NCBI Bookshelf. A service of the National Library of Medicine, National Institutes of Health.
National Institutes of Health (US); Biological Sciences Curriculum Study. NIH Curriculum Supplement Series [Internet]. Bethesda (MD): National Institutes of Health (US); 2007.
In simple terms, cancer is a group of more than 100 diseases that develop across time and involve the uncontrolled division of the body's cells. Although cancer can develop in virtually any of the body's tissues, and each type of cancer has its unique features, the basic processes that produce cancer are quite similar in all forms of the disease.
Cancer begins when a cell breaks free from the normal restraints on cell division and begins to follow its own agenda for proliferation (Figure 3). All of the cells produced by division of this first, ancestral cell and its progeny also display inappropriate proliferation. A tumor, or mass of cells, formed of these abnormal cells may remain within the tissue in which it originated (a condition called in situ cancer), or it may begin to invade nearby tissues (a condition called invasive cancer). An invasive tumor is said to be malignant, and cells shed into the blood or lymph from a malignant tumor are likely to establish new tumors (metastases) throughout the body. Tumors threaten an individual's life when their growth disrupts the tissues and organs needed for survival.
What happens to cause a cell to become cancerous? Thirty years ago, scientists could not offer a coherent answer to this question. They knew that cancer arose from cells that began to proliferate uncontrollably within the body, and they knew that chemicals, radiation, and viruses could trigger this change. But exactly how it happened was a mystery.
Research across the last three decades, however, has revolutionized our understanding of cancer. In large part, this success was made possible by the development and application of the techniques of molecular biology, techniques that enabled researchers to probe and describe features of individual cells in ways unimaginable a century ago. Today, we know that cancer is a disease of molecules and genes, and we even know many of the molecules and genes involved. In fact, our increasing understanding of these genes is making possible the development of exciting new strategies for avoiding, forestalling, and even correcting the changes that lead to cancer.
Unraveling the Mystery of Cancer
People likely have wondered about the cause of cancer for centuries. Its name derives from an observation by Hippocrates more than 2,300 years ago that the long, distended veins that radiate out from some breast tumors look like the limbs of a crab. From that observation came the term karkinoma in Greek, and later, cancer in Latin.
With the work of Hooke in the 1600s, and then Virchow in the 1800s, came the understanding that living tissues are composed of cells, and that all cells arise as direct descendants of other cells. Yet, this understanding raised more questions about cancer than it answered. Now scientists began to ask from what kinds of normal cells cancer cells arise, how cancer cells differ from their normal counterparts, and what events promote the proliferation of these abnormal cells. And physicians began to ask how cancer could be prevented or cured.
Clues from epidemiology
One of the most important early observations that people made about cancer was that its incidence varies between different populations. For example, in 1775, an extraordinarily high incidence of scrotal cancer was described among men who worked as chimney sweeps as boys. In the mid-1800s, lung cancer was observed at alarmingly high rates among pitch blende miners in Germany. And by the end of the 19th century, using snuff and cigars was thought by some physicians to be closely associated with cancers of the mouth and throat.
These observations and others suggested that the origin or causes of cancer may lie outside the body and, more important, that cancer could be linked to identifiable and even preventable causes. These ideas led to a widespread search for agents that might cause cancer. One early notion, prompted by the discovery that bacteria cause a variety of important human diseases, was that cancer is an infectious disease. Another idea was that cancer arises from the chronic irritation of tissues. This view received strong support with the discovery of X-rays in 1895 and the observation that exposure to this form of radiation could induce localized tissue damage, which could lead in turn to the development of cancer. A conflicting view, prompted by the observation that cancer sometimes seems to run in families, was that cancer is hereditary.
Such explanations, based as they were on fragmentary evidence and incomplete understanding, helped create the very considerable confusion about cancer that existed among scientists well into the mid-twentieth century. The obvious question facing researchers—and no one could seem to answer it—was how agents as diverse as this could all cause cancer. Far from bringing science closer to understanding cancer, each new observation seemed to add to the confusion.
Yet each new observation also, ultimately, contributed to scientists' eventual understanding of the disease. For example, the discovery in 1910 that a defined, submicroscopic agent isolated from a chicken tumor could induce new tumors in healthy chickens showed that a tumor could be traced simply and definitively back to a single cause. Today, scientists know this agent as Roussarcoma virus, one of several viruses that can act as causative factors in the development of cancer.
Although cancer-causing viruses are not prime agents in promoting most human cancers, their intensive study focused researchers' attention on cellular genes as playing a central role in the development of the disease.
Likewise, investigations into the association between cancer and tissue damage, particularly that induced by radiation, revealed that while visible damage sometimes occurs, something more subtle happens in cells exposed to cancer-causing agents. One clue to what happens came from the work of Herman Muller, who noticed in 1927 that X-irradiation of fruit flies often resulted in mutant offspring. Might the two known effects of X-rays, promotion of cancer and genetic mutation, be related to one another? And might chemical carcinogens induce cancer through a similar ability to damage genes?
Support for this idea came from the work of Bruce Ames and others who showed in 1975 that com pounds known to be potent carcinogens (cancer-causing agents) generally also were potent mutagens (mutation-inducing agents), and that compounds known to be only weak carcinogens were only weak mutagens. Although scientists know today that many chemicals do not follow this correlation precisely, this initial, dramatic association between mutagenicity and carcinogenicity had widespread influence on the development of a unified view of the origin and development of cancer.
Finally, a simple genetic model, proposed by Alfred Knudson in 1971, provided both a compelling explanation for the origins of retinoblastoma, a rare tumor that occurs early in life, and a convincing way to reconcile the view of cancer as a disease produced by external agents that damage cells with the observation that some cancers run in families. Knudson's model states that children with sporadic retinoblastoma (children whose parents have no history of the disease) are genetically normal at the moment of conception, but experience two somatic mutations that lead to the development of an eye tumor. Children with familial retinoblastoma (children whose parents have a history of the disease) already carry one mutation at conception and thus must experience only one more mutation to reach the doubly mutated configuration required for a tumor to form. In effect, in familial retinoblastoma, each retinal cell is already primed for tumor development, needing only a second mutational event to trigger the cancerous state. The difference in probabilities between the requirement for one or two mutational events, happening randomly, explains why in sporadic retinoblastoma, the affected children have only one tumor focus, in one eye, while in familial retinoblastoma, the affected children usually have multiple tumor foci growing in both eyes.
Although it was years before Knudson's explanation was confirmed, it had great impact on scientists' understanding of cancer. Retinoblastoma, and by extension, other familial tumors, appeared to be linked to the inheritance of mutated versions of growth-suppressing genes. This idea led to the notion that cells in sporadically arising tumors might also have experienced damage to these critical genes as the cells moved along the path from the normal to the cancerous state.
Clues from cell biology
Another field of study that contributed to scientists' growing understanding of cancer was cell biology. Cell biologists studied the characteristics of cancer cells, through observations in the laboratory and by inferences from their appearance in the whole organism. Not unexpectedly, these investigations yielded a wealth of information about normal cellular processes. But they also led to several key understandings about cancer, understandings that ultimately allowed scientists to construct a unified view of the disease.
One such understanding is that cancer cells are indigenous cells—abnormal cells that arise from the body's normal tissues. Furthermore, virtually all malignant tumors are monoclonal in origin, that is, derived from a single ancestral cell that somehow underwent conversion from a normal to a cancerous state. These insights, as straight for ward as they seem, were surprisingly difficult to reach. How could biologists describe the cell pedigree of a mass of cells that eventually is recognized as a tumor?
One approach to identifying the origin of cancer cells came from attempts to transplant tissues from one person to another. Such transplants work well between identical twins, but less well as the people involved are more distantly related. The barrier to successful transplantation exists because the recipient's immune system can distinguish between cells that have always lived inside the self and cells of foreign origin. One practical application of this discovery is that tissues can be classified as matching or nonmatching before a doctor attempts to graft a tissue or organ into another person's body. Such tissue-typing tests, when done on cancer cells, reveal that the tumor cells of a particular cancer patient are always of the same transplantation type as the cells of normal tissues located elsewhere in the person's body. Tumors, therefore, arise from one's own tissues, not from cells introduced into the body by infection from another person.
How do we know that tumors are monoclonal? Two distinct scenarios might explain how cancers develop within normal tissues. In the first, many individual cells become cancerous, and the resulting tumor represents the descendants of these original cells. In this case, the tumor is polyclonal in nature (Figure 4). In the second scenario, only one cell experiences the original transformation from a normal cell to a cancerous cell, and all of the cells in the tumor are descendants of that cell.

Figure 4
Two schemes by which tumors can develop. Most—if not all—human cancer appears to be monoclonal.
Direct evidence supporting the monoclonal origin of virtually all malignant tumors has been difficult to acquire because most tumor cells lack obvious distinguishing marks that scientists can use to demonstrate their clonal relationship. There is, however, one cellular marker that scientists can use as an indication of such relationships: the inactivated X chromosome that occurs in almost all of the body cells of a human female. X-chromosome inactivation occurs randomly in all cells during female embryonic development. Because the inactivation is random, the female is like a mosaic in terms of the X chromosome, with different copies of the X turned on or off in different cells of the body. Once inactivation occurs in a cell, all of the future generations of cells coming from that cell have the same chromosome inactivated in them as well (either the maternal or the paternal X). The observation that all the cells within a given tumor invariably have the same X chromosome inactivated suggests that all cells in the tumor must have descended from a single ancestral cell.
Cancer, then, is a disease in which a single normal body cell undergoes a genetic transformation into a cancer cell. This cell and its descendants, proliferating across many years, produce the population of cells that we recognize as a tumor, and tumors produce the symptoms that an individual experiences as cancer.
Even this picture, although accurate in its essence, did not represent a complete description of the events involved in tumor formation. Additional research revealed that as a tumor develops, the cells of which it is composed become different from one another as they acquire new traits and form distinct subpopulations of cells within the tumor. As shown in Figure 5, these changes allow the cells that experience them to compete with increasing success against cells that lack the full set of changes. The development of cancer, then, occurs as a result of a series of clonal expansions from a single ancestral cell.

Figure 5
A series of changes leads to tumor formation. Tumor formation occurs as a result of successive clonal expansions. This figure illustrates only three such changes; the development of many cancers likely involves more than three.
A second critical understanding that emerged from studying the biology of cancer cells is that these cells show a wide range of important differences from normal cells. For example, cancer cells are genetically unstable and prone to rearrangements, duplications, and deletions of their chromosomes that cause their progeny to display unusual traits. Thus, although a tumor as a whole is monoclonal in origin, it may contain a large number of cells with diverse characteristics.
Cancerous cells also look and act differently from normal cells. In most normal cells, the nucleus is only about one-fifth the size of the cell; in cancerous cells, the nucleus may occupy most of the cell's volume. Tumor cells also often lack the differentiated traits of the normal cell from which they arose. Whereas normal secretory cells pro duce and release mucus, cancers derived from these cells may have lost this characteristic. Likewise, epithelial cells usually contain large amounts of keratin, but the cells that make up skin cancer may no longer accumulate this protein in their cytoplasms.
The key difference between normal and cancerous cells, however, is that cancer cells have lost the restraints on growth that characterize normal cells. Significantly, a large number of cells in a tumor are engaged in mitosis, whereas mitosis is a relatively rare event in most normal tissues. Cancer cells also demonstrate a variety of unusual characteristics when grown in culture; two such examples are a lack of contact inhibition and a reduced dependence on the presence of growth factors in the environment. In contrast to normal cells, cancer cells do not cooperate with other cells in their environment. They often proliferate indefinitely in tissue culture. The ability to divide for an apparently unlimited number of generations is another important characteristic of the cancerous state, allowing a tumor composed of such cells to grow without the constraints that normally limit cell growth.
A unified view
By the mid-1970s, scientists had started to develop the basis of our modern molecular understanding of cancer. In particular, the relationship Ames and others had established between mutagenicity and carcinogenicity pro vided substantial support for the idea that chemical carcinogens act directly through their ability to damage cellular genes. This idea led to a straightforward model for the initiation of cancer: Carcinogens induce mutations in critical genes, and these mutations direct the cell in which they occur, as well as all of its progeny cells, to grow abnormally. The result of this abnormal growth appears years later as a tumor. The model could even explain the observation that cancer sometimes appears to run in families: If cancer is caused by mutations in critical genes, then people who inherit such mutations would be more susceptible to cancer's development than people who do not.
As exciting as it was to see a unified view of cancer begin to emerge from the earlier confusion, cancer researchers knew their work was not finished. The primary flaw in their emerging explanation was that the nature of these cancer-causing mutations was unknown. Indeed, their very existence had yet to be proven. Evidence from work with cancer-causing viruses suggested that only a small number of genes were involved, and evidence from cell biology pointed to genes that normally control cell division. But now scientists asked new questions: Exactly which genes are involved? What are their specific roles in the cell? and How do their functions change as a result of mutation?
It would take another 20 years and a revolution in the techniques of biological research to answer these questions. However, today our picture of the causes and development of cancer is so detailed that scientists find themselves in the extraordinary position of not only knowing many of the genes involved but also being able to target prevention, detection, and treatment efforts directly at these genes.
Cancer as a Multistep Process
A central feature of today's molecular view of cancer is that cancer does not develop all at once, but across time, as a long and complex succession of genetic changes. Each change enables precancerous cells to acquire some of the traits that together create the malignant growth of cancer cells.
Two categories of genes play major roles in triggering cancer. In their normal forms, these genes control the cell cycle, the sequence of events by which cells enlarge and divide. One category of genes, called proto-oncogenes, encourages cell division. The other category, called tumor suppressor genes, inhibits it. Together, proto-oncogenes and tumor suppressor genes coordinate the regulated growth that normally ensures that each tissue and organ in the body maintains a size and structure that meets the body's needs.
What happens when proto-oncogenes or tumor suppressor genes are mutated? Mutated proto oncogenes become oncogenes, genes that stimulate excessive division. And mutations in tumor suppressor genes inactivate these genes, eliminating the critical inhibition of cell division that normally prevents excessive growth. Collectively, mutations in these two categories of genes account for much of the uncontrolled cell division that occurs in human cancers (Figure 6).
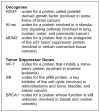
Figure 6
Some Genes Involved in Human Cancer
The role of oncogenes
How do proto-oncogenes, or more accurately, the oncogenes they become after mutation, contribute to the development of cancer? Most proto-oncogenes code for proteins that are involved in molecular pathways that receive and process growth-stimulating signals from other cells in a tissue. Typically, such signaling begins with the production of a growth factor, a protein that stimulates division. These growth factors move through the spaces between cells and attach to specific receptor proteins located on the surfaces of neighboring cells. When a growth-stimulating factor binds to such a receptor, the receptor conveys a stimulatory signal to proteins in the cytoplasm. These proteins emit stimulatory signals to other proteins in the cell until the division-promoting message reaches the cell's nucleus and activates a set of genes that help move the cell through its growth cycle.
Oncogenes, the mutated forms of these proto oncogenes, cause the proteins involved in these growth-promoting pathways to be overactive. Thus, the cell proliferates much faster than it would if the mutation had not occurred. Some oncogenes cause cells to overproduce growth factors. These factors stimulate the growth of neighboring cells, but they also may drive excessive division of the cells that just produced them. Other oncogenes produce aberrant receptor proteins that release stimulatory signals into the cytoplasm even when no growth factors are present in the environment. Still other oncogenes disrupt parts of the signal cascade that occurs in a cell's cytoplasm such that the cell's nucleus receives stimulatory messages continuously, even when growth factor receptors are not prompting them.
The role of tumor suppressor genes
To become cancerous, cells also must break free from the inhibitory messages that normally counterbalance these growth-stimulating pathways. In normal cells, inhibitory messages flow to a cell's nucleus much like stimulatory messages do. But when this flow is interrupted, the cell can ignore the normally powerful inhibitory messages at its surface.
Scientists are still trying to identify the normal functions of many known tumor suppressor genes. Some of these genes apparently code for proteins that operate as parts of specific inhibitory pathways. When a mutation causes such proteins to be inactivate or absent, these inhibitory pathways no longer function normally. Other tumor suppressor genes appear to block the flow of signals through growth-stimulating pathways; when these genes no longer function properly, such growth-promoting pathways may operate without normal restraint. Mutations in all tumor suppressor genes, however, apparently inactivate critical tumor suppressor proteins, depriving cells of this restraint on cell division.
The body's back-up systems
In addition to the controls on proliferation afforded by the coordinated action of proto-oncogenes and tumor suppressor genes, cells also have at least three other systems that can help them avoid runaway cell division. The first of these systems is the DNA repair system. This system operates in virtually every cell in the body, detecting and correcting errors in DNA. Across a lifetime, a person's genes are under constant attack, both by carcinogens imported from the environment and by chemicals produced in the cell itself. Errors also occur during DNA replication. In most cases, such errors are rapidly corrected by the cell's DNA repair system. Should the system fail, however, the error (now a mutation) becomes a permanent feature in that cell and in all of its descendants.
The system's normally high efficiency is one reason why many years typically must pass before all the mutations required for cancer to develop occur together in one cell. Mutations in DNA repair genes themselves, however, can undermine this repair system in a particularly devastating way: They damage a cell's ability to repair errors in its DNA. As a result, mutations appear in the cell (including mutations in genes that control cell growth) much more frequently than normal.
A second cellular back-up system prompts a cell to commit suicide (undergo apoptosis) if some essential component is damaged or its control system is deregulated. This observation suggests that tumors arise from cells that have managed to evade such death. One way of avoiding apoptosis involves the p53 protein. In its normal form, this protein not only halts cell division, but induces apoptosis in abnormal cells. The product of a tumor suppressor gene, p53 is inactivated in many types of cancers.
This ability to avoid apoptosis endangers cancer patients in two ways. First, it contributes to the growth of tumors. Second, it makes cancer cells resistant to treatment. Scientists used to think that radiation and chemotherapeutic drugs killed cancer cells directly by harming their DNA. It seems clear now that such therapy only slightly damages the DNA in cells; the damaged cells, in response, actively kill themselves. This discovery suggests that cancer cells able to evade apoptosis will be less responsive to treatment than other cells.
A third back-up system limits the number of times a cell can divide, and so assures that cells cannot reproduce endlessly. This system is governed by a counting mechanism that involves the DNA segments at the ends of chromosomes. Called telomeres, these segments shorten each time a chromo some replicates. Once the telomeres are shorter than some threshold length, they trigger an internal signal that causes the cell to stop dividing. If the cells continue dividing, further shortening of the telomeres eventually causes the chromosomes to break apart or fuse with one another, a genetic crisis that is inevitably fatal to the cell.
Early observations of cancer cells grown in culture revealed that, unlike normal cells, cancer cells can proliferate indefinitely. Scientists have recently discovered the molecular basis for this characteristic—an enzyme called telomerase, that systematically replaces telomeric segments that are trimmed away during each round of cell division. Telomerase is virtually absent from most mature cells, but is present in most cancer cells, where its action enables the cells to proliferate endlessly.
The multistep development of cancer
Cancer, then, does not develop all at once as a massive shift in cellular functions that results from a mutation in one or two wayward genes. Instead, it develops step-by-step, across time, as an accumulation of many molecular changes, each contributing some of the characteristics that eventually pro duce the malignant state. The number of cell divisions that occur during this process can be astronomically large—human tumors often become apparent only after they have grown to a size of 10 billion to 100 billion cells. As you might expect, the time frame involved also is very long— it normally takes decades to accumulate enough mutations to reach a malignant state.
Understanding cancer as a multistep process that occurs across long periods of time explains a number of long-standing observations. A key observation is the increase in incidence with age. Cancer is, for the most part, a disease of people who have lived long enough to have experienced a complex and extended succession of events. Because each change is a rare accident requiring years to occur, the whole process takes a very long time, and most of us die from other causes before it is complete.
Understanding cancer in this way also explains the increase in cancer incidence in people who experience unusual exposure to carcinogens, as well as the increased cancer risk of people who inherit predisposing mutations. Exposure to carcinogens increases the likelihood that certain harmful changes will occur, greatly increasing the probability of developing cancer during a normal life span. Similarly, inheriting a cancer -susceptibility mutation means that instead of that mutation being a rare event, it already has occurred, and not just in one or two cells, but in all the body's cells. In other words, the process of tumor formation has leapfrogged over one of its early steps. Now the accumulation of changes required to reach the malignant state, which usually requires several decades to occur, may take place in one or two.
Finally, understanding the development of cancer as a multistep process also explains the lag time that often separates exposure to a cancer-causing agent and the development of cancer. This explains, for example, the observation that severe sunburns in children can lead to the development of skin cancer decades later. It also explains the 20-to 25-year lag between the onset of widespread cigarette smoking among women after World War II and the massive increase in lung cancer that occurred among women in the 1970s.
The Human Face of Cancer
For most Americans, the real issues associated with cancer are personal. More than 8 million Americans alive today have a history of cancer (National Cancer Institute, 1998; Rennie, 1996). In fact, cancer is the second leading cause of death in the United States, exceeded only by heart disease.
Who are these people who develop cancer and what are their chances for surviving it? Scientists measure the impact of cancer in a population by looking at a combination of three elements: (1) the number of new cases per year per 100,000 persons (incidence rate), (2) the number of deaths per 100,000 persons per year (mortality rate), and (3) the proportion of patients alive at some point after their diagnosis of cancer (survival rate). Data on incidence, mortality, and survival are collected from a variety of sources. For example, in the United States there are many statewide cancer registries and some regional registries based on groups of counties, many of which surround large metropolitan areas. Some of these population-based registries keep track of cancer incidence in their geographic areas only; others also collect follow-up information to calculate survival rates.
In 1973, the National Cancer Institute began the Surveillance, Epidemiology, and End Results (SEER) Program to estimate cancer incidence and patient survival in the United States. SEER collects cancer incidence data in 11 geographic areas and two supplemental registries, for a combined population of approximately 14 percent of the entire U.S. population. Data from SEER are used to track cancer incidence in the United States by primary cancer site, race, sex, age, and year of diagnosis. For example, Figure 7 shows SEER data for the age-adjusted cancer incidence rates for the 10 most common sites for Caucasian and African-American males and females for the period 1987–1991.
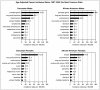
Figure 7
Age-Adjusted Cancer Incidence Rates, 1987–1991
Cancer among children is relatively rare. SEER data from 1991 showed an incidence of only 14.1 cases per 100,000 children under age 15. Nevertheless, after accidents, cancer is the second leading cause of childhood death in the United States. Leukemias (4.3 per 100,000) and cancer of the brain and other nervous system organs (3.4 per 100,000) account for more than one-half of the cancers among children.
Everyone is at some risk of developing cancer. Cancer researchers use the term lifetime risk to indicate the probability that a person will develop cancer over the course of a lifetime. In the United States, men have a 1 in 2 lifetime risk of developing cancer, and women have a 1 in 3 risk.
For a specific individual, however, the risk of developing a particular type of cancer may be quite different from his or her lifetime risk of developing any type of cancer. Relative risk compares the risk of developing cancer between persons with a certain exposure or characteristic and persons who do not have this exposure or characteristic. For example, a person who smokes has a 10- to 20-fold higher relative risk of developing lung cancer compared with a person who does not smoke. This means that a smoker is 10- to 20-times more likely to develop lung cancer than a nonsmoker.
Scientists rely heavily on epidemiology to help them identify factors associated with the development of cancer. Epidemiologists look for factors that are common to cancer victims' histories and lives and evaluate these factors in the light of current understandings of the disease. With enough study, researchers may assemble evidence that a particular factor "causes" cancer, that is, that exposure to it increases significantly the probability of the disease developing. Although this information cannot be used to predict what will happen to any one individual exposed to this risk factor, it can help people make choices that reduce their exposure to known carcinogens (cancer-causing agents) and increase the probability that if cancer develops, it will be detected early (for example, by getting regular check-ups and participating in cancer screening programs).
As noted above, hereditary factors also can contribute to the development of cancer. Some people are born with mutations that directly promote the unrestrained growth of certain cells or the occurrence of more mutations. These mutations, such as the mutation identified in the 1980s that causes retinoblastoma, confer a high relative cancer risk. Such mutations are rare in the population, however, accounting for the development of fewer than 5 percent of the cases of fatal cancer.
Hereditary factors also contribute to the development of cancer by dictating a person's general physiological traits. For example, a person with fair skin is more susceptible to the development of skin cancer than a person with a darker complexion. Likewise, a person whose body metabolizes and eliminates a particular carcinogen relatively inefficiently is more likely to develop types of cancer associated with that carcinogen than a person who has more efficient forms of the genes involved in that particular metabolic process. These inherited characteristics do not directly promote the development of cancer; each person, susceptible or not, still must be exposed to the related environ mental carcinogen for cancer to develop. Nevertheless, genes probably do contribute in some way to the vast majority of cancers.
One question often asked about cancer is "How many cases of cancer would be expected to occur naturally in a population of individuals who somehow had managed to avoid all environmental carcinogens and also had no mutations that predisposed them to developing cancer?" Comparing populations around the world with very different cancer patterns has led epidemiologists to suggest that perhaps only about 25 percent of all cancers are "hard core"—that is, would develop anyway, even in a world free of external influences. These cancers would occur simply because of the production of carcinogens within the body and because of the random occurrence of unrepaired genetic mistakes.
Although cancer continues to be a significant health issue in the United States, a recent report from the American Cancer Society (ACS), National Cancer Institute (NCI), and Centers for Disease Control and Prevention (CDC) indicates that health officials are making progress in controlling the disease. In a news bulletin released on 12 March 1998, the ACS, NCI, and CDC announced the first sustained decline in the cancer death rate, a turning point from the steady increase observed throughout much of the century. The report showed that after increasing 1.2 percent per year from 1973 to 1990, the incidence for all cancers combined declined an average of 0.7 percent per year from 1990 to 1995. The overall cancer death rate also declined by about 0.5 percent per year across this period.
The overall survival rate for all cancer sites combined also continues to increase steadily, from 49.3 percent in 1974–1976 to 53.9 percent in 1983–1990 (Figure 8). In some cases—for example, among children age 15 and younger—survival rates have increased dramatically.
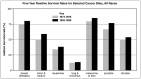
Figure 8
Five-Year Relative Survival Rates for Selected Cancer Sites, All Races
New Hope for Treating Cancer
What explanation can we offer for the steady increase in survival rates among cancer patients? One answer likely is the improvements scientists have made in cancer detection. These improvements include a variety of new imaging techniques as well as blood and other tests that can help physicians detect and diagnose cancer early. Although many Americans regularly watch for the early symptoms of cancer, by the time symptoms occur many tumors already have grown quite large and may have metastasized. Likewise, many cancers have no symptoms. Clearly, great effort is needed to educate Americans that cancer screening (checking for cancer in people with no symptoms) is key to early detection.
Another explanation for increased survival is improved treatment. Today, the traditional workhorses of cancer treatment—surgery, radiation, and chemotherapy—are being used in ways that are increasingly specific to the type of cancer involved. In fact, many cases of cancer now are being fully cured.
But is this the best we can do? What will the future bring? Hellman and Vokes, in their 1996 article in Scientific American, note that war often serves as a metaphor for cancer research. In 1971, two days before Christmas, President Richard M. Nixon signed the National Cancer Act, committing the United States to a "war" on cancer. Although the analogy is not perfect, Hellman and Vokes suggest that it can help us understand our current position with respect to cancer prevention, detection, and treatment. Looking at the "map" of cancer research after almost 30 years of "war," we can see that we have made some modest advances. But these successes do not reveal the tremendous developments that lie ahead of us by virtue of the new, strategic position we have achieved. In fact, most scientists expect that our newly gained understanding of the molecular basis of cancer will eventually give rise to a whole generation of exciting new techniques, not only for detecting and treating cancer but also for preventing it.
A key area of interest lies in learning how to exploit the molecular abnormalities of cancer cells to bring about their destruction. For example, understanding the role of oncogenes in the development of cancer suggests new targets for anticancer therapies. Some drug companies are working on drugs designed to shut down abnormal receptor proteins. Other potential targets are the aberrant proteins within the cytoplasm that transmit stimulatory signals even without being stimulated by surface receptors.
As in the case of oncogenes, a better understanding of the role of tumor suppressor genes in preventing runaway cell division may help scientists develop new therapies directed at these genes. For example, various studies have shown that introducing a normal tumor suppressor gene into a cell can help restore the cell to normalcy. Similarly, a therapy capable of restoring a cell's capacity for apoptosis would improve significantly the effectiveness of current cancer treatments. Even telomerase represents an important potential target for scientists looking for new and more powerful treatments for cancer. If telomerase could be blocked in cancer cells, their telomeres would continue to shorten with each division until their own proliferation pushed them into a genetic crisis and death.
One bold new research initiative that offers significant promise is the Cancer Genome Anatomy Project (CGAP). The project's goal is to identify all the genes responsible for the establishment and growth of human cancer. The work is based on a simple concept: Although almost every cell in the body contains the full set of human genes, only about one-tenth of them are expressed in any particular type of cell. Thus, different types of cells— for example, muscle cells and skin cells—can be distinguished by their patterns of gene expression.
Establishing for a particular cell the repertoire of genes expressed, together with the amount of nor mal or altered gene product produced by each expressed gene, yields a powerful "fingerprint" or "signature" for that cell type. Not unexpectedly, during the transformation of a normal cell to a cancer cell, this signature changes. Some changes are quantitative. That is, gene A may be expressed in both cells, but at greatly different levels, or it may be expressed in one cell but not the other. Other changes are qualitative: Gene B may be expressed at the same level in both cells, but pro duce an altered product in the cancerous cell.
Scientists expect that being able to "read" these signatures—in other words, being able to compare the signatures of cells in their normal and cancerous states—will change cancer detection, diagnosis, and treatment in many exciting ways. Specifically, studying the exact sequence of molecular changes a cell undergoes during its transformation to a cancerous state will help scientists identify new molecular-level targets for prevention, detection, and treatment. One observation scientists have recently made is that cells surrounding an incipient tumor also may undergo changes that indicate that cancer is present. For example, early tobacco-induced molecular changes in the mouth may predict the risk of developing lung cancer, and cancers of the urinary tract may be signaled by molecularly-altered cells that are shed in the urine. Reading the signatures of these easily accessed cells may enable scientists to develop simple, non-invasive tests that will allow early detection of cancerous or precancerous cells hidden deep within the body.
Reading such signatures will also enhance the specificity of cancer diagnosis by allowing scientists to differentiate among tumors at the molecular level. By assessing the meaning of individual changes in a cell's signature, scientists will be able to determine which cancers are most likely to progress and which are not—a dilemma that confronts doctors in the treatment of prostate cancer—thereby allowing patients to avoid the harmful consequences of unnecessary treatment.
Finally, molecular fingerprinting will allow researchers to develop new treatments specifically targeted at cellular subtypes of different cancers. Often, patients suffering from tumors that by traditional criteria are indistinguishable, nevertheless experience quite different outcomes despite having received the same treatment. Research indicates that these different outcomes sometimes are related to the presence or absence of particular gene products. In the future, such molecular characteristics likely will be used to identify patients who would benefit from one type of treatment as compared with another.
The ultimate goal of such work, of course, is to push back the detection and diagnosis of cancer to its earliest stages of development. For the first time in the history of humankind, scientists can now envision the day when medical intervention for cancer will become focused at identifying incipient disease and preventing its progression to overt disease, rather than treating the cancer after it is well established.
Cancer and Society
But what does this mean for society? The financial costs of cancer loom large, not only for the individual but also for the community. The NCI estimates overall annual costs for cancer at about $107 billion. This cost includes $37 billion for direct medical costs, $11 billion for morbidity costs (cost of lost productivity), and $59 billion for mortality costs. Interestingly, treatment for breast, lung, and prostate cancers account for more than one-half of the direct medical costs.
Although early detection and successful treatment can reduce cancer deaths, the most desirable way to reduce them is prevention. In fact, scientists estimate that as many as one-half of the deaths from cancer in the United States and Europe, two areas with closely tracked cancer rates, could theoretically be prevented.
Nevertheless, the widespread persistence of unhealthful habits suggests that many Americans remain unconvinced about the power of prevention as a defense against cancer. Part of the reason may be that the only data we have about factors related to cancer are drawn from whole populations. These data cannot tell us who will develop cancer. Nor can they tell us whether healthful choices prevented its appearance in a particular individual.
Unhealthful habits also may persist because of the long time that elapses between the exposures that trigger the development of cancer and its actual appearance as disease. Conversely, there is a time lag between the institution of a beneficial personal habit (such as quitting smoking) or public policy (such as banning use of a known carcinogen) and its positive impact on personal and public health.
In their article "Strategies for Minimizing Cancer Risk," Willett, Colditz, and Mueller propose four levels on which to focus cancer prevention efforts. The first level is that of the individual. These authors argue that because most of the actions that can prevent cancer must be taken by individuals, dissemination of accurate information directly to the American public, together with peer support for behavioral changes, are critical.
A second level is health care providers, who are in a position to provide both counseling and screening to individuals under their care. Here, dissemination of accurate and timely information also is key.
A third level of prevention is the national level, where government agencies can impose regulations that help minimize the public's exposure to known carcinogens and implement policies that improve public health. Examples include regulating industries to cease using potent carcinogens and providing community facilities for safe physical activity.
Finally, a fourth level of prevention is at the international level, where the actions of developed countries can affect the incidence of cancer worldwide. Unfortunate examples of this include promoting the exportation of tobacco products and moving hazardous manufacturing processes to unregulated developing countries.
How do we think about devising and implementing measures to improve personal and public health in a pluralist society? One way to address this question is by attending to the ethical and public policy issues raised by our understanding and treatment of cancer.

Figure 9
A history of severe sunburns is strongly linked to the development of skin cancer later in life.
Ethics is the study of good and bad, right and wrong. It has to do with the actions and character of individuals, families, communities, institutions, and societies. During the last 2,500 years, Western philosophy has developed a variety of powerful methods and a reliable set of concepts and technical terms for studying and talking about the ethical life. Generally speaking, we apply the terms "right" and "good" to actions and qualities that foster the interests of individuals, families, communities, institutions, and society. Here, an "interest" refers to a participant's share in a situation. The terms "wrong" or "bad" apply to actions and qualities that impair interests. Often there are competing, well-reasoned answers to questions about what is right and wrong and good and bad about an individual's or group's conduct or actions.
Ethical considerations are complex, multifaceted, and raise many questions. In the United States, for example, we value protecting individuals from preventable harms. We support restrictions on who can purchase cigarettes and where smoking can occur. We inform pregnant women of the risks of drinking and smoking. However, we also value individual freedom and autonomy. We do not ban cigarettes outright; instead, we allow individuals over 18 years of age to take personal risks and be exposed to the related consequences. We permit pregnant women to buy and use liquor and cigarettes.
The inevitability of ethical tradeoffs is not simply a mark of the discussions in the United States. When considering differing health policy issues between and among countries, one cannot avoid encountering a pluralism of ethical considerations. Developing countries, whose health standards often differ from those in the United States, provide different cultural approaches to cancer and different standards for marketing and using tobacco and other known carcinogens. These different approaches raise a variety of ethical questions. For example, is there any legal and ethical way for people in the United States to prevent the widespread use of tobacco in other countries, a practice that contributes to the rise of lung cancer worldwide? Is there any legal and ethical way to govern other choices of individuals (for example, poor diet and lack of exercise) that contribute to cancer?
Typically, answers to such questions all involve an appeal to values. A value is something that has significance or worth in a given situation. One of the exciting events to witness in any discussion in ethics in a pluralist society is the varying ways in which the individuals involved assign value to things, persons, and states of affairs. Examples of values that students may appeal to in discussions of ethical issues include autonomy, freedom, privacy, sanctity of life, protecting another from harm, promoting another's good, justice, fairness, relationships, scientific knowledge, and technological progress.
Acknowledging the complex, multifaceted nature of ethical discussions is not to suggest that "anything goes." Experts generally agree on the following features of ethics. First, ethics is a process of rational inquiry. It involves posing clearly formulated questions and seeking well-reasoned answers to those questions. Well-reasoned answers to ethical questions constitute arguments. Ethical analysis and argument, then, result from successful ethical inquiry.
Second, ethics requires a solid foundation of information and rigorous interpretation of that information. For example, one must have a solid understanding of cancer to discuss the ethics of requiring protective covering to be worn to prevent skin cancer. Ethics is not strictly a theoretical discipline but is concerned in vital ways with practical matters.
Third, because tradeoffs among interests are complex, constantly changing, and sometimes uncertain, there are often competing, well-reasoned answers to questions about what is right and wrong and good and bad. This is especially true in a pluralist society.
Public policy is a set of guidelines or rules that results from the actions or lack of actions of government entities. Government entities act by making laws. In the United States, laws can be made by each of the three branches of government: by legislatures (statutory law), by courts (case law), and by regulatory agencies (regulatory law). Regulatory laws are written by the executive branch of the government, under authorization by the legislative branch. All three types of law are pertinent to how we respond to cancer. When laws exist to regulate behavior, public policy is called de jure public policy.
Whether one makes public policy involves at least the following five considerations:
- -the costs of implementing particular policies (including financial, social, and personal costs),
- the urgency of implementing a new policy,
- how effective a particular policy is likely to be,
- -whether appropriate means exist to implement the policy, and
- social, cultural, and political factors.
For example, many argue that there is overwhelming evidence to support increased public policy restrictions on access to and use of cigarettes. Cigarette smoking is said to be linked to 85–90 percent of lung cancer cases. In 1998, 171,500 new cases of lung cancer were predicted. Of these, 160,100 were expected to end in death. Public policy prohibitions on cigarette use and access may be seen to satisfy four of the five criteria: (1) the cost of the policy would be minimal because cigarette access and use restrictions are in place, (2) the urgency of the situation is serious given the large number of deaths, (3) prohibiting purchase by minors and raising the prices (through taxation) are seen as effective, and (4) means are already in place for additional restrictions. The challenge in this era of high economic interest in cigarette production is the social, cultural, and political considerations (5).

Figure 10
Where do we spend our money? A consequence of allowing unhealthful habits, such as smoking, is that public funds may be spent on cancer treatments instead of on other societal benefits, such as improved school facilities.
It is important to recognize that sometimes the best public policy is not to enact a law in response to a controversy, but rather to allow individuals, families, communities, and societies to act in the manner they choose. Clearly, de jure public policy can only go so far in regulating people's behaviors. De jure public policy in the United States offers no match for the addictive power of nicotine and the marketing clout of the tobacco industry. In addition, any decline in cigarette use brought about by de jure public policy in the United States has been more than offset in recent years by a rapid increase of cigarette consumption elsewhere in the world.
When no laws exist to regulate behavior, public policy is called de facto (actual) public policy. With regard to lung cancer prevention programs, many think that other approaches are needed: improved general education and cultivation of an antismoking ethos. In any discussion of society's response to a social problem, it is important to think about other ways to address the problem.
Knowledge, choice, behavior, and human welfare
We can conclude that science plays an important role in assisting individuals to make choices about enhancing personal and public welfare. Science provides evidence that can be used to support ways of understanding and treating human disease, ill ness, deformity, and dysfunction. But the relationships between scientific information and human choices, and between choices and behaviors, are not linear. Human choice allows individuals to choose against sound knowledge, and choice does not necessarily lead to particular actions.
Nevertheless, it is increasingly difficult for most of us to deny the claims of science. We are continually presented with great amounts of relevant scientific and medical knowledge that is publicly accessible. We are fortunate to have available a large amount of convincing data about the development, nature, and treatment of particular cancers. As a consequence, we might be encouraged to think about the relationships among knowledge, choice, behavior, and human welfare in the following ways:
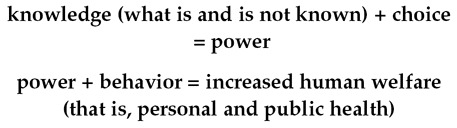
One of the goals of this module is to encourage students to think in terms of these relationships, now and as they grow older.
References
- Baron-Faust R. Breast cancer: What every woman should know. New York: Hearst Books; 1995.
- Biological Sciences Curriculum Study. Teaching tools. Dubuque, IA: Kendall/Hunt Publishing Company; 1999.
- Bonwell CC, Eison JA. Active learning: Creating excitement in the classroom. Washington, DC: The George Washington University: School of Education and Human Development; 1991. (ASHE-ERIC Higher Education Report No. 1)
- Brody CM. Collaborative or cooperative learning? Complementary practices for instructional reform. The Journal of Staff, Program, & Organizational Development. 1995;12(3):134–143.
- Knapp MS, Shields PM, Turnbull BJ. Academic challenge in high-poverty classrooms. Phi Delta Kappan. 1995;76(10):770–776.
- McKinnell RG, Parchment RE, Perantoni AO, Pierce GB. The biological basis of cancer. New York: Cambridge University Press; 1998.
- Murphy GP, Morris LB, Lange D. Informed decisions: The complete book of cancer diagnosis, treatment, and recovery. Viking Penguin: The American Cancer Society; 1997.
- National Cancer Institute. New report on declining cancer incidence and death rates: Report shows progress in controlling cancer. March 12, 1998. [Online press release]. Available http://nci
.nih.gov/massmedia /pressreleases/deathrate.html. - National Institutes of Health. Congressional justification. Bethesda, MD: Author; 1996.
- National Institutes of Health (NIH). Jun, 1999. [Online]. Available http://www
.nih.gov. - National Research Council. National science education standards. Washington, DC: National Academy Press; 1996.
- Moore JA. Science as a way of knowing: The foundations of modern biology. Cambridge, MA: Harvard University Press; 1993.
- Patterson JT. The dread disease: Cancer and modern American culture. Cambridge, MA: Harvard University Press; 1987.
- Perkins D. Smart schools: Better thinking and learning for every child. New York: The Free Press; 1992.
- Project Kaleidoscope. What works: Building natural science communities. Vol. 1. Washington, DC: Stamats Communications, Inc; 1991.
- Rennie J, Rusting, R Making headway against cancer. Scientific American. 1996 September;275(3):56. [PubMed: 8701294]
- Rennie J. Editorial. Scientific American. 1996 September;275(3):6.
- Roblyer MD, Edwards J, Havriluk MA. Integrating educational technology into teaching. Upper Saddle River, NJ: Prentice-Hall, Inc; 1997.
- Saunders WL. The constructivist perspective: Implications and teaching strategies for science. School Science and Mathematics. 1992;92(3):136–141.
- Sizer TR. New York: Houghton Mifflin Co; Horace's school: Redesigning the American high school. 1992
- Trichopoulos D, Li FP, Hunter DJ. What causes cancer? Scientific American. 1996 September;275(3):80. [PubMed: 8701297]
- Varmus H, Weinberg RA. Genes and the biology of cancer. New York: Scientific American Library; 1993.
- Vogelstein B, Kinzler KW. The genetic basis of cancer. New York: McGraw Hill; 1998.
- Weinberg RA. Racing to the beginning of the road: The search for the origin of cancer. New York: Harmony Books; 1996.
- Weinberg RA. How cancer arises. Scientific American. 1996 September;275(3):62. [PubMed: 8701295]
- Willett WC, Colditz GA, Mueller NE. Strategies for minimizing cancer risk. Scientific American. 1996 September;275(3):88. [PubMed: 8701299]
Glossary
The following glossary was modified from the glossary on the National Cancer Institute's Web site, available from http://www.nci.nih.gov.
- acute lymphocytic leukemia
Type of blood cancer that originates in lymphatic cells of the bone marrow.
- acute myelogenous leukemia
Type of blood cancer that involves accumulation of myeloid cells in the bone marrow and bloodstream.
- adenocarcinoma
Cancer that begins in cells that line certain internal organs.
- adenoma
Noncancerous tumor.
- alpha-fetoprotein
Protein often found in abnormal amounts in the blood of patients with liver cancer.
- Ames test
Mutagenesis assay (a measure of mutagenic ability) that involves specially engineered strains of bacteria. Because of the relationship between mutagenicity and carcinogenicity, the test is used as a rapid and relatively inexpensive first screening of untested chemicals that are suspected to be carcinogens.
- anaplastic
Term used to describe cancer cells that divide rapidly and bear little or no resemblance to normal cells.
- angiogenesis
Blood vessel formation, which usually accompanies the growth of malignant tissue.
- angiosarcoma
Type of cancer that begins in the lining of blood vessels.
- apoptosis
Normal cellular process involving a genetically programmed series of events leading to the death of a cell.
- asymptomatic
Presenting no signs or symptoms of disease.
- ataxia telangiectasia
Hereditary disorder characterized by problems with muscle coordination, immunodeficiency, inadequate DNA repair, and an increased risk of developing cancer.
- atypical hyperplasia
Benign (noncancerous) condition in which tissue has certain abnormal features.
- basal cell
Small, round cell found in the lower part, or base, of the epidermis, the outer layer of the skin.
- basal cell carcinoma
Type of skin cancer that arises from the basal cells.
- benign
Not cancerous; does not invade nearby tissue or spread to other parts of the body.
- benign tumor
A noncancerous growth that does not spread to other parts of the body.
- biological therapy
Use of the body's immune system, either directly or indirectly, to fight cancer or to lessen side effects that may be caused by some cancer treatments. Also known as immuno-therapy, biotherapy, or biological response modifier therapy.
- biopsy
Removal of a sample of tissue, which is then examined under a microscope to check for cancer cells.
- bone marrow
Soft, spongy tissue in the center of large bones that produces white blood cells, red blood cells, and platelets.
- bone marrow aspiration
Removal of a small sample of bone marrow (usually from the hip) through a needle for examination under a microscope to see whether cancer cells are present.
- bone marrow biopsy
Removal of a sample of tissue from the bone marrow with a large needle. The cells are checked to see whether they are cancerous. If cancerous plasma cells are found, the pathologist estimates how much of the bone marrow is affected. Bone marrow biopsy is usually done at the same time as bone marrow aspiration.
- bone marrow transplantation
Procedure in which doctors replace marrow destroyed by treatment with high doses of anticancer drugs or radiation. The replacement marrow may be taken from the patient before treatment or may be donated by another person.
- bone scan
Technique to create images of bones on a computer screen or on film. A small amount of radioactive material is injected and travels through the bloodstream. It collects in the bones, especially in abnormal areas of the bones, and is detected by a scanner.
- brachytherapy
Internal radiation therapy using an implant of radioactive material placed directly into or near the tumor.
- BRCA1
Gene located on chromosome 17 that normally helps restrain cell growth. Inheriting an altered version of BRCA1 predisposes an individual to breast, ovarian, or prostate cancer.
- BRCA2
Gene located on chromosome 13 that scientists believe may account for 30 to 40 percent of all inherited breast cancer.
- breast reconstruction
Surgery to rebuild a breast's shape after a mastectomy.
- Burkitt lymphoma
Type of non-Hodgkin lymphoma that most often occurs in young people between the ages of 12 and 30. The disease usually causes a rapidly growing tumor in the abdomen.
- cancer
Term for a group of more than 100 diseases in which abnormal cells divide without control. Cancer cells can invade nearby tissues and can spread through the bloodstream and lymphocytic system to other parts of the body.
- carcinogen
Any substance that is known to cause cancer.
- carcinogenesis
Process by which normal cells are transformed into cancer cells.
- carcinoma
Cancer that begins in the lining or covering of an organ.
- carcinoma in situ
Cancer that involves only the cells in which it began and has not spread to other tissues.
- CEA assay
Laboratory test to measure the level of carcinoembryonic antigen (CEA), a substance that is sometimes found in an increased amount in the blood of patients with certain cancers.
- cell cycle
Sequence of events by which cells enlarge and divide. Includes stages typically named G1, S, G2, and M.
- chemoprevention
Use of natural or laboratory-made substances to prevent cancer.
- chemotherapy
Treatment with anticancer drugs.
- chronic lymphocytic leukemia
Type of blood cancer that involves overproduction of mature lymphocytes.
- chronic myelogenous leukemia
Type of blood cancer that involves accumulation of granulocytes (a type of white blood cell) in the bone marrow and bloodstream.
- clinical trial
Research study that involves patients. Each study is designed to find better ways to prevent, detect, diagnose, or treat cancer and to answer scientific questions.
- colonoscopy
Procedure that uses a flexible fiber optic endoscope to examine the internal surface of the colon along its entire length.
- combination chemotherapy
Treatment in which two or more chemicals are used to obtain more effective results.
- computed tomography
X-ray procedure that uses a computer to produce a detailed picture of a cross section of the body; also called CAT or CT scan.
- contact inhibition
Inhibition of cell division in normal (noncancerous) cells when they contact a neighboring cell.
- CT (or CAT) scan
See computed tomography.
- cytotoxic
Poisonous to cells. In chemotherapy, used to describe an agent that is poisonous to cancer cells.
- diagnosis
Process of identifying a disease by the signs and symptoms.
- dysplasia
Abnormal cells that are not cancer.
- dysplastic nevi
Atypical moles; moles whose appearance is different from that of common moles. Dysplastic nevi are generally larger than ordinary moles and have irregular and indistinct borders. Their color often is not uniform and ranges from pink or even white to dark brown or black; they usually are flat, but parts may be raised above the skin surface.
- encapsulated
Confined to a specific area; an encapsulated tumor remains in a compact form.
- endometrial
Having to do with the mucous membrane that lines the cavity of the uterus.
- environmental tobacco smoke
Smoke that comes from the burning end of a cigarette and smoke that is exhaled by smokers. Also called ETS or secondhand smoke. Inhaling ETS is called involuntary or passive smoking.
- epidemiology
Study of the factors that affect the prevalence, distribution, and control of disease.
- epidermis
Upper or outer layer of the two main layers of cells that make up the skin.
- Epstein-Barr virus
Virus that has been associated with the development of infectious mononucleosis and also with Burkitt lymphoma.
- estrogen
Female hormone produced by the ovary. Responsible for secondary sex characteristics and cyclic changes in the lining of the uterus and vagina.
- etiology
Study of the causes of abnormal condition or disease.
- familial polyposis
Inherited condition in which several hundred polyps develop in the colon and rectum. These polyps have a high potential to become malignant.
- fecal occult blood test
Test to reveal blood hidden in the feces, which may be a sign of colon cancer.
- fiber
Parts of fruits and vegetables that cannot be digested. Also called bulk or roughage.
- fibroid
Benign uterine tumor made up of fibrous and muscular tissue.
- gene therapy
Treatment that alters genes (the basic units of heredity found in all cells in the body). In studies of gene therapy for cancer, researchers are trying to improve the body's natural ability to fight the disease or to make the tumor more sensitive to other kinds of therapy.
- genetic
Inherited; having to do with information that is passed from parents to children through DNA in the genes.
- grade
Describes how closely a cancer resembles normal tissue of its same type, along with the cancer's probable rate of growth.
- grading
System for classifying cancer cells in terms of how malignant or aggressive they appear microscopically. The grading of a tumor indicates how quickly cancer cells are likely to spread and plays a role in treatment decisions.
- herpes virus
Member of the herpes family of viruses. One type of herpes virus is sexually transmitted and causes sores on the genitals.
- hormonal therapy
Treatment of cancer by removing, blocking, or adding hormones.
- human papillomaviruses
Viruses that generally cause warts. Some papillomaviruses are sexually transmitted. Some of these sexually transmitted viruses cause wartlike growths on the genitals, and some are thought to cause abnormal changes in cells of the cervix.
- hyperplasia
Precancerous condition in which there is an increase in the number of normal cells lining an organ.
- imaging
Tests that produce pictures of areas inside the body.
- immunotherapy
Treatment that uses the body's natural defenses to fight cancer. Also called biotherapy or biological modifier response therapy.
- incidence
Number of new cases of a disease diagnosed each year.
- incidence rate
Number of new cases per year per 100,000 persons.
- initiation
Preneoplastic change in the genetic material of cells caused by a chemical carcinogen. Cancer develops when initiated cells are subsequently exposed to the same or another carcinogen.
- in situ cancer
Cancer that has remained within the tissue in which it originated.
- invasion
As related to cancer, the spread of cancer cells into healthy tissue adjacent to the tumor.
- invasive cancer
Cancer that has spread beyond the layer of tissue in which it developed.
- keratin
Insoluble protein that is the major constituent of the outer layer of the skin, nails, and hair.
- lesion
Area of abnormal tissue change.
- leukemia
Cancer of the blood cells.
- lifetime risk
Probability that a person, over the course of a lifetime, will develop cancer.
- Li-Fraumeni syndrome
Rare family predisposition to multiple cancers, caused by an alteration in the p53 tumor suppressor gene.
- lumen
An enclosed space bounded by an epithelial membrane; for example, the lumen of the gut.
- malignant
Cancerous; can invade nearby tissue and spread to other parts of the body.
- melanin
Skin pigment (substance that gives the skin its color). Dark-skinned people have more melanin than light-skinned people.
- melanocyte
Cell in the skin that produces and contains the pigment called melanin.
- melanoma
Cancer of the cells that produce pigment in the skin. Melanoma usually begins in a mole.
- metastasis
Cancer growth (secondary tumors) that is anatomically separated from the site at which the original cancer developed.
- metastasize
To spread from one part of the body to another. When cancer cells metastasize and form secondary tumors, the cells in the metastatic tumor are like those in the original (primary) tumor.
- mole
Area on the skin (usually dark in color) that contains a cluster of melanocytes. See also nevus.
- monoclonal
Population of cells that was derived by cell division from a single ancestral cell.
- morbidity
Disease.
- mortality
Death.
- mortality rate
Number of deaths per 100,000 persons per year.
- mutagen
Any substance that is known to cause mutations.
- mutagenesis
Process by which mutations occur.
- mutation
Change in the way cells function or develop, caused by an inherited genetic defect or an environmental exposure. Such changes may lead to cancer.
- National Cancer Institute (NCI)
The largest of the 24 separate institutes, centers, and divisions of the National Institutes of Health. The NCI coordinates the federal government's cancer research program.
- National Institutes of Health (NIH)
One of eight health agencies of the Public Health Service (the Public Health Service is part of the U.S. Department of Health and Human Services). Composed of 24 separate institutes, centers, and divisions, NIH is the largest biomedical research facility in the world.
- necrosis
Cell death.
- neoplasia
Abnormal new growth of cells.
- neoplasm
New growth of tissue. Can be referred to as benign or malignant.
- nevus
Medical term for a spot on the skin, such as a mole. A mole is a cluster of melanocytes that usually appears as a dark spot on the skin.
- non-Hodgkin lymphoma
One of the several types of lymphoma (cancer that develops in the lymphocytic system) that are not Hodgkin lymphoma. Hodgkin lymphoma is rare and occurs most often in people aged 15 to 34 and in people over 55. All other lymphomas are grouped together and called non-Hodgkin lymphoma.
- nonmelanoma skin cancer
Skin cancer that does not involve melanocytes. Basal cell cancer and squamous cell cancer are nonmelanoma skin cancers.
- Office of Science Education (OSE)
The Office of Science Education of the National Institutes of Health (NIH) coordinates science education activities at NIH and sponsors science education projects in-house.
- oncogene
Gene that normally directs cell growth but also can promote or allow the uncontrolled growth of cancer if damaged (mutated) by an environmental exposure to carcinogens or if damaged or missing because of an inherited defect.
- oncogenic
Having the capacity to cause cancer.
- oncologist
Doctor who specializes in treating cancer. Some oncologists specialize in a particular type of cancer treatment. For example, a radiation oncologist specializes in treating cancer with radiation.
- oncology
Study of tumors encompassing their physical, chemical, and biologic properties.
- oopherectomy
Surgical removal of one or both ovaries.
- p53
Gene that normally inhibits the growth of tumors, which can prevent or slow the spread of cancer.
- palliative treatment
Treatment that does not alter the course of a disease, but improves the quality of life.
- polyclonal
Population of cells that was derived by cell division from more than one ancestral cell.
- polyp
Mass of tissue that projects into the colon.
- precancerous
Term used to describe a condition that may or is likely to become cancer.
- precancerous polyp
Growths in the colon that often become cancerous.
- progesterone
Female hormone produced by the ovaries and placenta; responsible for preparing the uterine lining for implantation of an early embryo.
- prognosis
Probable outcome or course of a disease; the chance of recovery.
- promotion
Expression of the cancerous potential of initiated cells after exposure to the same or a different carcinogen.
- prophylactic
Treatment administered or taken to prevent disease.
- proto-oncogene
Gene that, when converted to an oncogene by a mutation or other change, can cause a normal cell to become malignant. Normal oncogenes function to control normal cell growth and differentiation.
- radiation therapy
Treatment with high-energy rays (such as X-rays) to kill cancer cells. The radiation may come from outside the body (external radiation) or from radioactive materials placed directly in the tumor (implant radiation). Also called radiotherapy.
- radioactive
Giving off radiation.
- radon
Radioactive gas that is released by uranium, a substance found in soil and rock. When too much radon is breathed in, it can damage lung cells and lead to lung cancer.
- relative risk
Comparison of the risk of developing cancer in persons with a certain type of exposure or characteristic with the risk in persons who do not have this exposure or characteristic.
- remission
Disappearance of the signs and symptoms of cancer. When this happens, the disease is said to be "in remission." A remission can be temporary or permanent.
- retinoblastoma
Eye cancer caused by the loss of both copies of the tumor suppressor gene RB; the inherited form typically occurs in childhood because one gene is missing from the time of birth.
- retrovirus
Small RNA virus that has an RNA genome. Acts as a template for the production of the DNA that is integrated into the DNA of the host cell. Many retroviruses are believed to be oncogenic.
- risk factor
Something that increases the chance of developing a disease.
- Rous sarcoma virus
Chicken retrovirus that was the first virus shown to cause a malignancy.
- sarcoma
Malignant tumor that begins in connective and supportive tissue.
- screening
Checking for disease when there are no symptoms.
- secondary tumor
Metastasis.
- SEER Program
Surveillance, Epidemiology, and End Results Program of the National Cancer Institute. Started in 1973, SEER collects cancer incidence data in nine geographic areas with a combined population of approximately 9.6 percent of the total population of the United States.
- side effect
Problem that occurs when treatment affects healthy cells. Common side effects of cancer treatment are fatigue, nausea, vomiting, decreased blood cell counts, hair loss, and mouth sores.
- somatic cell
Any of the body cells except the reproductive cells.
- SPF (sun protection factor)
Scale for rating sun-screens. Sunscreens with an SPF of 15 or higher provide the best protection from the sun's harmful rays.
- squamous cell cancer
Type of skin cancer that arises from the squamous cells.
- stage
Extent of a cancer, especially whether the disease has spread from the original site to other parts of the body.
- staging
Doing exams and tests to learn the extent of the cancer, especially whether it has spread from its original site to other parts of the body.
- stem cells
Cells from which all blood cells develop.
- sun protection factor
See SPF.
- sunscreen
Substance that blocks the effect of the sun's harmful rays. Using lotions or creams that contain sunscreens can protect the skin from damage that may lead to cancer. See also SPF.
- survival rate
Proportion of patients alive at some point after their diagnosis of a disease.
- telomerase
Enzyme that is present and active in cells that can divide without apparent limit (for example, cancer cells and cells of the germ line). Telomerase replaces the missing repeated sequences of each telomere.
- telomere
End of a chromosome. In vertebrate cells, each telomere consists of thousands of copies of the same DNA sequence, repeated again and again. Telomeres become shorter each time a cell divides; when one or more telomeres reaches a minimum length, cell division stops. This mechanism limits the number of times a cell can divide.
- testosterone
Male sex hormone.
- transformation
Change that a normal cell undergoes as it becomes malignant.
- tumor
Abnormal mass of tissue that results from excessive cell division. Tumors perform no useful body function. They may be either benign (not cancerous) or malignant (cancerous).
- tumor marker
Substance in blood or other body fluids that may suggest that a person has cancer.
- tumor suppressor gene
Gene in the body that can suppress or block the development of cancer.
- ultraviolet (UV) radiation
Invisible rays that are part of the energy that comes from the sun. UV radiation can burn the skin and cause melanoma and other types of skin cancer. UV radiation that reaches the earth's surface is made up of two types of rays, UVA and UVB rays. UVB rays are more likely than UVA rays to cause sunburn, but UVA rays pass further into the skin. Scientists have long thought that UVB radiation can cause melanoma and other types of skin cancer. They now think that UVA radiation also may add to skin damage that can lead to cancer. For this reason, skin specialists recommend that people use sunscreens that block or absorb both kinds of UV radiation.
- X-chromosome inactivation
Process by which one of the two X chromosomes in each cell from a female mammal becomes condensed and inactive. This process assures that most genes on the X chromosome are expressed to the same extent in both males and females.
- X-ray
High-energy radiation used in low doses to diagnose diseases and in high doses to treat cancer.
- xeroderma pigmentosum
Hereditary disease characterized by extreme sensitivity to the sun and a tendency to develop skin cancers. Caused by inadequate DNA repair.
- PubMedLinks to PubMed
- Understanding Cancer - NIH Curriculum Supplement SeriesUnderstanding Cancer - NIH Curriculum Supplement Series
- Chain F, Glutamate dehydrogenaseChain F, Glutamate dehydrogenasegi|747155622|pdb|4XGI|FProtein
- PDE7B-AS1 PDE7B antisense RNA 1 [Homo sapiens]PDE7B-AS1 PDE7B antisense RNA 1 [Homo sapiens]Gene ID:644135Gene
- Gm9946 predicted gene 9946 [Mus musculus]Gm9946 predicted gene 9946 [Mus musculus]Gene ID:330403Gene
- CFAP20DC CFAP20 domain containing [Homo sapiens]CFAP20DC CFAP20 domain containing [Homo sapiens]Gene ID:200844Gene
Your browsing activity is empty.
Activity recording is turned off.
See more...