase in fat percentage throughout the milking process is due to the clustering of fat globules trapped in the alveoli (Jenness, 1985). Thus, if cows are not milked out completely, fat percentage will be lower than normal, but, at the next milking, fat content will be higher than normal. Furthermore, when milking intervals are unequal, the highest fat percentage is obtained after the shortest interval (Wheelock, 1980). Milk fatty acid composition is not affected by milking interval or time of day milking (Christie, 1979). The effect of milking three versus two times a day on milk fat percentage has varied, with some researchers reporting no change (Amos et al., 1985; DePeters et al., 1985; Gisi et al., 1986) and others reporting decreases (Allen et al., 1986; Gisi et al., 1986).
Health/Physiology
Mastitis (inflammation of the udder) generally causes a decline in milk fat percentage and a change in milk fat composition (Kitchen, 1981; Needs and Anderson, 1984; Schultz, 1977). The decrease in fat percentage, however, is less (about 10 percent) than that observed for lactose or casein (about 15 percent). Reported changes in milk fat composition from mastitis have varied. There is general agreement on increases in amounts of free fatty acids and short-chain fatty acids, but both increases (Needs and Anderson, 1984) and decreases (Kitchen, 1981; Schultz, 1977) in phospholipid and long-chain fatty acids have been reported.
The effects of hormones on milk fat percentage are not well known (Bauman and Elliot, 1983; Tucker, 1985). It has been demonstrated that adrenaline and noradrenaline increase lipolytic activity in adipose tissue, but their effect on milk fat is unknown. Administration of exogenous growth hormone has resulted both in no change (Bauman et al., 1985; Peel et al., 1985) and in changes (Eppard et al., 1985) in milk fat percentage and composition. At low doses (5 and 10 IU/day), growth hormone lowered fat percentage with no change in fat composition, but at high doses (50 and 100 IU/day), milk fat percentage was increased and milk fat contained more endogenous fatty acids (Eppard et al., 1985). Growth hormone affected both synthesis of fatty acids in the mammary gland and uptake of preformed fatty acids from the blood, depending on dose level and energy balance of the cow. Sutton (1980) reported that the use of thyroprotein, 1,3-butanediol, and glucocorticoids have generally not increased milk fat percentage.
Nutrition
Diets for today's high-producing dairy cows are typically higher in energy from readily fermentable carbohydrates than fats. Feeding of these diets often causes a condition known as low-milk-fat syndrome. Characteristics of low-milk-fat syndrome are a reduction in milk fat percentage (as much as 60 percent) and changes in milk fat composition (an increase in C18 polyunsaturated and monounsaturated acids and decreases in C160 and C180 fatty acids) (Banks et al., 1983; Christie, 1979). Causes of low-milk-fat syndrome probably involve both an alteration in rumen fermentation and availability of endogenous fatty acid sources (Christie, 1979). Feeding of readily fermentable carbohydrates depresses fiber digestion and pH in the rumen and thus decreases acetic and butyric acid production and increases propionic acid production. Increased propionic acid concentrations in the rumen lead to increased lactic acid and glucose production, which, in turn, stimulates insulin production, reducing free fatty acid release from adipose tissue. Thus, the main precursors of milk fat (acetic and butyric acids derived from rumen fermentation, long-chain fatty acids of dietary origin, and acetic acid and long-chain fatty acids from endogenous sources) can be affected by diet through changes in rumen fermentation or addition of fats for direct absorption and inclusion into milk fat.
Rumen Fermentation. Milk fat percentage is related positively to rumen molar percentages of acetic and butyric acids and negatively to that of propionic acid. Davis (1978) reported that rumen molar percentage of propionate must be above 25 before a highly significant negative relationship between milk fat percentage and propionate exists. Sutton (1980) estimated that 60 percent of the variations observed in milk fat percentage can be accounted for by changes in the molar proportion of propionate in the rumen.
A positive relationship exists between the molar ratio of acetate to propionate and milk fat percentage. A linear increase in milk fat percentage occurs as the ratio of acetate to propionate increases up to 2.2 (Davis, 1978). Above a ratio of 2.2 there is little change in milk fat percentage. Thus, diets that increase propionate production have the greatest effect on milk fat percentage.
Numerous dietary factors affect rumen fermentation (Sutton, 1980). Those most commonly associated with changes in the acetate to propionate ratio are forage to concentrate ratio, type of carbohydrate in the diet, physical form of the diet, processing of ingredients, additives, and the frequency and method of offering feed. The following discussion summarizes the influence of these factors on rumen fermentation, acetate to propionate ratio, and change in milk fat percentage.
The general effect of decreasing the forage to concentrate ratio on rumen fermentation is to decrease pH, increase propionic acid production, and reduce fiber digestion. Thus, as forage declines, milk fat percentage falls proportionately; however, milk fat yields may increase (Sutton, 1980). The critical forage to concentrate ratio appears to be about 40:60, beyond which additional concentrate drastically lowers milk fat percentage (Coppock, 1985; Sutton, 1985). However, Sutton (1980) reported that the actual level of forage needed in a diet to maintain normal milk fat percentage may be affected by total feed intake. At high levels of intake, more forage is needed than at low-intake levels to maintain the same milk fat percentage. Recent work by Shaver et al. (1986) has shown similar results, with milk fat percentages being higher in milk from cows fed a 60:40 forage to grain diet at 2.93 percent of body weight than at 3.75 percent of body weight. Declines in milk fat percentage with high-grain feeding are accompanied by a change in milk fatty acid composition from saturated fatty acids to more unsaturated acids, especially those containing 16 carbons or less (Banks et al., 1983; Sutton, 1980).
The type of forage and its effect on milk fat percentage are influenced by forage particle size, maturity, and fiber content of the forage. It has been known for a while that finely ground forages reduce milk fat percentage. Finely ground forages apparently result in higher levels of propionate being produced during rumen fermentation than forages of adequate particle size (Sutton, 1980). Recent work by Woodford et al. (1986) has shown that a mean forage particle length of 0.64 cm or more is needed to keep rumen molar percentage of propionate below 25 and milk fat above 3.6 percent. Mertens (1985) recommended a minimum of 28 percent neutral detergent fiber and about 18 percent acid detergent fiber in diets to maximize milk production and fat percentage. The daily amount of neutral detergent fiber needed was estimated to be 1.2 percent of body weight.
Stage of forage maturity is an important factor in the supply of adequate fiber in the diet. More immature alfalfa hay was required in the diet to obtain maximum production of 4 percent fat-corrected milk than when mid- or late-bloom alfalfa hay was fed (Kawas et al., 1983). Recent work (Hansen et al., 1984) has shown that an interaction between forage species and concentrate level in the diet affects milk fat percentage. Bromegrass supported a higher milk fat percentage at higher concentrate feeding than did alfalfa. No difference between the two forage sources was observed at lower concentrate levels.
Carbohydrate source can influence rumen fermentation and consequently milk fat percentage. Sutton (1985) reported that the lower ruminal degradability of corn compared with that of barley would result in the production of milk with a higher fat percentage. Recent work (DePeters and Taylor, 1985) has confirmed that barley-based concentrates tend to depress fiber digestibility, resulting in lower ruminal acetate to propionate ratios and lower milk fat percentages than those with corn-based concentrates. The higher digestion of barley in the rumen produces more propionate and results in less starch being presented to the lower digestive tract for conversion to glucose than with corn. However, the increased production of propionate in the rumen from barley appeared to stimulate milk yield more than glucose derived directly from corn in the lower digestive tract. The mechanism(s) by which these two differences in nutrient supply affect milk fat is not well known. Processing of grains such as grinding, rolling, heating, steam flaking, and pelleting increases digestion of the starch in the rumen and produces effects similar to those reported above for barley (Sutton, 1980).
Increasing butyric acid production in the rumen should also help to maintain or increase milk fat percentages. Sutton (1980) suggested that beet pulp is a promoter of butyric acid production in the rumen. Other carbohydrates such as whey (Casper and Schingoethe, 1986; Schingoethe, 1976), sucrose, and lactose (Sutton, 1980) have been evaluated as sources of soluble carbohydrate to prevent milk fat depression.
The pattern of feeding, often referred to as feeding strategy, was found to have little if any benefit in terms of increasing milk fat percentage under normal conditions (Linn and Otterby, 1984). However, under feeding regimes where fat-depressing conditions are likely, increasing the frequency of offering concentrates to six or more times per day appears to stabilize the rumen environment (Bragg et al., 1986) and increase milk fat percentage (Sutton, 1980, 1985).
The mixing of all feed ingredients before feeding does not affect milk fat yield or percentage any differently than if the ingredients were fed separately (Holter et al., 1977; Marshall and Voigt, 1975; Owen, 1981).
Thomas and Chamberlain (1984) summarized the effects of infusion of specific nutrients into cows on changes in milk constituents. Intraruminal infusions of acetic acid consistently increase milk yield, lactose yield, and milk fat yield, whereas infusions of propionate reduce milk fat yield. Glucose infusions, either intraabomasal or intravenous, increase milk yield and decrease milk constituent percentages. Infusions of protein or amino acids (Schwab et al., 1976) have had variable or no effect on milk fat percentage.
The effects of dietary protein on milk fat percentage are variable but generally small when diets within normally accepted ranges of nutrients have been fed (Sutton, 1980; Thomas and Chamberlain, 1984). Changes in fat percentage result from changes in milk yield rather than from a direct effect of dietary protein source or amount. Insufficient amounts of rumen-degradable protein may lower milk fat percentage because of a lack of ruminal ammonia for optimal microbial digestion of fiber and other feed-stuffs.
Additives such as buffers and methionine hydroxy analog have been used to promote increases in milk fat percentage. Cows in early lactation fed high-concentrate diets were shown to benefit from the inclusion of the methionine hydroxy analog in their rations (Lundquist et al., 1983). Feeding of 25 grams of methionine hydroxy analog daily during the first 120 days of lactation increased milk fat 0.35 percentage units.
Buffers are compounds used to raise rumen pH through the neutralization of volatile fatty acids. However, other modes of action have been indicated for the group of compounds commonly alluded to as buffers (sodium bicarbonate, potassium bicarbonate, limestone, magnesium oxide, and bentonite) (Chalupa and Schneider, 1985). In general, the bicarbonates have been effective in maintaining or increasing milk fat percentages of cows fed high-grain diets, especially when corn silage was the main forage source (Chalupa and Schneider, 1985; Davis, 1978; Sutton, 1980). Magnesium oxide has also been shown to help prevent milk fat percentage depression; however, it appears that the mechanism of action is through transfer of lipid into the mammary gland from blood rather than through a change in rumen fermentation (Chalupa and Schneider, 1985).
Added fats. Dietary fats can alter milk fat composition in a number of ways (Christie, 1979). One route is for fatty acids to be unaltered during digestion and absorption and therefore appear in milk fat directly. Another route is for the rumen microorganisms to hydrogenate the fatty acid, which can then appear in milk fat in this form or be further modified by desaturation before appearing in milk fat. Dietary fatty acids can appear in milk fat in the same form in which they were fed or be completely changed to another form before entering milk. In addition, the amount of particular fatty acids in the diet can alter lipid metabolism in the animal through mammary gland uptake problems or enzyme inhibitions. Dietary long-chain fatty acids can affect rumen fermentation and thus alter the amount of volatile fatty acids (acetic, propionic, and butyric acids) available for fat synthesis in the mammary, gland.
The use of fats and oils in the diets of dairy cows has received considerable attention (Fogerty and Johnson, 1980; Linn, 1983; Palmquist and Jenkins, 1980; Storry, 1980; Storry and Brumby, 1980). Numerous lipid sources, from natural to manufactured, have been evaluated. Their effects on milk yield and composition depend on type of fat, characteristics of the diet into which they are incorporated, rate and form fed, and method of feeding. Only a brief summary of changes in milk fat percentage and composition is reported here.
The changes in milk fat percentage and composition observed with the use of fat in diets of dairy cows are a reflection of the change in output of different fatty acids from the mammary gland; shortand medium-chain fatty acids (C4 to C14) are synthesized in the mammary gland, the C18 fatty acids come from the diet, and the C16 fatty acids come from both synthesis and dietary sources. Although dietary fats and oils may alter milk fat composition, the output of total milk fat depends on the balance of increased dietary transfer and decreased synthesis. However, there is probably a minimum content of short-chain fatty acids necessary to maintain melting points at body temperatures (Christie, 1979).
Both protected and unprotected fats and oils have been fed to dairy cows. Some of the unprotected fat or oil sources reported in the literature are tallow, yellow grease, vegetable oils, blends of animal-vegetable fats, and whole oilseeds (soybeans, sunflowers, cottonseed, and rapeseed). The common protected fat sources, so called because they are unavailable in the rumen and therefore do not alter rumen fermentation, fed are tallow and vegetable oils. Common methods of protection are formaldehyde-protein coating (Storry and Brumby, 1980) and formation of insoluble calcium salts of the fat (Jenkins and Palmquist, 1984).
In general, the addition of unprotected fat to dairy diets results in variable effects on milk yield and milk fat composition. The addition of fats, oils, or long-chain fatty acids depresses the synthesis of C4 to C16 fatty acids in the mammary gland. This most likely results from an alteration in rumen fermentation rather than an inhibition of mammary gland acetyl-CoA carboxylase activity (Banks et al., 1983; Storry, 1980; Thomas, 1980). The effect on rumen fermentation is most pronounced with unsaturated fatty acid feeding. Long-chain fatty acid sources (more than 20 carbons) such as fish oils and Seterculia seed fats have a specific inhibitory action on the uptake of preformed fatty acids by the mammary gland. The changes in milk fat composition that occur with fat feeding are predominantly in the triglyceride fraction, with very little change occurring in the phospholipid and fat membrane fractions (Storry, 1980).
Protected polyunsaturated fatty acids appear to be the most promising for consistently increasing milk fat percentage and altering milk fat composition. Protected oil-seeds or oils rich in linoleic acid (sunflower, corn, and soybean) produce large, rapid increases in the linoleic acid content of milk fat when fed. The increases in linoleic acid content are generally associated with declines in myristic, palmitic, and oleic acids. Transfer of linoleic acid from protected supplements to milk is reported to be between 20 and 40 percent (Christie, 1979; Fogerty and Johnson, 1980).
Feeding of protected saturated fats, the most common source being tallow, generally invokes the same response in increase of milk fat percentage as feeding of protected polyunsaturated fats. However, protected hydrogenated soybean oil has decreased the milk fat percentage (Banks et al., 1983). Protected tallow increases the amounts of C4, C161, C180, and C181 fatty acids found in milk fat (Christie, 1979). Similar results were reported for unprotected tallow.
Protein
The total (crude) protein content of milk is determined by analyzing milk for nitrogen and multiplying by a factor of 6.38. The total protein percentage of milk is generally considered to be about 3.5, of which 94 to 95 percent is in the form of true protein (Davies et al., 1983; Jenness, 1985). Casein accounts for approximately 80 percent of the true protein, and milk serum or whey proteins account for about 20 percent. Urea is the largest single nonprotein nitrogen (NPN) component, accounting for approximately 50 percent of the total NPN (Wolf schoon-Pombo and Klostermeyer, 1981).
Milk proteins fall into several families of polypeptide chains, for which a systematic nomenclature system has been defined (Eigel et al., 1984). Casein proteins are characterized by ester-bound phosphate, high proline contents, and few or no cysteine residues and are precipitable from milk at pH 4.6 and 20ºC. The main casein types in milk are alpha-, beta-, gamma-, and kappa-caseins. Whey proteins are distinguished from casein by remaining in solution upon precipitation of casein proteins. The major whey proteins are beta-lactoglobulin and alpha-lactalbumin. Serum albumin, immunoglobulins, proteose peptones, lactoferrin, and transferrin represent a smaller proportion of the whey protein fraction (Davies et al., 1983; Jenness, 1985; Kuzdzal-Savoie et al., 1980).
Biosynthesis
The synthesis of milk proteins has been extensively reviewed (Larson, 1979, 1985; Mercier and Gaye, 1083). In general, protein synthesis in mammary alveolar cells is similar to other protein synthesis systems in which DNA controls protein synthesis. Messenger RNA carries the encoded DNA message from the nucleus to the ribosomes located in the rough endoplasmic reticulum (RER) and cytoplasm. Ribosomes are composed of ribosomal RNA and several proteins combined into a ribonucleoprotein complex, which, in conjunction with transfer RNA, combines amino acids into peptide chains. As the polypeptide chains are elongated to form proteins, they pass out of the RER, through the lumen, and into the region of the Golgi apparatus where they accumulate and polymerize into different milk protein molecules. Casein must be phosphorylated, bound with calcium, and stabilized by calcium phosphate linkages and other ionic bonds before being released from the vesicles. The presence of alpha-lactalbumin in the region of the Golgi apparatus promotes synthesis of lactose. The secretory vesicles containing essentially nonfat milk constituents leave the cell by moving to the apical surface and fusing with the plasma membrane and discharging the vesicular contents into the cell lumen.
Most of the proteins present in milk are synthesized in the mammary gland, although some immunoglobulins and albumins are transferred from the blood (Larson, 1979). Blood leukocytes can also cross mammary barriers either by passing between secretory cells or by pushing secretory cells directly into the lumen. Urea diffuses freely across mammary cells, so there is a high correlation between blood plasma and milk urea concentrations (Thomas, 1980).
The synthesis of milk protein requires that both essential and nonessential amino acids be supplied to the mammary gland (Clark et al., 1978; Mepham, 1982). Uptake of free amino acids from the blood by the mammary gland can occur via several transport systems (Baumrucker, 1985). Mepham (1982) has classified essential and nonessential amino acids into three groups according to uptake by the mammary gland. Group I essential amino acids (methionine, histidine, phenylalanine, tyrosine, and tryptophan) are taken up in amounts just sufficient to meet milk protein synthesis needs. Group II essential amino acids (valine, leucine, isoleucine, arginine, lysine, and threonine) are taken up in excess. However, some data (Thomas, 1983) suggest that lysine and possibly leucine, isoleucine, and threonine should also be included in group I. Group III is the nonessential amino acids. The amounts taken up vary with animal, time, and availability. In addition to free amino acid uptake from blood, there is evidence that red blood cells and the recycling of amino acids also contribute to the cellular amino acid pool (Baumrucker, 1985). Breakdown of red blood cell glutathionine can make a significant contribution to the amount of cysteine, glycine, and glutamic acid available in the cell. Recycling of casein proteins is reported to account for at least 7 percent of the protein synthetic capacity in the mammary gland.
Factors Affecting Milk Protein Content
Breed/Genetics
Breeds differ in total milk protein percentage and type of milk protein produced. Jersey and Guernsey cattle have the highest percentages of total protein, casein, and whey. Variability of the major protein fractions within breeds has also been reported (Rolleri et al., 1956), with Holstein milk containing less of the major caseins and more gamma-casein than milk from other breeds. Genetic variants have been demonstrated for the milk protein groups, and breed differences have been found for the frequency of occurrence of these variants (Gaunt, 1980).
Genetic selection would increase the percentage of protein in milk 0.075 percentage units but decrease milk yield 231 pounds. Joint selection for milk yield, protein, and fat is recommended if the desired result is increased yield of protein and fat (Gaunt, 1980; Van Vleck, 1978; Wilcox, 1978). Gaunt (1980) estimated that it would take about 11 generations for milk protein percentages to equal milk fat percentages if protein yield with no change in fat percentage were used as the selection criterion.
Environment/Management
Age has a significant effect on milk protein percentage and composition in cows (Jenness, 1985; Ng-Kwai-Hang et al., 1982; Rogers and Stewart, 1982). Milk protein percentage declines in cows older than 3 years, with a 0.4 percentage unit drop being reported over five lactations (Rogers and Stewart, 1982). This decline appears to be primarily in the casein fraction; however, changes in whey protein fractions have also been reported (Kroeker et al., 1985). Suggested reasons for the change are deterioration of udder tissue, selective culling for high production, and increased incidences of mastitis. The increase in immunoglobulins with advancing age reported by Kroeker et al. (1985) supports the latter suggestion.
Stage of lactation has a considerable influence on milk protein concentration (Davies et al., 1983; Ng-Kwai-Hang et al., 1982, 1985; Rogers and Stewart, 1982). At the beginning of lactation, colostrum is exceptionally rich in protein containing large quantities of immunoglobulins and about twice the levels of casein, beta-lactoglobulin, and alpha-lactalbumin found in mid-lactation milk. Total protein amounts fall rapidly during the first few days of transition from colostrum to normal milk and reach a minimum about 5 to 10 weeks into lactation, corresponding inversely to maximum milk yield. Thereafter, the amount of protein tends either to increase gradually as lactation progresses or to rise sharply when the cow becomes pregnant.
Milk protein percentage (Ng-Kwai-Hang et al., 1982) and yield (Keown et al., 1986) are higher during fall and winter than spring and summer. However, stage of lactation and feeding practices confound these observations as cows on spring pasture have elevated milk protein concentrations (Rogers and Stewart, 1982). Whey proteins have been found to have no definite seasonal variations (Kroeker et al., 1985). High environmental temperatures, above 29ºC, have been suggested to depress milk protein percentage, but cows offered cold water (10ºC) during heat stress do not show increased milk protein concentrations over cows offered 28ºC water (Milam et al., 1986).
Variations in milking procedure or frequency have a minor effect, if any, on milk protein percentage. Milk protein or SNF percentages do not change during the milking process (Jenness, 1985). Extended milking intervals do not change milk protein or SNF percentages until intervals exceed 16 hours (Rogers and Stewart, 1982). Increasing milking frequency from twice to three times daily for more than 15,000 Holstein cows did not change the percentage of SNF (Gisi et al., 1986). Similar results were reported by Amos et al. (1985) and DePeters et al. (1985).
Health/Physiology
Mastitis has very little effect on total milk protein percentage; however, it drastically alters the composition of milk protein (Kitchen, 1981; Schultz, 1977). The general effect of mastitis is to impair milk synthesis and loosen the connections between cells, thereby increasing permeability of blood constituents (Jenness, 1985; Wheelock, 1980). Milk proteins synthesized in the mammary gland (caseins, beta-lactoglobulin, and alpha-lactalbumin) decrease (Kitchen, 1981; Schultz, 1977), whereas blood serum proteins (whey proteins) increase (Kitchen, 1981; Kroeker et al., 1985; Poutrel et al., 1983; Schultz, 1977). Grappin et al. (1981) reported a whey protein to total protein ratio increase of 2.08 percent and a casein to total protein ratio decrease of 1.85 percent for every I log unit increase in somatic cell count. The same change in somatic cell count was reported by Ng-Kwai-Hang et al. (1982) to decrease the ratio of casein to total protein by 2.79 percent.
The hormone requirement for milk synthesis and secretion is prolactin, adrenocorticotrophic hormone, and estrogens and the relative absence of progesterone. Of particular importance to milk protein synthesis is prolactin (Tucker, 1985). Current studies (Bauman et al., 1985; Peel et al., 1985) on administration of exogenous growth hormone have generally shown increases in milk yield without significant changes in composition. However, Eppard et al. (1985) observed a slight decrease in milk protein percentage and an increase in alpha-lactalbumin as a percentage of total milk protein with increasing dosage levels (0 to 100 IU/ day) of bovine growth hormone.
Nutrition
Dietary crude protein affects milk yield and consequently milk protein yield more than milk protein percentage (Emery, 1978; Kaufman, 1980; Thomas, 1980, 1983). A small effect of dietary crude protein concentration on milk protein percentage was reported by Emery (1978): a 0.02 percentage unit increase in milk protein with every 1 percentage unit increase in dietary crude protein between 9 and 17 percent. More recently, Cragle et al. (1986) reported an increase of 0.1273 Mcal in milk protein energy content per i Mcal gross energy increase in feed protein. Neither of these studies, however, considered source of dietary crude protein or change in milk protein composition. Thus, the increases in milk protein observed may have been in milk NPN and not true milk protein. Elevated milk protein concentrations from cows fed diets high in rumen-degradable protein or NPN most likely will be from increased milk urea or NPN levels (Oltner et al., 1985; Thomas, 1980). On the other hand, diets low in rumen-degradable protein or balanced for optimal microbial protein synthesis should increase supplies of amino acids available to the mammary gland for protein synthesis, and thus, more true milk protein should be produced (Kaufman, 1980; Old-ham, 1984; Thomas, 1980). However, the proportions between true milk proteins (caseins, beta-lactoglobulin, and alpha-lactalbumin) do not appear to change with increases or decreases in milk protein synthesis (Thomas, 1983).
In experiments where protein (usually casein) has been abomasally infused to increase amino acid supplies to the tissue, increases in milk protein percentage along with milk yield have been reported (Clark, 1975; Clark et al., 1977). Abomasal infusions of amino acid mixtures also increased milk protein percentage, with methionine and lysine accounting for more than 68 percent of the observed increase (Schwab et al., 1976). Based on these responses, it could be concluded that increasing the intestinal supply of amino acids through increased rumen protein synthesis or low rumen-degradable protein sources would increase milk protein percentage and probably milk yield. However, on a practical feeding basis, milk protein responses to dietary, proteins with different rumen degradabilities have been quite variable but generally of no effect. A number of studies (Crawford and Hoover, 1984; Crooker et al., 1983; Forester et al., 1983; Henderson et al., 1985; Holter et al., 1985; Kung and Huber, 1983; Lundquist et al., 1986) reported no increases in milk protein when protected proteins were fed. Madsen's (1982) study, however, reported significant increases. Again, none of the studies cited determined milk protein composition except that of Lundquist et al. (1986), which showed no change in milk NPN content within equal dietary crude protein percentages due to feeding formaldehyde-treated soybean meal compared with feeding an untreated soybean meal.
Kaufman (1980) summarized the effects of dietary protein supply on milk protein concentration. Insufficient amounts of dietary protein will reduce milk protein concentrations, but the reduction is minimized when low rumen-degradable protein supplements are fed. Increasing dietary crude protein supply has little effect on milk protein percentage.
The amount of energy consumed, density of energy in the diet, and the source of energy in the diet all influence milk protein percentage and yield. Cragle et al. (1986) compared 59 percent versus 49 percent concentrate feeding and found that cows fed rations containing 59 percent concentrate produced an average of 11 percent more milk, 13 percent more protein, 3 percent more fat, and 11 percent more lactose than cows fed 49 percent concentrate rations. Of the increase in milk protein, 85 percent was attributed to increased yield and only 15 percent to increased percentage in the milk. Emery, (1978) reported that milk protein percentage increases 0.015 percent for each Meal of additional net energy fed from 9 to 40 Meal/day and that the increased protein percentage was usually accompanied by an increased milk yield. Mild energy malnutrition has been reported to slightly reduce milk protein percentage; however, under severe energy malnutrition, milk protein percentage is unaltered but yields decrease drastically (Thomas, 1980, 1983).
Rogers and Stewart (1982) reviewed the effects of various forage sources in the diet on milk composition. Cows grazing early spring pastures were reported to have increased milk protein percentages. However, the confounding of energy, protein, and condition of the cow in most forage studies where milk composition is reported prohibits the drawing of definite conclusions.
Thomas (1980, 1983) discussed the notion that increasing propionic acid in the rumen through increased concentrate feeding or reduced forage particle size affects milk protein percentage. To summarize, there is a strong positive correlation between rumen production of propionic acid and milk protein; however, the exact mechanism is unknown. One suggestion is that propionate increases glutamic acid availability to the mammary gland and, through its role in amino acid transamination, enhances synthesis of nonessential amino acids. A second hypothesis is that propionate through insulin could enhance plasma concentrations of glutamine and alanine. Propionate could also enhance glutamate output from the liver by increasing its synthesis or reducing usage in gluconeogenesis.
Intake of energy can also be increased through inclusion of fats or oils in the diet. Feeding protected lipids, vegetable fats, or vegetable oils to lactating cows depressed milk protein percentage, whereas animal fats had no effect or minimal effect on milk protein percentages (Linn, 1983; Palmquist and Jenkins, 1980). Dunkley et al. (1977) indicated that the depressing effect was on the casein fraction. Although the exact depressing mechanism is unknown, it may be through altered glucose metabolism (Palmquist and Jenkins, 1980), changes in rumen metabolism (Jenkins and Palmquist, 1984), or both. Thus, the source of increased dietary energy (carbohydrate versus lipid) fed to lactating cows has a significant effect on milk protein percentage changes.
Carbohydrates
The predominant carbohydrate in milk is the disaccharide lactose. It is composed of one molecule of glucose and one molecule of galactose joined in a 1-4 carbon linkage as beta-galactoside. The principal biological function of lactose in milk is the regulation of water content and, thus, the regulation of osmotic content (Davies et al., 1983; Jenness, 1985). Because of this function, lactose is the most constant constituent in milk, averaging 4.6 percent.
Carbohydrates other than lactose that are found in milk are monosaccharides, sugar phosphates, nucleotide sugars, free neutral and acid oligosaccharides, and glycosyl groups of peptides and proteins (Jenness, 1985). Free glucose and galactose and the sugar alcohol myo-inositol are also present in milk. However, the amounts of these carbohydrate fractions are minor compared with that of lactose.
Biosynthesis
Glucose is the primary substrate for lactose synthesis, with 85 percent of the carbon secreted in lactose derived from blood glucose (Thomas and Chamberlain, 1984). Lactose synthesis is initiated in the Golgi apparatus and continues in the vesicles with an influx of water and ionic constituents that causes the vesicles to swell as they pass toward the cell surface. Glucose and uridine diphosphate (UDP)-galactose, derived from glucose, combine to form lactose under the action of the enzyme lactose synthetase. The milk protein beta-lactalbumin must be present for glucose and UDP-galactose to combine. Thus, beta-lactalbumin appears to be a prime regulator of lactose synthesis (Kuhn, 1983; Larson, 1985). Entry of water into the vesicle is linked with lactose synthesis to maintain osmotic equilibrium with surrounding fluids. Thus, the rate of lactose synthesis regulates water secretion and consequently milk yield.
Minerals
Factors Affecting Milk Mineral Content
The mineral content of milk is derived from minerals found in circulating body fluids. The factors influencing mineral content of milk are discussed below.
Breed/Genetics
Cerbulis and Farrel (1976) reported the ash, calcium, phosphorus, and magnesium contents of milk from different breeds of dairy cattle. The average ash content varied from 0.74 percent for Holsteins to 0.83 percent for Jerseys. The highest calcium and phosphorus contents in milk were reported for Jerseys.
Environment/Management
It is well documented that the mineral composition of colostrum is higher than that of milk. Calcium, phosphorus, potassium, and chloride concentrations follow the same lactation curves as fat and protein—that is, high in colostrum, lowest at peak milk yield, and then gradually increasing as lactation progresses (Iyengar, 1982; Jenness, 1985). Milk inorganic phosphorus levels were shown to be higher in first lactation cows than in multiparous cows, and milk phosphate levels were lowest during the summer (Forar et al., 1982).
Health/Physiology
Mastitis increases the percentages of sodium and chloride in milk and decreases the percentage of potassium (Kitchen, 1981; Peaker and Faulkner, 1983; Schultz, 1977). Bacterial infection of the udder results in damage to the ductal and secretory epithelium and increases the permeability of blood capillaries. Thus, sodium and chloride, which are higher in blood, pour into the lumen of the alveolus, and in order to maintain osmolarity, potassium is decreased proportionally. Fernando et al. (1985) reported the decline in lactose and potassium and increase in sodium and chloride in mastitic milk was most prominent in strippings of mink after milking.
The percentages of calcium and phosphorus in milk decline with mastitis infections (Kitchen, 1981; Schultz, 1977). Most likely this reflects lower casein levels, since both ions are complexed with casein micelles. Contradictory evidence exists regarding the effect of mastitic infections on levels of magnesium. Trace elements may increase slightly in mastitic milk (Tallamy and Randolph, 1970).
Administration of exogenous growth hormone has relatively little effect on the percentages of minerals in milk, but yields of minerals increased with increasing milk production (Eppard et al., 1985).
Nutrition
Normal dietary regimes have little influence on the mineral composition of milk, especially the macromineral constituents. Forar et al. (1982) fed two levels of phosphorus (0.31 and 0.54 percent) and two levels of calcium (1.0 and 1.8 percent) in four diets to lactating cows and found no differences in milk inorganic phosphorus percentages. Diets depressing milk fat percentage have been shown to lower the percentage of citrate and soluble calcium in milk (Davies et al., 1983). Changes in milk phosphorus and calcium percentages would not be expected, since very few of these ions are in the free form in milk. Dietary factors affecting citrate and casein contents of milk would be expected to correspond with small changes in calcium, since calcium is complexed and secreted with these substances.
Fettman et al. (1984) observed decreases in milk chloride percentage when cows were fed chloride-deficient rations during early lactation. Milk potassium percentage declined along with chloride levels, reflecting altered mineral metabolism in chloride-de-ficient cows. A recent report (Schneider et al., 1986) evaluating dietary sodium and potassium effects on heat-stressed cows found no change in milk potassium percentages based on quantity of potassium fed or source of sodium fed. However, cows offered shade had higher milk potassium percentages than cows given no shade. Percentages of sodium in milk were lowered significantly by feeding cows sodium bicarbonate and only slightly by feeding salt or high levels of potassium, as compared with results for control cows. The lower milk sodium percentages corresponded with lower plasma sodium levels in cows fed sodium bicarbonate.
Milk iodine levels have been shown to increase with increased feeding of iodine. Franke et al. (1983) observed progressive increases in milk iodine concentrations during lactation when as little as 4 ppm of organic iodine were added to the diet. Larson et al. (1983) found cow age, season of calving, milk production, and health status to have no effect on the concentration of iodine in milk.
Iyengar (1982) reported that iodine, manganese, molybdenum, selenium, zinc, and cobalt concentrations in milk could be altered by dietary means. However, very limited research has been directed toward this end. Most of the changes that have been observed are the result of marked dietary, changes. The effects of slightly underfeeding or overfeeding a required dietary mineral or the effects of mineral interactions on the mineral composition of milk are not well known.
Other Milk Solubles
There are many other components in milk in addition to those already discussed. They can be categorized as either natural or contaminant. They appear in milk both from leakage during the normal secretory process and by actual secretion. Whatever the mode of entry, their concentrations can vary considerably, but their significance and purpose remain largely unknown.
The natural compounds that have been detected in milk are gases, alcohol, aldehydes, ketones, carboxylic acid, sulfur-containing compounds, nucleotide material, hormones, phosphate esters, glucose, acetate, and citrate. Many of these are products of intermediary metabolism of the mammary gland (Davies et al., 1983; Jenness, 1985; Peaker and Faulkner, 1983). The reasons for changes in the concentrations of these compounds in milk are unknown.
An exception to the above is the compound citrate. The concentration of citrate in milk is modifiable and is important from a milk-processing standpoint. Alteration of citrate concentrations changes the amount of free calcium in the soluble phase of milk, which, in turn, affects the precipitation of milk proteins. Milk citrate concentrations are highly correlated with fat percentage, and therefore diets that lower milk fat percentage also decrease the citrate content of milk (Faulkner and Peaker, 1982). Stage of lactation and season of the year also affect milk citrate levels. It appears that the source of milk citrate is from synthesis within secretory cells and that secretion into milk is similar to that of lactose and casein (Faulkner and Peaker, 1982).
The other category of components—contaminants-includes compounds that are not normally found in milk but that enter accidentally or by design. Included here are chemicals, pesticides, herbicides, fungicides, heavy metals, and drugs. These items are mentioned as a reminder that milk can contain compounds other than those of nutritional importance to humans and that maintaining a nutritious, wholesome milk supply is of utmost importance.
Manipulating Milk Constituents—Summary and Conclusions
Variations in milk composition arise from differences in relative rates of synthesis and secretion of milk components by the mammary gland. The processes involved for lactose, protein, and fat synthesis and secretion are independent but regulated through nutrient or substrate availability and hormonal control of nutrient utilization. Thus, genetics, which mediates hormonal effects, and diet, which regulates nutrient availability, are the major factors affecting milk composition.
The most variable milk constituent is fat. Considerable variation exists between and within dairy cattle breeds. Genetic selection for fat percentage can change fat content of milk but will also affect other constituents since there is a high correlation between the percentage of components in milk. Genetic selection for fat content would alter the quantity of fat produced but not the composition of the fat. The best hope for altering composition is through diet. Changes in fat percentage and composition can be accomplished by altering the diet to produce changes in fermentation patterns or the composition of fat absorbed from the digestive tract. Diets that increase the proportion of propionate in the rumen depress milk fat percentage, but changes in fat composition are minimal, including slight increases in C18 polyunsaturated fatty acids and slight decreases in C160 and C180 fatty acids. Inclusion of fats in the diet, particularly rumen-protected fats, is the most effective way to alter milk fat composition. Significant increases in long-chain fatty acids can be achieved by including fat containing these acids in the diet. However, the amount and composition of fats in the diet need to be controlled to avoid impairment of digestion of other dietary constituents in the rumen. Unsaturated fatty acids are hydrogenated extensively in the rumen.
Milk protein percentage and composition can be manipulated through genetic selection. Variations in the casein, beta-lactoglobulin, and alpha-lactalbumin fractions are known to exist. Heritability estimates of protein percentage range from 0.3 to 0.7. Increasing milk protein percentage through genetic selection is feasible; however, increasing milk protein yield through selection is more desirable. The percentage of true proteins in milk cannot be manipulated through feeding. Total protein percentage in milk can be lowered by including fats in the diet or raised in relation to milk fat percentage by feeding high-concentrate diets. Dietary protein percentage has a minimal effect on milk protein percentage when it is within practical feeding ranges.
Levels of other nutritional components of milk—lactose, vitamins, and minerals—are rather constant and not subject to large changes through genetic or nutritional manipulation.
The manipulation of milk components through changes in dais, management practices, breeding, feeding, health, environment, and general management appears to be rather limited. Milk fat percentage and composition can be changed through feeding, whereas milk protein percentage is best changed through genetics. Any changes will be slow in coming and minor compared to those achieved through processing and manufacturing. The goal of milk producers should be to modify composition as much as possible to meet market demand but to emphasize maximum yield of components in high-quality, wholesome milk.
References
- Allen, D. B., E. J. DePeters, and R. C. Laben. 1986. Three times a day milking. Effects on milk production, reproductive efficiency and udder health. J. Dairy Sci. 69:1441. [PubMed: 3722553]
- Amos, H. E., T. Kiser, and M. Loewenstein. 1985. Influence of milking frequency on reproductive and productive efficiencies of cows. J. Dairy Sci. 68:732. [PubMed: 3989091]
- Banks, W., J. L. Clapperton, and W. Steele. 1983. Dietary manipulation of the content and fatty acid composition of milk fat. Proc. Nutr. Soc. 42:399. [PubMed: 6361769]
- Bauman, D. E., and J. M. Elliot. 1983. Control of nutrient partitioning in lactating ruminants. Ch. 14 in Biochemistry of Lactation, T. B. Mepham, editor. , ed. Amsterdam: Elsevier.
- Bauman, D. E., P. J. Eppard, M. J. DeGeeter, and G. M. Lanza. 1985. Responses of high-producing dairy cows to long term treatment with pituitary somatotropin and recombinant somatotropin. J. Dairy Sci. 68:1352. [PubMed: 4019880]
- Baumrucker, C. R. 1985. Amino acid transport systems in bovine mammary tissue. J. Dairy Sci. 68:2436. [PubMed: 2866205]
- Bragg, D. St. A., M. R. Murphy, and C. L. Davis. 1986. Effect of source of carbohydrate and frequency of feeding on rumen parameters in dairy steers. J. Dairy Sci. 69:392. [PubMed: 3700791]
- Casper, D. P., and D. J. Schingoethe. 1986. Evaluation of urea and dried whey in diets of cows during early lactation. J. Dairy Sci. 69:1346. [PubMed: 3487561]
- Cerbulis, J., and H. M. Farrell, Jr. 1976. Composition of the milk of dairy cattle. II. Ash, calcium, magnesium and phosphorus J. Dairy Sci. 59:589. [PubMed: 1262573]
- Chalupa, W, and P. L. Schneider. 1985. Buffers for dairy cattle. 20th Annual Proceedings of the 1985 Pacific Northwest Animal Nutrition Conference, sponsored by the Pacific Northwest Feed Manufacturers. October 1985, Boise, Idaho.
- Christie, W. W. 1979. The effects of diet and other factors on the lipid composition of ruminant tissues and milk. Prog. Lipid Res. 17:245. [PubMed: 370841]
- Clark, J. H. 1975. Lactational responses to post-ruminal administration of proteins and amino acids. J. Dairy Sci. 58:1178. [PubMed: 1099124]
- Clark, J. H., H. R. Spires, R. G. Derrig, and M. R. Bennik. 1977. Milk production, nitrogen utilization and glucose synthesis in lactating cows infused post-ruminally with sodium caseinate and glucose. J. Nutr. 107:631. [PubMed: 845699]
- Clark, J. H., H. R. Spires, and C. L. Davis. 1978. Uptake and metabolism of nitrogenous compounds by the lactating mammary gland. Fed. Proc. 37:1233. [PubMed: 640005]
- Coppock, C. E. 1985. Energy nutrition and metabolism of the lactating cow. J. Dairy Sci. 68:3403.
- Cragle, R. G., M. R. Murphy, S. W. Williams, and J. H. Clark. 1986. Effects of altering milk production and composition by feeding on multiple component milk pricing system. J. Dairy Sci. 69:282.
- Crawford, R. J., Jr., and W. H. Hoover. 1984. Effects of particle size and formaldehyde treatment of soybean meal on milk production and composition for dairy cows. J. Dairy Sci. 67:1945. [PubMed: 6541665]
- Crooker, B. A., J. H. Clark, and R. D. Shanks. 1983. Effects of formaldehyde treated soybean meal on milk yield, milk composition and nutrient digestibility in the dairy cow. J. Dairy Sci. 66:492. [PubMed: 6682428]
- Davies, D. T., C. Holt, and W. W. Christie. 1983. The composition of milk. Ch. 5 in Biochemistry of Lactation, T. B. Mepham, editor. , ed. Amsterdam: Elsevier.
- Davis, C. L. 1978. The use of buffers in the rations of lactating dairy cows. In Proceedings of the Regulation of Acid-Base Balance, sponsored by the University of Arizona and Church and Dwight Co., Inc., W. H. Hale, editor; and P. Meinhardt, editor. , eds. Tuscon: University of Arizona.
- DePeters, E. J. and S. J. Taylor. 1985. Effects of feeding corn or barley on composition of milk and diet digestibility. J. Dairy Sci. 68:2027. [PubMed: 4044966]
- DePeters, E. J., N. E. Smith, and J. Acedo-Rico. 1985. Three or two times daily milking of older cows and first lactation cows for entire lactations. J. Dairy Sci. 68:123. [PubMed: 3980809]
- Dils, R. R. 1983. Milk fat synthesis. Ch. 5 in Biochemistry of Lactation, T. B. Mepham, editor. , ed. Amsterdam: Elsevier.
- Dils, R. R. 1986. Comparative aspects of milk fat synthesis. J. Dairy Sci. 69:904. [PubMed: 3711414]
- Dunkley, W. L., N. E. Smith, and A. A. Franke. 1977. Effects of feeding protected tallow on composition of milk and milk fat. J. Dairy Sci. 60:1863. [PubMed: 599201]
- Eigel, W. N., J. E. Butler, C. A. Ernstrom, H. M. Farrell, Jr, V. R. Harwalker, R. Jenness, and R. McL. Whitney. 1984. Nomenclature of proteins of cow's milk: Fifth revision. J. Dairy Sci. 67:1599.
- Emery, R. S. 1978. Feeding for increased milk protein. J. Dairy Sci. 61:825.
- Eppard, P. J., D. E. Bauman, J. Bitman, D. L. Wood, R. M. Akers, and W. A. House. 1985. Effect of dose of bovine growth hormone on milk composition Alpha-lactalbumin, fatty acids, and mineral elements. J. Dairy Sci. 68:3047. [PubMed: 4078130]
- Faulkner, A, and M. Peaker. 1982. Secretion of citrate into milk. J. Dairy Res. 49:159. [PubMed: 7042779]
- Fernando, R. S., S. L. Spahr, and E. H. Jaster. 1985. Comparison of electrical conductivity of milk with other indirect methods for detection of subclinical mastitis. J. Dairy Sci. 68:449. [PubMed: 3989085]
- Fettman, M J, L. E. Chase, J. Bentinck-Smith, C. E. Coppock, and S. A. Zinn. 1984. Nutritional chloride deficiency in early lactation Holstein cows. J. Dairy Sci. 67:2321. [PubMed: 6501651]
- Fogerty, A. C., and A. R. Johnson. 1980. Influence of nutritional factors on the yield and content of milk fat: Protected polyunsaturated fat in the diet. Int. Dairy Fed. Bull. Doc. 125:96.
- Forar, F. L., R. L. Kincaid, R. L. Preston, and J. K. Hillers. 1982. Variation of inorganic phosphate in blood plasma and milk lactating cows. J. Dairy Sci. 65:760. [PubMed: 7202021]
- Forester, R. J., D. G. Grieve, J. G. Buchanan-Smith, and G. K. MacLeod. 1983. Effect of dietary protein degradability on cows in early lactation. J. Dairy Sci. 66:1653.
- Franke, A. A., J. C. Bruhn, and R. B. Osland. 1983. Factors affecting iodine concentration of milk of individual cows. J. Dairy Sci. 66:997. [PubMed: 6875067]
- Gaunt, S. N. 1973. Genetic and environmental changes in milk consumption. J. Dairy Sci. 56:270.
- Gaunt, S. N. 1980. Genetic variation in the yields and contents of milk constituents. Int. Dairy Fed. Bull. Doc. 125:73.
- Gisi, D. D., E. J. DePeters, and C. L. Pelissier. 1986. Three times daily milking of cows in California dairy herds. J. Dairy Sci. 69:863.
- Grappin, R., V. S. Packard, and R. E. Ginn. 1981. Variability and interrelationship of various herd milk components. J. Food Prot. 44:69. [PubMed: 30836469]
- Hansen, W. P., D. E. Otterby, J. D. Donker, R. G. Lundquist, and J. G. Linn. 1984. Influence of grain concentrations, forage type, and methionine hydroxy analog on lactational performance of dairy cattle. J. Dairy Sci. 67(Suppl. 1):99 (Abstr.).
- Henderson, S. J., H. E. Amos, and J. J. Evans. 1985. Influence of dietary crude protein concentration and degradability on milk production, composition, and ruminal protein metabolism. J. Dairy Sci. 68:2227.
- Holter, J. B., W. E. Urban, Jr., H. H. Hayes, and H. A. Davis. 1977. Utilization of diet components fed blended or separately to lactating cows. J. Dairy Sci. 60:1288.
- Holter, J. B., W. E. Hylton, and C. K. Bozak. 1985. Varying protein content and nitrogen solubility for pluriparious, lactating Holstein cows: Lactation performance and profitability. J. Dairy Sci. 68:1984. [PubMed: 4044963]
- Iyengar, G. V. 1982. Elemental Composition of Human and Animal Milk: A Review. International Atomic Energy Agency Technical Document 269. Vienna: International Atomic Energy Agency.
- Jenkins, T. C., and D. L. Palmquist. 1984. Effect of fatty acids of calcium soaps on rumen and total nutrient digestibility of dairy rations. J. Dairy Sci. 67:978. [PubMed: 6330189]
- Jenness, R. 1985. Biochemical and nutritional aspects of milk and colostrum. Ch. 5 in Lactation, B. L. Larson, editor. , ed. Ames: Iowa State University Press.
- Kaufman, W. 1980. Protein degradation and synthesis within the reticulorumen in relation to milk protein synthesis. Intl. Dairy Fed. Bull. Doe. 125:152.
- Kawas, J. R., N. A. Jorgensen, A. R. Hardie, and J. L. Danelon. 1983. Change in feeding value of alfalfa hay with stage of maturity and concentrate level. J. Dairy Sci. 66:181 (Abstr.).
- Keown, J. F., R. W. Everett, N. B. Empet, and L. H. Wadell. 1986. Lactation curves. J. Dairy Sci. 69:769.
- Kitchen, B. J. 1981. Bovine mastitis: Milk compositional changes and related diagnostic tests. J. Dairy Res. 48:167. [PubMed: 7021617]
- Kroeker, E. M., K. F. Ng-Kwai-Hang, J. F. Hayes, and J. E. Moxley. 1985. Effect of beta-lactoglobulin variant and environmental factors on variation in the detailed composition of bovine milk serum proteins. J. Dairy Sci. 68:1637.
- Kuhn, N. J. 1983. The biosynthesis of lactose. Ch. 6 in Biochemistry of Lactation, T. B. Mepham, editor. , ed. Amsterdam. Elsevier.
- Kung, L., Jr., and J. T. Huber. 1983. Performance of high producing cows in early lactation fed protein of varying amounts, sources, and degradability. J. Dairy Sci. 66:227. [PubMed: 6682122]
- Knzdzal-Savoie, S., W. Manson, and J. H. Moore. 1980. The constituents of cow's milk. Int. Dairy Fed. Bull. Doc. 125:4-13.
- Larson, B. L. 1979. Biosynthesis and secretion of milk protein: A review. J. Dairy Res. 46:161. [PubMed: 381339]
- Larson, B. L. 1985. Biosynthesis and cellular secretion of milk. Ch. 4 in Lactation, B. L. Larson, editor. , ed. Ames: Iowa State University Press.
- Larson, L. L., S. E. Wallen, F. G. Owen, and S. R. Lowry. 1983. Relation of age, season, production and heath indices to iodine and beta-carotene concentrations in cow's milk. J. Dairy Sci. 66:2257. [PubMed: 6668379]
- Linn, J. G. 1983. The addition of fats to diets of lactating dairy cows: A review. In Proceedings of a Feed Fat Seminar, sponsored by Central Bi-Products. Redwood Falls, Minn.
- Linn, J. G., and D. E. Otterby. 1984. Feeding strategies in dairy nutrition. Pp. 13-22 in Proceedings of the 45th Minnesota Nutrition Conference. St. Paul, Minn.: University of Minnesota Press.
- Lundquist, R. L., J. G. Linn, and D. E. Otterby. 1983. Influence of dietary, energy and protein on yield and composition of milk from cows fed methionine hydroxy analog. J. Dairy Sci. 66:475. [PubMed: 6841748]
- Lundquist, R. L., D. E Otterby, and J. G. Linn. 1986. Influence of formaldehyde-treated soybean meal on milk production. J. Dairy, Sci. 69:1337. [PubMed: 3722547]
- Madsen, J. 1982. The effect of formaldehyde-treated protein and urea on milk yield and composition in dairy cows. Acta Agric. Scand. 32:389.
- Marshall, S. P., and A. R. Voigt. 1975. Complete rations for dairy cattle. I. Methods of preparation and roughage-to-concentrate ratios of blended rations with corn silage. J. Dairy, Sci. 58:891.
- Mepham, T. B. 1982. Amino acid utilization by lactating mammary gland. J. Dairy Sci. 65:287. [PubMed: 7042785]
- Mercier, J.-C., and P. Gaye. 1983. Milk protein synthesis. Ch. 7 in Biochemistry of Lactation, T. B. Mepham, editor. , ed. Amsterdam: Elsevier.
- Mertens, D. R. 1985. Effect of fiber on feed quality for dairy cows. Pp. 209-224 in Proceedings of the 46th Minnesota Nutrition Conference. St. Paul, Minn.: University of Minnesota Press.
- Milam, K. Z., C. E. Coppock, J. W. West, J. K. Lanham, D. H. Nave, J. M. Labore, R. A. Stermer, and C. F. Brasington. 1986. Effects of drinking water temperature on production responses m lactating Holstein cows in summer. J. Dairy Sci. 69:1013. [PubMed: 3722525]
- Needs, E. C., and M. Anderson. 1984. Lipid composition of milk from cows with experimentally induced mastitis. J. Dairy Res. 51:239. [PubMed: 6373863]
- Ng-Kwai-Hang, K. F., J. F. Hayes, J. E. Moxley, and H. G. Monardes. 1982. Environmental influences on protein content and composition of bovine milk. J. Dairy Sci. 65:1993. [PubMed: 6890960]
- Ng-Kwai-Hang, K. F., J. F. Hayes, J. E. Moxley, and H. G. Monardes. 1985. Percentages of protein and nonprotein nitrogen with varying fat and somatic cells in bovine milk. J. Dairy Sci. 68:1257. [PubMed: 3842864]
- Oldham, J. D. 1984. Amino acid metabolism in ruminants. Pp. 137-151 in Proceedings of the Cornell Nutrition Conference, sponsored by Cornell University and American Feed Manufacturers Association. Ithaca, N.Y., Cornell University.
- Oltner, R., M. Emanuelson, and H. Wiktorsson. 1985. Urea concentrations in milk in relation to milk yield, live weight, lactation number and amount and composition of feed given to dairy cows. Livestock Prod. Sci. 12:47.
- Owen, J. B. 1981. Complete-diet feeding of dairy cows. In Recent Development in Ruminant Nutrition, W. Haresign, editor; and D. J. A. Cole, editor. , eds. London: Butterworth.
- Palmquist, D. L., and T. C. Jenkins. 1980. Fat in lactation rations: Review. J. Dairy Sci. 63:1. [PubMed: 6989864]
- Peaker, M., and A. Faulkner. 1983. Soluble milk constituents. Proc. Nutr. Soc. 42:419. [PubMed: 6361771]
- Peel, C. J., L. D. Sandles, K. J. Quelch, and A. C. Herington. 1985. The effects of long-term administration of bovine growth hormone on the lactational performance of identical-twin dairy cows. Anim. Prod. 41:135.
- Poutrel, B., J. P. Caffin, and P. Rainard. 1983. Physiological and pathological factors influencing bovine serum albumin content in milk. J. Dairy Sci. 66:535. [PubMed: 6404972]
- Rogers, G. L., and J. A. Stewart. 1982. The effects of some nutritional and nonnutritional factors on milk protein concentration and yield. Aust. J. Dairy Technol. 37:26.
- Rolleri, G. D., B. L. Larson, and R. W. Touchberry. 1956. Protein production in the bovine. Breed and individual variations in the specific protein constituents of milk. J. Dairy Sci. 39:1683.
- Rook, J. A. F., and P. C. Thomas. 1980. Principles involved in manipulating the yields and concentrations of constituents in milk. Int. Dairy Fed. Bull. Doc. 125:66.
- Schingoethe, D. J. 1976. Whey utilization in animal feeding: A summary and evaluation. J. Dairy Sci. 59:556.
- Schneider, P. L., D. K. Beede, and C. J. Wilcox. 1986. Responses of lactating cows to dietary sodium source and quantity and potassium quantity during heat stress. J. Dairy Sci. 69:99. [PubMed: 3009576]
- Schultz, L. H. 1977. Somatic cell in milk—physiological aspects and relationship to amount and composition of milk. J. Food Prot. 40:125. [PubMed: 30731547]
- Schwab, C. G., L. D. Satter, and A. B. Clay. 1976. Response of lactating cows to abomasal infusions of amino acids. J. Dairy Sci. 59:1254. [PubMed: 950397]
- Shaver, R. D., A. J. Nytes, L. D. Satter, and N. A. Jorgensen. 1986. Influence of amount of feed intake and forage physical form on digestion and passage of prebloom alfalfa hay in dairy cows. J. Dairy Sci. 69:1545.
- Storry, J. E. 1980. Influence of nutritional factors on the yield and content of milk: Nonprotected fat in the diet. Int. Dairy Fed. Bull. Doc. 125:88.
- Storry, J. E., and P. E. Brumby. 1980. Influence of nutritional factors on yield and content of milk: Protected nonpolyunsaturated fat in the diet. Int. Dairy Fed. Bull. Doc. 125:105.
- Sutton, J. D. 1980. Influence of nutritional factors on the yield and content of milk fat: Dietary components other than fat. Int. Dairy Fed. Bull. Doc. 125:126.
- Sutton J. D. 1985. Digestion and absorption of energy substrates in the lactating cow. J. Dairy Sci. 68:3376.
- Tallamy, P. T., and H. E. Randolph. 1970. Influence of mastitis on properties of milk. V. Total and free concentrations of major minerals in skim milk. J. Dairy Sci. 53:1386. [PubMed: 5528758]
- Thomas, P. C. 1980. Influence of nutrition on the yield and content of protein in milk. Dietary protein and energy supply. Int. Dairy Fed. Bull. Doc. 125:142.
- Thomas, P. C., and D. G. Chamberlain. 1984. Manipulation of milk composition to meet market needs. Ch. 14 in Recent Advances in Animal Nutrition, W. Haresign, editor; and D. J. A. Cole, editor. , eds. London: Butterworth.
- Tucker, H. A. 1985. Endocrine and neural control of the mammary gland. Ch. 2 in Lactation, B. L. Larson, editor. , ed. Ames: Iowa State University Press.
- Van Vleck, L. D. 1978. Breeding for increased milk protein. J. Dairy Sci. 61:815.
- Wheelock, J. V. 1980. Influence of physiological factors on the yields and contents of milk constituents. Int. Dairy Fed. Bull. Doc. 125:83.
- Wilcox, C. J. 1978. Genetic considerations of economic importance: Milk yield, composition and quality. Ch. 2 in Large Dairy Herd Management, C. J. Wilcox, editor; , H. H. Van Horn, editor; , B. Harris, Jr., editor; , H. H. Head, editor; , S. P. Marshall, editor; , W. W. Thatcher, editor; , D. W. Webb, editor; , and J. M. Wing, editor. , eds. Gainesville: University Presses of Florida.
- Wolfschoon-Pombo, A., and H. Klostermeyer. 1981. The NPN-fraction of cow milk. I. Amount and composition. Milchwissenschaft 36:598.
- Woodford, J. A., N. A. Jorgensen, and G. P. Barrington. 1986. Impact of dietary fiber and physical form on performance of lactating dairy cows. J. Dairy Sci. 69:1035. [PubMed: 3013961]
- Young, C. W., J. K. Hillers, and A. E. Freeman. 1986. Production, consumption, and pricing of milk and its components. J. Dairy Sci. 69:272.
- PubMedLinks to PubMed
- Factors Affecting the Composition of Milk from Dairy Cows - Designing FoodsFactors Affecting the Composition of Milk from Dairy Cows - Designing Foods
- ISOPROPYL ALCOHOL - Emergency and Continuous Exposure Limits for Selected Airbor...ISOPROPYL ALCOHOL - Emergency and Continuous Exposure Limits for Selected Airborne Contaminants
- Chain A, PROTEIN (CHALCONE SYNTHASE)Chain A, PROTEIN (CHALCONE SYNTHASE)gi|5821988|pdb|1CGZ|AProtein
Your browsing activity is empty.
Activity recording is turned off.
See more...