NCBI Bookshelf. A service of the National Library of Medicine, National Institutes of Health.
Jamison DT, Breman JG, Measham AR, et al., editors. Disease Control Priorities in Developing Countries. 2nd edition. Washington (DC): The International Bank for Reconstruction and Development / The World Bank; 2006. Co-published by Oxford University Press, New York.

Helminth infections caused by soil-transmitted helminths (STHs) and schistosomes are among the most prevalent afflictions of humans who live in areas of poverty in the developing world. The morbidity caused by STHs and schistosomes is most commonly associated with infections of heavy intensity. Approximately 300 million people with heavy helminth infections suffer from severe morbidity that results in more than 150,000 deaths annually (Crompton 1999; Montresor and others 2002). In addition to their health effects, helminth infections also impair physical and mental growth in childhood, thwart educational advancement, and hinder economic development. Because of the geographic overlap of these afflictions and their impact on children and adolescents, the World Health Organization (WHO); the World Bank; and other United Nations agencies, bilaterals, and civil society are working to integrate STH and schistosome control through a program of periodic school-based, targeted anthelmintic drug treatments.
Causes and Characteristics of Helminth Infections
Emphasis is placed on the four most common STH infections and the three most common schistosome infections. Together, these infections account for most of the global helminth disease burden.
Soil-transmitted Helminths
The four most common STHs are roundworm (Ascaris lumbricoides), whipworm (Trichuris trichiura), and the anthropophilic hookworms (Necator americanus and Ancylostoma duodenale). Recent estimates suggest that A. lumbricoides infects 1.221 billion people, T. trichiura 795 million, and hookworms 740 million (de Silva and others 2003) (table 24.1). The greatest numbers of STH infections occur in the Americas, China and East Asia, and Sub-Saharan Africa. Strongyloides stercoralis is also a common STH in some of these regions, although detailed information on the prevalence of strongyloidiasis is lacking because of the difficulties in diagnosing human infection. The life cycles of Ascaris, Trichuris, and hookworm follow a general pattern. The adult parasite stages inhabit the gastrointestinal tract (Ascaris and hookworm in the small intestine; Trichuris in the colon), reproduce sexually, and produce eggs, which are passed in human feces and deposited in the external environment.
Table 24.1
Global Prevalence and Distribution of Helminth Infections.
STH infections rarely cause death. Instead, the burden of disease is related less to mortality than to the chronic and insidious effects on the hosts' health and nutritional status (Stephenson, Latham, and Ottesen 2000; Stoltzfus and others 1997). Hookworms have long been recognized as an important cause of intestinal blood loss leading to iron deficiency and protein malnutrition. The iron deficiency anemia that accompanies moderate and heavy hookworm burdens is sometimes referred to as hookworm disease (Hotez and others 2004). When host iron stores are depleted, the extent of iron deficiency anemia is linearly related to the intensity of hookworm infection (Stoltzfus and others 1997). Because of their underlying poor iron status, children, women of reproductive age, and pregnant women are frequently the ones most susceptible to developing hookworm anemia (Brooker, Bethony, and Hotez 2004). Iron deficiency anemia during pregnancy has been linked to adverse maternal-fetal consequences, including prematurity, low birth-weight, and impaired lactation (WHO 2002).
Chronic STH infections resulting from Ascaris, Trichuris, and hookworm can dramatically affect physical and mental development in children (WHO 2002). Studies have also shown that the growth and physical fitness deficits caused by chronic STH infections are sometimes reversible following treatment with anthelmintic drugs (Stephenson, Latham, and Ottesen 2000). The effects on growth are most pronounced in children with the heaviest infections, but light infections may also contribute to growth deficits if the nutritional status of the community is poor (Stephenson, Latham, and Ottesen 2000).
Schistosomiasis
Five major species of parasitic trematodes of the family Schistosomatidae—Schistosoma haematobium, S. intercalatum, S. japonicum, S. mansoni, and S. mekongi—infect humans. These parasites have a complex, indirect life cycle involving an intermediate snail host. Disease is caused primarily by schistosome eggs, which are deposited by adult worms in the blood vessels surrounding the bladder or intestines. Urinary schistosomiasis, in which the bladder is affected, is caused by infection with S. haematobium, which occurs mainly in Africa. Intestinal schistosomiasis results from infection with S. mansoni, which occurs in the Middle East, South America, and Africa, and from infection with S. japonicum, which occurs in parts of China and the Philippines (Ross and others 2002). Two other schistosome species are known to cause intestinal schistosomiasis in restricted geographical areas: S. intercalatum, found in Central Africa, and S. mekongi, found in Cambodia and the Lao People's Democratic Republic. Schistosomiasis is estimated to affect 187 million people worldwide (table 24.1).
A serious acute illness accompanied by fever and lymphadenopathy, known as Katayama Syndrome, can result from heavy schistosome infections. Chronic disease is mostly due to perforation of blood vessels and entrapment of eggs by host tissues. The host's reaction to entrapped eggs results in granuloma formation. S. haematobium causes bladder wall pathology, leading to ulcer formation, hematuria, and dysuria. Granulomatous changes and ulcers of the bladder wall and ureter can lead to bladder obstruction, dilatation, secondary urinary tract infections and subsequent bladder calcification, renal failure, lesions of the female and male genital tracts, and hydronephrosis. S. haematobium is also associated with increased risk of bladder cancer. The morbidity commonly associated with S. mansoni infection includes lesions of the liver, portal vein, and spleen, leading to periportal fibrosis, portal hypertension, hepatosplenomegaly, splenomegaly, and ascites. Schistosomiasis also causes chronic growth faltering and can contribute to anemia (Ross and others 2002).
Epidemiology of STH Infections and Schistosomiasis
The most striking epidemiological features of human helminth infections are aggregated distributions in human communities, predisposition of individuals to heavy (or light) infection, rapid reinfection following chemotherapy, and age-intensity profiles that are typically convex (with the exception of hookworm).
For all the major human STH and schistosome infections studied to date, worm burdens exhibit a highly aggregated (overdispersed) distribution so that most individuals harbor just a few worms in their intestines, although a few hosts harbor disproportionately large worm burdens (Anderson and May 1991). As a rule, 20 percent of the host population harbors approximately 80 percent of the worm population. This overdispersion has many consequences, both with regard to the population biology of the helminths and the public health consequence for the host, because heavily infected individuals are simultaneously at highest risk of disease and the major source of environmental contamination. One feature that may help explain overdispersion is that individuals tend to be predisposed to heavy (or light) infections. Predisposition has been demonstrated for all four major STHs and the schistosomes. The underlying cause of such predisposition remains poorly understood. However, a combination of heterogeneity in exposure to infection or differences in susceptibility to infection and the ability to mount effective immunity (genetic and nutritional factors) is likely to be important.
People of all ages rapidly reacquire infection following treatment, but in schistosomiasis, older people reacquire infection at slower rates than younger ones (Kabatereine and others 1999). The rate of reinfection is specific to certain species of helminths and depends on the life expectancy of that species (short-lived helminths reinfect more rapidly), on the intensity of transmission within a given community, and on the treatment efficacy and coverage. The basic reproductive rate (Ro)describes the transmission potential of a parasite (and thus its ability to reinfect the host). It defines the average number of female offspring produced during the life span of the parasite that survive to reproductive maturity in the absence of density dependence.Ro is determined by parasite immigration anddeath rates as well as by host density (and, in schistosomiasis, also snail density). A parasite will fail to become established unless Ro is greater than unity (Anderson and May 1991). Adult worms usually survive between one and four years, whereas eggs can sometimes remain viable for several more years in the environment. Therefore, reinfection rates will remain high until adults are removed with chemotherapy and until infective stages, through time, become uninfective. In reality, density-dependent processes regulate parasite populations; at endemic equilibrium, the effective reproductive ratio equals unity (that is, each female replaces herself). Control programs rely on reducing the effective reproductive ratio long enough for the parasite population to be driven to local elimination. Theoretically,Ro provides useful insights, and it is helpful to think of control programs attempting to break the transmission cycle by reducing to less than unity. Therefore,Ro estimates can be made about how long and how many rounds of chemotherapy are required to treat intestinal helminths. For example, A. lumbricoides with an of three and a life Roexpectancy of one year will need to be treated annually with a drug that is 95 percent efficacious and with coverage of more than 91 percent of the population. Where Ro is five—that is,in areas where transmission is higher—treatment must be given more frequently than once a year (Anderson and May 1991).
The age-dependent patterns of infection prevalence are generally similar among the major helminth species, exhibiting a rise in childhood to a relatively stable asymptote in adulthood (figure 24.1). Maximum prevalence of A. lumbricoides and T. trichiura is usually attained before five years of age, and the maximum prevalence of hookworm and schistosome infections is usually attained in adolescence or in early adulthood. The nonlinear relationship between prevalence and intensity has the consequence that the observed age-prevalence profiles provide little indication of the underlying profiles of age intensity (age in relation to worm burden). Because intensity is linked to morbidity, the age-intensity profiles provide a clearer understanding of which populations are vulnerable to the different helminths (figure 24.1). For A. lumbricoides and T. trichiura infections, the age-intensity profiles are typically convex in form, with the highest intensities in children 5 to 15 years of age (Bundy 1995). For schistosomiasis, a convex pattern is also observed, with a similar peak but with a plateau in adolescents and young adults 15 to 29 years of age (Kabatereine and others 1999). In contrast, the age-intensity profile for hookworm exhibits considerable variation, although intensity typically increases with age until adulthood and then plateaus (Brooker, Bethony, and Hotez 2004). In East Asia it is also common to find the highest intensities among the elderly. However, more generally, children and young adults are at higher risk of both harboring higher levels of infection (thus greater levels of morbidity) and becoming reinfected more quickly. Both may occur at vital stages in a child's intellectual and physical development.

Figure 24.1
Age-associated Prevalence and Intensity Profiles of STH and Schistosome Infections: Typical Age Profiles of Prevalence and Intensity of STH Infections and Schistosomiasis
Risk Factors
Both host-specific and environmental factors have been identified that may affect the risk of acquiring or harboring heavy-intensity helminth infections.
Genetics
No genes that control for human helminth infection have yet been identified. However, recent genome scans have identified a locus possibly responsible for controlling S. mansoni infection intensity on chromosome 5q31–33 and loci controlling A. lumbricoides intensity on chromosomes 1 and 13. There is also evidence for genetic control of pathology attributable to S. mansoni, with linkage reported to a region containing the gene for the interferon gamma receptor 1 subunit (Quinnell 2003).
Behavior, Household Clustering, and Occupation
Specific occupations, household clustering, and behaviors influence the prevalence and intensity of helminth infections (Bethony and others 2001), particularly for hookworm, in which the highest intensities occur among adults (Brooker, Bethony, and Hotez 2004). Engagement in agricultural pursuits, for example, remains a common denominator for hookworm infection. Behavioral and occupational factors, through their effect on water contact, interact with environmental factors to produce variation in the epidemiology of schistosomiasis.
Poverty, Sanitation, and Urbanization
STH and schistosomiasis depend for transmission on environments contaminated with egg-carrying feces. Consequently, helminths are intimately associated with poverty, poor sanitation, and lack of clean water. The provision of safe water and improved sanitation are essential for the control of helminth infection. Although the STH and schistosome infections are neglected diseases that occur predominantly in rural areas, the social and environmental conditions in many unplanned slums and squatter settlements of developing countries are ideal for the persistence of A. lumbricoides (Crompton and Savioli 1993). Schistosomiasis transmission can also occur in urban areas.
Climate, Water, and Season
Adequate warmth and moisture are key features for each of the STHs. Wetter areas exhibit increased transmission, and in some endemic areas, both STH and schistosome infections exhibit marked seasonality (Brooker and Michael 2000). Recent use of geographical information systems and remote sensing has identified the distributional limits of STH and schistosomes on the basis of temperature and rainfall patterns (Brooker and Michael 2000). For schistosomiasis, specific snail intermediate hosts prefer certain types of aquatic environments. Construction of dams is known to extend the range of snail habitats, thereby promoting the reemergence of schistosomiasis.
Burden of the Disease
The revised estimates in 2003 (de Silva and others 2003) use the methodology developed by Chan and others (1994) and build on recent applications of geographical information systems to derive updated atlases of helminth infections. To reflect recent changes in the epidemiology of infection, de Silva and others used data from only 1990 onward. These data confirm that STH infections are the most prevalent infections of humans and that a large proportion of the population in developing countries is at risk. Of the 187 million cases of schistosomiasis estimated to occur worldwide, most are caused by S. haematobium in Sub-Saharan Africa (table 24.1).
WHO (2002) estimates that 27,000 people die annually from STH infections and schistosomiasis (case fatality rate of 0.0014 percent). Many investigators, however, believe that this figure is an underestimate. Crompton (1999) estimated that 155,000 deaths annually occur from these infections (case fatality rate of 0.08 percent), whereas Van der Werf and others (2003), using the limited data available from Africa, estimated the schistosomiasis mortality alone at 280,000 per year (case fatality rate of 0.014 percent) because of nonfunctioning kidneys (from S. haematobium) and hematemesis (from S. mansoni). Therefore, the difference between estimates for helminth-associated mortality is more than 10-fold.
Because it is uncommon for STHs and schistosomes to kill their human host, citing mortality figures provides only a small window on their health impact. Instead, measurements of disease burden using disability-adjusted life years (DALYs) and similar tools portray a more accurate picture for helminthic disease burden. WHO estimates the global burden of disease from STH infections and schistosomiasis on the basis of the enormous number of infected individuals, together with an associated low disability weight (Van der Werf and others 2003). However, because an estimated 2 billion people are infected with STHs and schistosomes, even minor adjustments to the disability weights produce enormous variations in DALYs or other measurements of disease burden. This helps to explain why, for instance, in 1990 the disease burden for the STH infections and schistosomiasis was almost 18 million DALYs, whereas the 2001 estimate was only 4.7 million DALYs. In the intervening 11 years, the DALYs were as low as 2.6 million. Such disparities are substantial when one considers that the 1990 estimate ranks helminths close to major disease entities such as tuberculosis, measles, and malaria, whereas the lowest estimate during the 1990s ranks helminth infections on a par with gonorrhea, otitis media, and iodine deficiency. The Disease Control Priorities Project helminth working group has determined that the WHO global burden of disease estimates are low because they do not incorporate the full clinical spectrum of helminth-associated morbidity and chronic disability, including anemia, chronic pain, diarrhea, exercise intolerance, and undernutrition (King, Dickman, and Tisch 2005). However, for this chapter, the average disability weights estimated by WHO are used throughout. Some of the specific controversies are described below.
A. lumbricoides and T. trichiura Infections
Because the most significant physical and intellectual growth disturbances occur as a consequence of moderate and heavy worm burdens, the age-associated epidemiology of A. lumbricoides and T. trichiura infections has focused attention on infected school-age children in developing countries (Bundy 1995). In a revised estimate of the probable number of ascariasis infections worldwide and a better categorization of the morbidity, de Silva, Chan, and Bundy (1997) indicated that 59 million of the 1.2 billion people infected (including 51 million children less than 15 years of age) were at risk of faltering growth, decreased physical fitness, or both as a result of infection. They estimated that about 1.5 million children would never make up the deficit in growth, even if treated. In addition to these chronic, insidious effects, they estimated that about 11.5 million individuals (almost all of them children) were at risk of more acute clinical illness. Their figures also indicated that at least 10,500 deaths annually were directly attributable to one of the serious complications of ascariasis; children account for more than 90 percent of those deaths. The actual threshold at which A. lumbricoides and T. trichiura worm burdens result in childhood morbidity is controversial because of the nonlinear relationship between intensity and pathogenesis and the difficulties of measuring and attributing morbidity in under-served populations suffering from other underlying conditions (Bundy 1995).
Hookworm Infection
Hookworm infection causes more DALYs lost than any other helminthiasis with the exception of lymphatic filariasis. Even these DALY measurements may still underestimate the true disease burden of iron deficiency anemia and protein malnutrition resulting from hookworm disease. Iron deficiency anemia alone results in approximately 12 million DALYs lost annually, making it the world's most important nutrition problem. Data on the epidemiology of iron deficiency anemia in East Africa and elsewhere point to the important contribution of hookworms to this condition (Stoltzfus and others 1997). In Tanzania, where hosts' iron stores are often depleted, there is a correlation between the number of adult hookworms in the intestine and the amount of host blood loss (Stoltzfus and others 1997). However, it is unclear whether current disability weights effectively incorporate the full contribution of hookworm to severe iron deficiency anemia among iron-depleted populations or whether they take host protein losses and malnutrition into account. There is increasing interest in the importance of hookworm anemia in preschool children, especially in Africa (Brooker, Bethony, and Hotez 2004), where infants and preschool children are particularly vulnerable to the developmental and behavioral deficits caused by iron deficiency anemia (Stephenson, Latham, and Ottesen 2000). Closer assessment of the impact of hookworm on another important iron-deficient population—namely, women of reproductive age—could also significantly increase current DALY estimates. Approximately 44 million of these women harbor hookworms (Bundy, Chan, and Savioli 1995). In addition, severe anemia in pregnancy is associated with neonatal prematurity, reduced birthweight, and impaired lactation (Christian, Khatry, and West 2004).
Schistosomes
Scientists and public health workers disagree on the current assessments of both morbidity and mortality attributable to schistosomiasis. Several investigators have now initiated a process to recalculate the burden of disease attributable to schistosomiasis, focusing much more on the clinical course of the different types of schistosomiasis and chronic sequelae (King, Dickman, and Tisch 2005; Michaud, Gordon, and Reich 2003). Through a comprehensive literature review combined with mathematical modeling, Van der Werf and others (2003) estimate that urinary schistosomiasis in Africa results in approximately18 million cases of bladder wall pathology and 20 million cases of hydronephrosis, and African intestinal schistosomiasis results in approximately 8.5 million cases of hepatomegaly. Mortality in Africa attributable to urinary and intestinal schistosomiasis was extrapolated from these figures using a limited number of studies reporting case fatality rates for nonfunctioning kidney and hematemesis. From these extrapolations, Fenwick and others (2003) conclude that in Africa the mortality attributable to urinary schistosomiasis could be as high as 150,000 per year, and the number dying as a result of intestinal schistosomiasis could be as high as 130,000 per year.
Cost-Effectiveness Analysis of Interventions
Classifying Interventions
The three major interventions are anthelmintic drug treatment, sanitation, and health education.
Anthelmintic Drug Treatment
Anthelmintic drug treatment ("deworming") is aimed at reducing morbidity by decreasing the worm burden. Repeated chemotherapy at regular intervals (periodic deworming) in high-risk groups can ensure that the levels of infection are kept below those associated with morbidity (figure 24.2) and will frequently result in immediate improvement in child health and development. Anthelmintic drug treatment can prevent the development of irreversible consequences of schistosomiasis in adulthood. For ascariasis and trichuriasis, for which intensity peaks among school-age children, frequent and periodic deworming may reduce transmission over time. Obstacles that diminish the effectiveness of periodic deworming are the low efficacy of single-dose mebendazole and albendazole for the treatment of hookworm and trichuriasis, respectively (Adams and others 2004; Albonico and others 1994); high rates of posttreatment reinfection for STHs in areas of high endemicity (Albonico and others 1995); and diminished efficacy with frequent and repeated use (Albonico and others 2003), possibly because of anthelmintic resistance (see the section "Research and Development").
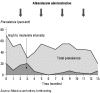
Figure 24.2
Predicted Effect on Ascaris and Trichuris Prevalence following Frequent and Periodic Dewormings with Benzimidazole Anthelmintics
Improved Sanitation
Improved sanitation is aimed at controlling transmission by reducing soil and water contamina tion. Sanitation is the only definitive intervention to eliminate STH infections, but to be effective it should cover a high percentage of the population. Therefore, because of the high costs involved, implementing this strategy is difficult where resources are limited (Asaolu and Ofoezie 2003). Moreover, when used as the primary means of control, it can take years or even decades for sanitation to be effective (Brooker, Bethony, and Hotez 2004).
Health Education
Health education is aimed at reducing transmission and reinfection by encouraging healthy behaviors. For STH infections and schistosomiasis, the aim is to reduce contamination of soil and water by promoting the use of latrines and hygienic behavior. Without a change in defecation habits, periodic deworming cannot attain a stable reduction in transmission. Health education can be provided simply and economically and presents no contraindications or risks. Furthermore, its benefits go beyond the control of helminth infections. In this perspective, it is reasonable to include this component in all helminth control programs.
Other Control Measures
In specific epidemiological conditions, environmental or chemical control of snails can be useful tools for reducing the transmission of schistosomiasis. Research to develop new tools for control is in progress, including vaccine development programs for hookworm infection and schistosomiasis (see "Research and Development").
Choosing Interventions
Periodic deworming stands out as the most cost-effective means to reduce the morbidity of STH and schistosome infections.
Periodic Anthelmintic Therapy
Periodic anthelmintic therapy, or periodic deworming, represents the main measure in areas where infections are intensely transmitted, resources for disease control are limited, and funding for sanitation is lacking. Drug treatment can be administered in the community using different strategies:
- Universal treatment. The entire community is treated, irrespective of age, sex, infection status, and other characteristics.
- Targeted treatment. Treatment targets population groups, which may be defined by age, sex, or other social characteristics, irrespective of the infectious status.
- Selective treatment. Treatment targets individual-level application of anthelmintic drugs, which is selected on the basis of either diagnosis or a suspicion of current infection.
Recommended drugs for use in public health interventions to control STH infection are the benzimidazole anthelmintics (BZAs), albendazole (single dose: 400 mg, reduced to 200 mg for children between 12 and 24 months), or mebendazole (single dose: 500 mg), as well as levamisole or pyrantel pamoate (WHO 2002). Praziquantel (PZQ) (single dose: 40–60 mg/kg) is the major drug used for the treatment of schistosomiasis. However, therapy with oxamniquine has been the cornerstone for treatment of S. mansoni infection in South American national control programs over the past 20 years. The efficacy of oxamniquine and PZQ is comparable, although that of PZQ is slightly better. The BZAs and PZQ are inexpensive; they have undergone extensive safety testing and have been used by millions of individuals with only a few minor side effects. Drugs that do not need dosage according to weight, such as BZAs (in school-age children), are considered easier to use for population-based interventions; however, the use of proxy indicators—for example, substituting height for weight—has proved a successful implementation strategy for PZQ (Hall and others 1999).
Distribution Strategy and Frequency of Treatment
The selection of the distribution strategy and the frequency of treatment is based on epidemiological data. The recommended strategy for helminth control is a population-based approach, in which individuals in targeted communities are treated irrespective of their infection status (WHO 2002). This strategy is justified for several reasons, including the simplicity and safety of delivering treatment. Individual diagnosis is difficult and expensive and offers no safety benefit.
The intrinsic transmission potential of the parasite species determines the frequency of treatment (see "Epidemiology of STH Infections and Schistosomiasis," earlier in this chapter). To control morbidity in areas of intense transmission (prevalence greater than 70 percent and more than 10 percent of moderate-and heavy-intensity infection), WHO (2002) recommends treatment two or three times a year for STH infections. In areas with a lower intensity of transmission (prevalence between 40 and 60 percent and less than 10 percent of moderate- and heavy-intensity infection), intervention once a year is recommended (WHO 2002).
School-Age Children as a High-Risk Population
School-age children typically have the highest intensity of worm infection of any age group, and chronic infection negatively affects all aspects of children's health, nutrition, cognitive development, learning, and educational access and achievement (World Bank 2003). Regular deworming can cost-effectively reverse and prevent much of this morbidity. Furthermore, schools offer a readily available, extensive, and sustained infrastructure with a skilled workforce that is in close contact with the community. With support from the local health system, teachers can deliver the drugs safely. Teachers need only a few hours of training to understand the rationale for deworming and to learn how to give out the pills and keep a record of their distribution. School-based deworming also has major externalities for untreated children and the whole community. By reducing transmission in the community of Ascaris and Trichuris infections, deworming substantially improves the health and school participation of both treated and untreated children, both in treatment schools and in neighboring schools (Bundy and others 1990; Miguel and Kremers 2003).
These observations provided a basis for the adoption of resolution 54.19 at the 2001 World Health Assembly, which urged member states to ensure access to essential drugs for STH and schistosome infections in endemic areas for the treatment of clinical cases and groups at high risk for morbidity (box 24.1). To achieve this goal, WHO has developed a broad partnership that promotes the incorporation of deworming into existing institutions and programs, for both the education sectors and the health sectors. The Partnership for Parasite Control was launched in 2001 with the aim of mobilizing resources and promoting synergy among public and private efforts for the control of soil-transmitted helminths and schistosomiasis at global and national levels. School-based deworming has its full effect when delivered within an integrated school health program that includes elements of the Focusing Resources on Effective School Health (FRESH) framework.
Other At-Risk Populations
Not only school-age children can benefit from treatment. Preschool children (one to five years of age) are vulnerable to the developmental and behavioral deficits caused by iron deficiency anemia, and recent analyses by Brooker, Bethony, and Hotez (2004) indicate that hookworm is an important contributor to anemia in that age group (see "Estimating Intervention Effectiveness"). Women of reproductive age (15 to 49 years of age) are particularly susceptible to iron deficiency anemia because of iron loss during menstruation and because of increased needs during pregnancy (Bundy, Chan, and Savioli 1995). In certain circumstances, male worker populations can also be at increased risk (Guyatt 2000).
Estimating Intervention Effectiveness
The evidence base for the health and educational effect of periodic deworming has accumulated significantly over the past decade.
STH Infections
All the anthelmintic drugs mentioned above substantially reduce the number of adult worms in the gastrointestinal tract. This effect is also reflected in reduced fecal egg counts. In some cases, however, the efficacy of single-dose mebendazole or albendazole on hookworm and Trichuris infections is low (Adams and others 2004; Albonico and others 1994). Moreover, pyrantel pamoate has little effect on T. trichiura. Overall, however, anthelmintic treatment significantly improves physical and cognitive outcomes in the following ways:
- Preschool children. Periodic distribution of anthelmintics has a positive effect on motor and language development and reduces malnutrition in very young children (Stoltzfus and others 2004).
- School-age children. Treating school-age children has a considerable effect on their nutritional status (Stoltzfus and others 2004), anemia, physical fitness, appetite, growth (Stephenson, Latham, and Ottesen 2000), and intellectual development (Drake and others 2000).
- Women of reproductive age. Studies of pregnant women conducted by Christian, Khatry, and West (2004) in Nepal indicate that albendazole treatment improves maternal hemoglobin as well as birth-weight and child survival.
Schistosomiasis
As with STH infections, anthelmintic chemotherapy for schistosomiasis has an important effect on child development, growth, and physical fitness (WHO 2002). Richter (2003) recently summarized details of the effect of PZQ on organ pathology. In S. haematobium infections, reversal of urinary tract pathology can be seen six months after a cure. In S. mansoni and S. japonicum infections, much of the intestinal pathology regresses after chemotherapy. However, more than one PZQ treatment is usually necessary to reverse hepatic pathology, especially in areas of intense transmission. Early intervention with PZQ is preferable to reverse organ pathology.
Intervention Costs
Several studies have evaluated the costs of school-based periodic deworming in several different settings, whereas comparable studies on other interventions are still lacking.
Periodic Deworming
The advantage of periodic deworming lies in its simplicity (one tablet per child) and safety. Teachers and other personnel without medical training can easily apply the simple measures, which can be incorporated without difficulty in existing health and nonhealth activities that reach the high-risk group. Several organizations, including nongovernmental organizations, include an STH and schistosome infection-control package within their routine activities and, with very limited budgets, relieve the burden of helminth infections in the population covered. The costs of albendazole and PZQ are available through the International Drug Price Indicator Guide (http://www.msh.org). Delivery systems for deworming have often depended on vertical programs, in which mobile teams visit schools or communities to carry out treatment (WHO 2002). Estimated costs for this approach are outlined in table 24.2. For STH infections in Tanzania, Nigeria, and Montserrat, the costs range from US$0.21 to US$0.51 per treatment. However, by training teachers and other school officials to administer anthelmintic drugs, the system could achieve low-cost delivery by "piggy-backing" on existing programs in the educational sector (WHO 2002). Specific examples of such programs conducted in Ghana and Tanzania are summarized in the section "Implementation of Control Strategies: Lessons of Experience," later in this chapter. It was found that delivery of school-based targeted anthelmintic treatment could cost as little as US$0.03 per child, which may be as low as one-tenth of the estimated costs for vertical delivery (WHO 2002). Thus, at current drug prices, the total cost (drug plus delivery) of a single treatment with albendazole or mebendazole may be as low as US$0.05, and that of a combined treatment with PZQ may be as low as US$0.25 per child (WHO 2002).
Table 24.2
Recent Examples of Delivery Costs for a Single Mass Treatment.
Integrating drug distribution through the school system rather than using mobile teams, along with a marked decline in the price of BZAs and PZQ, has resulted in a 10-fold reduction in delivery costs. However, those costs are artificially low because they do not include the external costs for the coordinating center responsible for supporting those approaches (Guyatt 2003). It has been estimated, for instance, that mass albendazole treatment of school-age children in Kenya could cost more than US$3 million each year, equivalent to some 4 percent of current national public expenditure on health care (Guyatt 2003). This analysis has not been evaluated against actual operations, however, and current estimates from the parasite control authorities in Kenya suggest that the actual cost is likely to be far less. Large-scale chemotherapy programs for helminth control continue to rely heavily on donor support, suggesting that some affected countries may be unable to support the costs of deworming.
Monitoring of control programs is an important part of the managerial process, and it should be carried out at minimum cost so as not to divert resources from the intervention (Brooker and others 2004). It is recommended that, at the planning stage, approximately 5 to 10 percent of the program budget be reserved for monitoring activities (Montresor and others 2002).
Improved Sanitation
When sanitation improvements are made alongside deworming, the results obtained last longer. However, the investment needed to reach the level required to interfere with STH transmission could be high. To correctly evaluate the advantage of such investments, one must take into account the consequences for other health indicators and for economic development. An efficient sanitation infrastructure removes the underlying cause of most poverty-related communicable diseases and can boost the economic development of a country. The resources needed to improve hygienic standards can be huge and require the cooperation of several sectors of society (Asaolu and Ofoezie 2003). Currently, these are qualitative judgments, and no cost-effectiveness analysis (CEA) estimates exist for sanitation in this context.
Health Education and Communication
Measures to increase the health awareness of the population are included as an essential component of any population-based activity aimed at controlling morbidity attributable to helminth infections. However, the effectiveness of those activities in reducing transmission of infection varies according to different reports. In some cases, health education can decrease costs, increase levels of knowledge, and decrease reinfection rates (Lansdown and others 2002). Health education efforts can build trust and engage communities, aspects that are crucial to the success of public health initiatives. No CEA estimates exist for health education in this context.
Linking Costs and Effects of Interventions
Interventions to reduce morbidity from helminth infections fall into two categories: targeting the transmission mechanisms and treating individuals directly. The former encompasses improvements in infrastructure, including water supply and sanitation, and health education. The latter entails the periodic drug treatment of the population. Substantial improvements through prevention may be a long-term outcome of economic growth in general, because wealthier households have improved sanitation facilities and practices, but those improvements are not an option in the short term without large investments in infrastructure. As shown in the previous section, deworming options dominate on both effectiveness and cost-effectiveness criteria. Costs continue to fall as drug costs decrease. With better data and detailed mapping of disease distribution within communities, targeting individuals at high risk becomes more feasible, thus improving the cost-effectiveness of control programs (Michaud, Gordon, and Reich 2003).
Evidence from existing programs that narrow the intervention to school-age children (a high-risk group) shows that the treatment costs of chemotherapy for helminth infections are quite low—well below US$1 per school-age child. This finding is in part due to the accessibility of the target group and the cost savings of incorporating delivery into existing school and health programs. Moreover, as discussed in the following sections, the economic benefits of targeting this group may be substantial. Still other targeted groups may also have low cost per treatment when treatment is merged into existing programs. For example, interventions through prenatal care programs for pregnant women may be cost-effective. Likewise, evidence on costs of treatment through existing integrated management of infant and childhood illness (IMCI) programs for small children and health campaigns (such as vaccination and micronutrient programs) find low cost per case treated (Montresor and others 2002).
Several factors can potentially alter the ranking of interventions in regard to cost-effectiveness, although there are no existing studies to evaluate this. Previous analysis may underestimate the effectiveness and overestimate the cost-benefit ratios of mass treatment of school-age children if the externalities of treatment are not considered (Miguel and Kremer 2003). The cost-effectiveness of school-based deworming programs will change as the programs are extended to cover children who are not enrolled in school. Such program extensions are likely to have greater costs because they entail additional staff and outreach efforts per case treated. However, the effectiveness of mass treatment of school-age children (both enrolled and not enrolled) may be greater. Children who are not enrolled in school come from households with lower income levels. Lower income, which leads to poorer sanitation conditions, is associated with greater incidence and intensity of infections. Expanding mass treatment to children not enrolled in school will result in treating populations that have higher incidence and intensity, thus raising effectiveness (box 24.2).
Distributional and Equity Consequences
Interventions to control helminth infections can have equity implications in several dimensions. Programs designed to target communities with high prevalence or high intensity of helminth infection focus on areas with lower income, as described in the sections on the causes, characteristics, and epidemiology of such infections. Although no studies undertake benefit-incidence analysis of public spending on such health services, this targeting implies that state subsidies on deworming services will be of most benefit to lower-income groups. With the increasing availability of poverty maps, empirical evaluation of the equity implications of deworming will be feasible.
Averted, Avertable, and Nonavertable Burden
In the short run, deworming can avert helminth infections. In the long run, it is assumed that as income levels grow and infrastructure improves, the number of infections averted by reducing transmission will increase. However, given the slow rate of poverty reduction in the 1990s for the poorest regions, such as Sub-Saharan Africa, waiting for economic development to lead to a reduction in infections is only, at best, a slow-paced solution for the majority of the infected population. It is more likely that most averted infections will depend on periodic deworming. Thus, the question remains as to what portion of existing infections is potentially avertable through recommended interventions and what portion is currently averted with existing programs. For schistosomiasis, successful programs in several countries, including Brazil, China, and the Arab Republic of Egypt, and the issues related to the sustainability of these successes have been described (see "Implementation of Control Strategies: Lessons of Experience" later in this chapter). However, the number of averted schistosomiasis infections in Sub-Saharan Africa is likely to be small, because few serious attempts at widespread control have been made in recent years, and not much of the burden of STH and schistosome infections is currently averted through private treatment. In part, the low number of averted infections may be due to the lack of information on the part of infected individuals, the insidious nature of the condition, and the lack of drugs in the public or private health delivery system.
Economic Benefits of Intervention
The characteristics of helminth infections make a compelling case for public sector intervention if based only on the evidence related to the intervention's effect on health. From an economic perspective, the public sector has several reasons to become involved in improving health outcomes. First, other benefits may be gained, in addition to the benefit for the treated individual. Second, some forms of intervention are almost pure public goods; that is, no one can be excluded from using the goods or services the interventions deliver, and the private sector is thus unlikely to deliver them. Finally, preventive measures, such as information on the value of washing hands,may not be delivered through the private sector. The lack of knowledge about infections and subclinical symptoms may make individuals less likely to seek treatment. In analyzing the gains of interventions for worm control, one should account for the burden of helminth infections, which extends well beyond the health impacts and DALYs. The economic implications may be quite large.
The negative correlation between helminth infections and income level is clearly demonstrated both within and between countries (de Silva and others 2003). However, causality cannot be inferred from this established relationship; poverty promotes higher worm burdens, yet poor health induced by helminths can lead to lower income. There may also be opportunity costs to uninfected household members residing with infected persons. Few studies have been designed to evaluate, either directly or indirectly, the magnitude of the effect of deworming on economic productivity. The indirect evidence at the micro level suggests that helminth infection has a significant impact on adult productivity and, subsequently, on earnings. More direct evidence for children shows that helminth infection has long-term implications for educational attainment and economic status.
Studies are increasingly documenting a causal impact of adult health (broadly defined) on labor force participation, wages, and productivity in developing countries (Thomas and Strauss 1997). Moreover, helminth infection is known to affect some of the health conditions related to productivity—namely, iron deficiency anemia and wasting. Guyatt (2000) reviews numerous studies relating these conditions to physical fitness and productivity; Haas and Brownlie (2001) review studies on the effect of iron supplementation on work. The studies generally show productivity gains linked to better health along the various health dimensions studied. However, although some evidence points to the indirect impact of STHs on income earnings, these relationships have not been adequately studied, either directly or indirectly.
More compelling links between helminth infection and economic well-being may exist for children. The strong association between worm burden and poor health outcomes for children suggests that infections may affect school enrollment, attendance, grade repetition, and grade attainment. In turn, the potential impact on educational outcomes has implications for the assessment of the economic benefits of intervention. Numerous studies have demonstrated the benefits of schooling, showing that the return on education is quite high. Increased education is associated with, among other things, higher worker productivity and generally higher productivity in nonmarket production activities, including greater farmer efficiency and productivity (Psacharopoulos and Patrinos 2002).
Although observational studies show that lower levels of learning and schooling are linked to helminth infection (World Bank 2003), establishing a causal relationship requires adequately controlling for all unobserved or confounding factors. Miguel and Kremer (2003) note that several methodological issues hamper many existing randomized treatment-control evaluations. First, externalities associated with interventions can lead to underestimating impacts among the untreated population. Second, sample selection and attrition issues can affect the validity of findings, although the direction of this effect is ambiguous. Third, existing studies typically evaluate the impact of deworming on cognitive skills, likely the culmination of several years of health and education investments, as assessed by tests administered to treated and untreated children. Although studies find an effect on cognitive skills for those with the heaviest worm burden, they do not focus on other important education outcomes, which are likely to be more affected in the short run by health improvements, such as school enrollment and school attendance.
The study by Miguel and Kremer (2003) in Kenya attempts to address those shortcomings through improved study design and analytical methods. In addition to providing health gains, deworming reduced total primary school absenteeism by at least one-quarter in the first two years of the project. The gains were largest for the youngest children, who suffered from more intense worm infections. Externalities would cause a substantial underestimation of this effect. In terms of cost-effectiveness as an educational intervention, deworming proved to be far more effective at improving school attendance than other educational interventions implemented in a study in Kenya. Deworming offers a high rate of return, increasing the net present value of discounted wages by more than US$30 per treated child compared with per treatment costs of under US$1. For realistic estimates of returns to schooling, these results show in general that the net present discounted value of lifetime earnings is high compared with the costs of treatment even for small gains in school participation (figure 24.3).
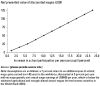
Figure 24.3
Returns to School Participation
Bleakley (2003) examined the effect of a hookworm control program undertaken about 1910 in the southern part of the United States. Hookworm infection was estimated to cause a 23 percent drop in the probability of school attendance, and children with greater exposure to the hookworm eradication campaign were more likely to be literate. Moreover, the long-term follow-up of affected cohorts showed that hookworm infection in childhood led to significantly lower wages in adulthood.
Helminth infections in preschool-age children can have consequences for subsequent schooling, such as delaying primary school enrollment and school attainment, thereby affecting future labor market outcomes. Bobonis, Miguel, and Sharma (2003) conducted a study of preschool-age children, using iron supplementation and deworming drugs administered to children two to six years of age. Preliminary results indicate that, in addition to the weight gain associated with treatment, average preschool participation rates increased sharply by 6.3 percentage points among assisted children older than two, reducing preschool absenteeism by roughly one-fifth.
Beyond the current impacts on schooling and implications for cognition, helminth infection in children can have long-term implications for economic outcomes in adulthood through its effect on physical growth. Height has been shown to affect wage-earning capacity as well as participation in the labor force for men and women (Thomas and Strauss 1997). This relationship may be strongest in settings where infection rates are highest—that is, low-income areas, where physical endurance yields high returns in the labor market.
Implementation of Control Strategies: Lessons of Experience
Two case studies illustrate the profound health effects of periodic deworming.
Case Study Number 1: Periodic Deworming in Ghana and Tanzania
The Partnership for Child Development (PCD) undertook an evaluation of the use of schools in Ghana and Tanzania for the delivery of health interventions, including research into the processes, costs, and benefits (PCD 1999). The effort also involved operations research and evaluation of programs with regard to health and education outcomes and people's perceptions of the programs (Hall and others 1999). The results demonstrated the following:
- Simple interventions, such as deworming, have the potential to improve children's health and educational achievement, especially for those worst affected and most disadvantaged.
- The delivery of school-based health services is efficient and cost-effective and is supported by the key stakeholders involved. Implementers of the school health programs in the education and health sectors and the community are positive regarding the teacher's role in health provision, as long as the health interventions are simple, safe, familiar, and effective and are seen as responding to local needs.
- The provision of health services through schools need not require long and complex training, nor significantly add to the workload of teachers or administrators.
- Delivery through the existing education sector could occur effectively without any additional infrastructure, as long as the existing educational system is adequately functional.
The results of the evaluation of these programs also highlighted the need for deworming to be carried out in the context of a wider framework of school health, which includes core activities such as effective and nondiscriminatory school health policies, provision of safe water and sanitation, and effective health education (http://www.freshschools.org and http://www.schoolsandhealth.org).
Case Study Number 2: Schistosomiasis Control in Egypt
In 1937, the prevalence of schistosomiasis in rural areas was about 50 percent, almost every boy had blood in his urine by the age of 12, and bladder cancer was the commonest cancer in Egypt. Molluscicides, from copper sulfate to niclosamide, have been used to try to kill the host snails, and drugs from antimony-based compounds, through niridazole, metrifonate, and PZQ, have been used to treat the millions of infected Egyptians. Finally, after a 14-year control campaign using PZQ, the prevalence of schistosomiasis has been reduced to below 10 percent. With infection intensities now low, the serious health consequences of schistosomiasis have disappeared. The program was started in 1988, when using loans from the African Development Bank and the World Bank, Egypt invested heavily in the purchase of PZQ, encouraging local production, to control morbidity caused by schistosomiasis. Since the drug was first made available in 1988, some 45 million doses have been dispensed. A television campaign has encouraged people to submit samples for diagnosis and to receive free treatment if their diagnoses are positive. Since 1997, a mass chemotherapy campaign was used to target populations in high-prevalence villages and children in selected governorates where prevalence was greater than 20 percent. In addition, molluscicides have been applied in canals around high-prevalence villages. The widespread use of PZQ has given dramatic results. Morbidity, including hematuria, has almost disappeared, and bladder cancer is on the decline.
Research and Development
Among the important tasks to be done to control helminth infections are collection of better data on helminth disease burden, research on the health and economic effects (and safety) of periodic deworming, monitoring of the emergence of anthelmintic drug resistance, and development of new tools to supplement or complement existing control strategies.
Health and Economic Impact
Overall, better estimates of disease burden are needed (Michaud, Gordon, and Reich 2003), especially with respect to obtaining a consistent and agreed-on estimate for the DALYs attributed to helminth infections. In a systematic review of randomized deworming trials, Dickson and others (2000) conclude that, although data support the effects of deworming on weight gain, there are inconsistencies among trials and insufficient evidence as to whether such interventions improve cognitive performance. Plausible mechanisms by which helminths suppress growth in childhood and exert negative impacts on intelligence, cognition, and school performance are largely unstudied and unknown. In addition, some reports have questioned whether albendazole itself could adversely affect growth (Forrester and others 1998). Those issues require clarification as widespread deworming programs become more common. The impact of helminths on populations other than schoolchildren, including preschool-age children, women of childbearing age, and adult workers, appears to be substantial. However, those populations are understudied. Also unclear is the impact of childhood STH and schistosome infections on productivity in adulthood. The effect of chemotherapy on many of the manifestations of schistosomiasis has not been assessed systematically. It has been postulated that PZQ treatment of schistosomiasis promotes partial immunity by destroying worms in the vasculature and releasing parasite antigens (Colley and Secor 2004). In contrast, the frequent and periodic treatment of STH infections (Albonico and others 1995) do not appear to promote natural protective immunity.
The role of helminths and coinfections also warrants further exploration. Some studies suggest that HIV-1 infection may promote susceptibility to schistosomiasis (Secor, Karanja, and Colley 2004), and human T-cell lymphotropic virus-1 (HTLV-1) infection may promote susceptibility to strongyloidiasis. In addition, emerging evidence indicates that STH and schistosome infections may promote susceptibility to other infectious agents, possibly including HIV/AIDS and malaria (Fincham, Markus, and Adams 2003). This phenomenon, if verified in an epidemiologic study, would further increase helminth-associated DALY estimates.
Anthelmintic Drug Resistance and New Drug Development
A concern about the feasibility of sustainable control with BZAs is the possible emergence of drug resistance among human STHs. BZA resistance occurs because of the spread of point mutations in nematode-tubulin alleles. This phenomenon has already resulted in widespread BZA drug resistance among STHs of ruminant livestock. There is still no direct evidence for BZA resistance among human STHs, although such resistance could account for an observed failure of mebendazole for human hookworm in southern Mali, as well as a diminished efficacy against hookworm in Zanzibar following frequent and periodic use of mebendazole (Albonico and others 2003). PZQ resistance must also be considered, especially as it begins to be widely used in Sub-Saharan Africa (Hagan and others 2004). Should PZQ resistance develop, there will be new demands for antischistosomal drugs. Recently, the artemisins have shown activity against schistosomulae and were successful in protecting against S. japonicum in China (Hagan and others 2004).
Anthelmintic Vaccines
The high rates of reinfection that can occur following treatment with anthelmintic drugs and concern about emerging drug resistance have prompted the search for alternative control tools. For most helminth infections, reduction in adult worm burden has been considered the "gold standard" for vaccine development. For schistosomiasis, however, a vaccine that targets parasite fecundity and egg viability, thereby reducing pathology and transmission, would also represent an important breakthrough. A 28-kDa glutathione S-transferase (GST) has shown promise as a protective antigen for S. haematobium infection (Capron and others 2005). The S. haematobium vaccine project based on GST has successfully passed phase 1 testing; the research group, which is based at the Pasteur Institute, is embarking on phase 2 clinical trials in Senegal and Niger. Additional schistosomiasis vaccines are also undergoing early-stage development. Efforts are also under way by the Human Hookworm Vaccine Initiative to develop and test a first-generation recombinant hookworm vaccine (Brooker and others 2005; Hotez, Bethony and others 2005). The first vaccine manufactured under current good manufacturing practices and tested for quality control and toxicity is the Na-ASP-2 hookworm vaccine, which was developed from research demonstrating human correlates of immunity and partial protection data in vaccinated laboratory animals. Phase 1 human trials for evaluating the safety and immunogenicity of the Na-ASP-2 hookworm vaccine are in progress. Additional research is needed to determine how an anthelmintic vaccine can be incorporated into existing control programs, as well how it would be used for at-risk populations not currently targeted for periodic deworming in schools.
Conclusions: Promises and Pitfalls
Fulfilling the mandate of World Health Assembly resolution 54.19 will require the regular treatment of hundreds of millions of children over decades. The obstacles in this undertaking are formidable, and success will depend on the ability of countries to identify or create reliable and sustained infrastructures for this purpose. A focus on using preexisting school systems may be key to achieving this goal. The treatment of schoolchildren for A. lumbricoides, T. trichiura, and schistosome infections achieves large externalities that reduce infection in other vulnerable age groups. However, the different epidemiology of hookworm raises concerns about the risks to preschool children and women of reproductive age who remain untreated. Providing regular treatment to these populations appears to be a less cost-effective option, largely because of the absence of a preexisting infrastructure. This situation presents a strong argument for developing a hookworm vaccine that could be used to protect these vulnerable groups. It has yet to be seen whether the emergence of BZA drug resistance is a genuine concern that could derail global deworming efforts in much the same way that resistance to DDT and chloroquine has affected the ambitions for global malaria control. It is possible that the specific dynamics of helminth populations will provide sufficient genetic flow to maintain susceptibility in much the same way that insecticides remain the effective staple of global agribusiness. Until new technologies become available, anthelmintic chemotherapy for school-age children remains the most practical and substantive means to control STH and schistosome infections in the developing world.
References
- Adams V. J., Lombard C. J., Dhansay M. A., Markus M. B., Fincham J. E. Efficacy of Albendazole against the Whipworm Trichuris Trichiura—A Randomized, Controlled Trial. South African Medical Journal. 2004;94:972–76. [PubMed: 15662995]
- Albonico M., Bickle Q., Ramsan M., Montresor A., Savioli L., Taylor M. Efficacy of Mebendazole and Levamisole Alone or in Combination against Intestinal Nematode Infections after Repeated Targeted Mebendazole Treatment in Zanzibar. Bulletin of the World Health Organization. 2003;81:343–52. [PMC free article: PMC2572452] [PubMed: 12856052]
- Albonico, M., A. Montresor, D. W. Crompton, and L. Savioli. Forthcoming."Intervention for the Control of Soil—Transmitted Helminthiasis."Advances in Parasitology. [PMC free article: PMC5633078] [PubMed: 16735168]
- Albonico M., Renganathan E., Bosman A., Kisumku U. M., Alawi K. S., Savioli L. Efficacy of a Single Dose of Mebendazole on Prevalence and Intensity of Soil-Transmitted Nematodes in Zanzibar. Tropical and Geographic Medicine. 1994;46:142–46. [PubMed: 7941002]
- Albonico M., Smith P. G., Ercole E., Hall A., Chwaya H. M., Alawi K. S., Savioli L. Rate of Reinfection with Intestinal Nematodes after Treatment of Children with Mebendazole or Albendazole in a Highly Endemic Area. Transactions of the Royal Society of Tropical Medicine and Hygiene. 1995;89:538–41. [PubMed: 8560535]
- Anderson, R. M., and R. M. May. 1991. Infectious Diseases of Humans.Oxford, U.K.: Oxford University Press.
- Asaolu S. O., Ofoezie I. E. The Role of Health Education and Sanitation in the Control of Helminth Infections. Acta Tropica. 2003;86:283–94. [PubMed: 12745145]
- Bethony J., Williams J. T., Kloos H., Blangero J., Alves-Fraga L., Buck G. et al. Exposure to Schistosoma mansoni Infection in a Rural Area in Brazil: II. Household Risk Factors. Tropical Medicine and International Health. 2001;6:136–45. [PubMed: 11251910]
- Bleakley H. Disease and Development: Evidence from Hookworm Eradication in the American South. Journal of the European Economic Association. 2003;1:376–86. [PMC free article: PMC3800113] [PubMed: 24146438]
- Bobonis, G., E. Miguel, C. Sharma. 2003. "Iron Deficiency Anemia and School Participation." University of California–Berkeley. http://emlab
.berkeley .edu/users/emiguel/miguel_anemia.pdf. - Brooker S., Bethony J., Hotez P. J. Human Hookworm Infection in the 21st Century. Advances in Parasitology. 2004;58:197–288. [PMC free article: PMC2268732] [PubMed: 15603764]
- Brooker S., Bethony J. M., Rodrigues L., Alexander N., Geiger S., Hotez P. J. Epidemiological, Immunological and Practical Considerations in Developing and Evaluating a Human Hookworm Vaccine. Expert Review of Vaccines. 2005;4:35–50.
- Brooker S., Michael E. The Potential of Geographical Information Systems and Remote Sensing in the Epidemiology and Control of Human Helminth Infections. Advances in Parasitology. 2000;47:245–87. [PubMed: 10997209]
- Brooker S., Whawell S., Kabatereine N. B., Fenwick A., Anderson R. M. Evaluating the Epidemiological Impact of National Control Programmes for Helminths. Trends in Parasitology. 2004;11:537–45. [PubMed: 15471706]
- Bundy, D. A. 1995. "Epidemiology and Transmission of Intestinal Helminths." In Enteric Infection 2, Intestinal Helminths, ed. M. J. G.Farthing, G. T. Keusch, and D. Wakelin, 5–24. London: Chapman & Hall Medical.
- Bundy D. A., Chan M. S., Savioli L. Hookworm Infection in Pregnancy. Transactions of the Royal Society of Tropical Medicine and Hygiene. 1995;89:521–22. [PubMed: 8560530]
- Bundy D. A., Wong M. S., Lewis L. L., Horton J. Control of Geohelminths by Delivery of Targeted Chemotherapy through Schools. Transactions of the Royal Society of Tropical Medicine and Hygiene. 1990;84:115–20. [PubMed: 2345909]
- Capron A., Riveau G., Capron M., Trottein F. Schistosomes: The Road from Host-Parasite Interactions to Vaccines in Clinical Trials. Trends in Parasitology. 2005;21:143–49. [PubMed: 15734662]
- Chan M. S., Medley G. F., Jamison D., Bundy D. A. The Evaluation of Potential Global Morbidity Attributable to Intestinal Nematode Infections. Parasitology. 1994;109:373–87. [PubMed: 7970892]
- Christian P., Khatry S. K., West K. P. Antenatal Anthelmintic Treatment, Birthweight, and Infant Survival in Rural Nepal. Lancet. 2004;364:981–83. [PubMed: 15364190]
- Colley D. G., Secor E. W. Immunoregulation and World Health Assembly Resolution 54.19: Why Does Treatment Control Morbidity? Parasitology International. 2004;53:143–50. [PubMed: 15081946]
- Crompton D. W. How Much Helminthiasis Is There in the World? Journal of Parasitology. 1999;85:397–403. [PubMed: 10386428]
- Crompton D. W., Savioli L. Intestinal Parasitic Infections and Urbanization. Bulletin of the World Health Organization. 1993;71:1–7. [PMC free article: PMC2393435] [PubMed: 8440028]
- de Silva N. R., Brooker S., Hotez P. J., Montresor A., Engles D., Savioli L. Soil-Transmitted Helminth Infections: Updating the Global Picture. Trends in Parasitology. 2003;19:547–51. [PubMed: 14642761]
- de Silva N. R., Chan M. S., Bundy D. A. P. Morbidity and Mortality Due to Ascariasis: Re-estimation and Sensitivity Analysis of Global Numbers at Risk. Tropical Medicine and International Health. 1997;2:519–28. [PubMed: 9236818]
- Dickson R., Awasthi S., Williamson P., Demellweek C., Garner P. Effects of Treatment for Intestinal Helminth Infection on Growth and Cognitive Performance in Children: Systematic Review of Randomised Trials. British Medical Journal. 2000;320:1697–701. [PMC free article: PMC27412] [PubMed: 10864543]
- Drake L. J., Jukes M. C. H., Sternberg R. J., Bundy D. A. P. Geohelminth Infections (Ascariasis, Trichuriasis, and Hookworm): Cognitive and Developmental Impacts. Seminars in Pediatric Infectious Diseases. 2000;11:245–51.
- Fenwick A., Savioli L., Engels D., Bergquist N. R., Todd M. H. Drugs for the Control of Parasitic Diseases: Current Status and Development in Schistosomiasis. Trends in Parasitology. 2003;19:509–15. [PubMed: 14580962]
- Fincham J. E., Markus M. B., Adams V. J. Could Control of Soil-Transmitted Helminthic Infection Influence the HIV/AIDS Pandemic? Acta Tropica. 2003;86:315–33. [PubMed: 12745148]
- Forrester J. E., Bailar J. C. III, Esrey S. A., Jose M. V., Castillejos B. T., Ocamp G. Randomised Trial of Albendazole and Pyrantel in Symptomless Trichuriasis in Children. Lancet. 1998;353:1103–8. [PubMed: 9798586]
- Guyatt H. L. Do Intestinal Nematodes Affect Productivity in Adulthood? Parasitology Today. 2000;16:153–58. [PubMed: 10725902]
- ———.2003The Cost of Delivering and Sustaining a Control Programme for Schistosomiasis and Soil-Transmitted Helminthiasis Acta Tropica 86267–74. [PubMed: 12745143]
- Haas J. D., Brownlie T. Iron Deficiency and Reduced Work Capacity: A Critical Review of the Research to Determine a Causal Relationship. Journal of Nutrition. 2001;131(Suppl.):676S–88S. [PubMed: 11160598]
- Hagan P., Appleton C. C., Coles G. C., Kusel J. R., Tchuem-Tchuente L. A. Schistosomiasis Control: Keep Taking the Tablets. Trends in Parasitology. 2004;20:92–97. [PubMed: 14747023]
- Hall A., Nokes C., Wen S. T., Adjei S., Kihamia C., Mwanri L. et al. Alternatives to Bodyweight for Estimating the Dose of Praziquantel Needed to Treat Schistosomiasis. Transactions of the Royal Society of Tropical Medicine and Hygiene. 1999;93:652–58. [PubMed: 10717759]
- Hotez, P. J., S. Arora, J. Bethony, M. E. Bottazzi, A. Loukas, R. Correa-Oliveira, and S. Brooker. 2005. "Helminth Infections of Children: Prospects for Control." In Hot Topics in Infection and Immunity in Children, ed. A. J. Pollard and A. Finn. New York: Springer. [PubMed: 16108129]
- Hotez P. J., Bethony J., Bottazzi M. E., Brooker S., Buss P. Hookworm—`The Great Infection of Mankind.'. Public Library of Science Medicine. 2005;2:e67. [PMC free article: PMC1069663] [PubMed: 15783256]
- Hotez P. J., Brooker S., Bethony J. M., Bottazzi M. E., Loukas A., Xiao S. H. Current Concepts: Hookworm Infection. New England Journal of Medicine. 2004;351:799–807. [PubMed: 15317893]
- Kabatereine N. B., Vennervald B. J., Ouma J. H., Kemijumbi J., Butterworth A. E., Dunne D. W., Fulford A. J. Adult Resistance to Schistosomiasis Mansoni: Age-Dependence to Reinfection Remains Constant in Communities with Diverse Exposure Patterns. Parasitology. 1999;118:101–5. [PubMed: 10070667]
- King C. H., Dickman K., Tisch D. J. Reassessment of the Cost of Chronic Helmintic Infection: A Meta-analysis of Disability-Related Outcomes in Endemic Schistosomiasis. Lancet. 2005;365:1561–69. [PubMed: 15866310]
- Lansdown R., Ledward A., Hall A., Issac W., Yona E., Matulu J. et al. Schistosomiasis, Helminth Infection, and Health Education in Tanzania: Achieving Behaviour Change in Primary Schools. Health Education Research. 2002;17:425–33. [PubMed: 12197588]
- Michaud, C. M., W. S. Gordon, and M. R. Reich. 2003. "The Global Burden of Disease Due to Schistosomiasis." Disease Control Priorities Project Working Paper 19. http://www
.fic.nih.gov/dcpp/wps/wp19.pdf. - Miguel E. A., Kremer M. Worms: Identifying Impacts on Education and Health in the Presence of Treatment Externalities. Econometrica. 2003;72(1):159–217.
- Montresor, A., D. W. T. Crompton, T. W. Gyorkos, and L. Savioli. 2002.Helminth Control in School-Age Children: A Guide for Managers of Control Programmes. Geneva: World Health Organization.
- PCD (Partnership for Child Development). The Cost of Large-Scale School Health Programmes Which Deliver Anthelmintics to Children in Ghana and Tanzania. Acta Tropica. 1999;73(2):183–204. [PubMed: 10465058]
- Psacharopoulos, G., and H. Patrinos. 2002. "Returns to Investment in Education: A Further Update." Working Paper 2881, World Bank, Washington, DC.
- Quinnell R. J. Genetics of Susceptibility to Human Helminth Infection. International Journal of Parasitology. 2003;33:1219–31. [PubMed: 13678637]
- Richter J. The Impact of Chemotherapy on Morbidity Due to Schistosomiasis. Acta Tropica. 2003;86:161–83. [PubMed: 12745135]
- Ross A. G., Bartley P. B., Sleigh A. C., Olds G. R., Li Y., Williams G. M., McManus D. P. Schistosomiasis. New England Journal of Medicine. 2002;346:1212–20. [PubMed: 11961151]
- Secor W. E., Karanja D. M., Colley D. G. Interactions between Schistosomiasis and Human Immunodeficiency Virus in Western Kenya. Memorias do Instituto Oswaldo Cruz. 2004;99(5 Suppl. 1):93–95. [PubMed: 15486642]
- Stephenson L. S., Latham M. C., Ottesen E. A. Malnutrition and Parasitic Helminth Infections. Parasitology. 2000;121(Suppl.):S23–28. [PubMed: 11386688]
- Stoltzfus R. J., Chwaya H. M., Montresor A., Tielsch J. M., Jape J. K., Albonico M., Savioli L. Low Dose Daily Iron Supplementation Improves Iron Status and Appetite but Not Anemia, Whereas Quarterly Anthelmintic Treatment Improves Growth, Appetite, and Anemia in Zanzibari Preschool Children. Journal of Nutrition. 2004;134:348–56. [PubMed: 14747671]
- Stoltzfus R. J., Dreyfuss M. L., Chwaya H. M., Albonico M. Hookworm Control as a Strategy to Prevent Iron Deficiency Anemia. Nutrition Reviews. 1997;55:223–32. [PubMed: 9279058]
- Thomas D., Strauss J. Health and Wages: Evidence on Men and Women in Urban Brazil. Journal of Econometrics. 1997;77:159–85. [PubMed: 12292719]
- Van der Werf M. J., de Vlas S. J., Brooker S., Looman C. W., Nagelkerke N. J., Habbema J. D., Engels D. Quantification of Clinical Morbidity Associated with Schistosome Infection in Sub-Saharan Africa. Acta Tropica. 2003;86:125–39. [PubMed: 12745133]
- WHO (World Health Organization). 2002. Prevention and Control of Schistosomiasis and Soil-Transmitted Helminthiasis. WHO Technical Series Report 912. Geneva: WHO. [PubMed: 12592987]
- World Bank. 2003. School Deworming at a Glance. Public Health at a Glance Series. http://www
.worldbank.org/hnp.
- Causes and Characteristics of Helminth Infections
- Epidemiology of STH Infections and Schistosomiasis
- Burden of the Disease
- Cost-Effectiveness Analysis of Interventions
- Averted, Avertable, and Nonavertable Burden
- Economic Benefits of Intervention
- Implementation of Control Strategies: Lessons of Experience
- Research and Development
- Conclusions: Promises and Pitfalls
- References
- Prevalence and intensity of human soil transmitted helminth infections in the Akonolinga health district (Centre Region, Cameroon): Are adult hosts contributing in the persistence of the transmission?[Parasite Epidemiol Control. 2016]Prevalence and intensity of human soil transmitted helminth infections in the Akonolinga health district (Centre Region, Cameroon): Are adult hosts contributing in the persistence of the transmission?Bopda J, Nana-Djeunga H, Tenaguem J, Kamtchum-Tatuene J, Gounoue-Kamkumo R, Assob-Nguedia C, Kamgno J. Parasite Epidemiol Control. 2016 Jun; 1(2):199-204. Epub 2016 Mar 22.
- Prevalence of Schistosomes and Soil-Transmitted Helminths and Morbidity Associated with Schistosomiasis among Adult Population in Lake Victoria Basin, Tanzania.[Korean J Parasitol. 2015]Prevalence of Schistosomes and Soil-Transmitted Helminths and Morbidity Associated with Schistosomiasis among Adult Population in Lake Victoria Basin, Tanzania.Siza JE, Kaatano GM, Chai JY, Eom KS, Rim HJ, Yong TS, Min DY, Chang SY, Ko Y, Changalucha JM. Korean J Parasitol. 2015 Oct; 53(5):525-33. Epub 2015 Oct 29.
- Prevalence of Schistosomes and Soil-Transmitted Helminths among Schoolchildren in Lake Victoria Basin, Tanzania.[Korean J Parasitol. 2015]Prevalence of Schistosomes and Soil-Transmitted Helminths among Schoolchildren in Lake Victoria Basin, Tanzania.Siza JE, Kaatano GM, Chai JY, Eom KS, Rim HJ, Yong TS, Min DY, Chang SY, Ko Y, Changalucha JM. Korean J Parasitol. 2015 Oct; 53(5):515-24. Epub 2015 Oct 29.
- Review Schistosomiasis and soil-transmitted helminthiasis in Rwanda: an update on their epidemiology and control.[Infect Dis Poverty. 2017]Review Schistosomiasis and soil-transmitted helminthiasis in Rwanda: an update on their epidemiology and control.Rujeni N, Morona D, Ruberanziza E, Mazigo HD. Infect Dis Poverty. 2017 Mar 1; 6(1):8. Epub 2017 Mar 1.
- Review Immuno-epidemiology of Schistosoma mansoni infections in endemic populations co-infected with soil-transmitted helminths: present knowledge, challenges, and the need for further studies.[Acta Trop. 2008]Review Immuno-epidemiology of Schistosoma mansoni infections in endemic populations co-infected with soil-transmitted helminths: present knowledge, challenges, and the need for further studies.Geiger SM. Acta Trop. 2008 Nov-Dec; 108(2-3):118-23. Epub 2008 May 24.
- Helminth Infections: Soil-transmitted Helminth Infections and Schistosomiasis - ...Helminth Infections: Soil-transmitted Helminth Infections and Schistosomiasis - Disease Control Priorities in Developing Countries
- icaB [Staphylococcus aureus subsp. aureus Mu3]icaB [Staphylococcus aureus subsp. aureus Mu3]Gene ID:5561455Gene
- SpyM3_0383 [Streptococcus pyogenes MGAS315]SpyM3_0383 [Streptococcus pyogenes MGAS315]Gene ID:1008697Gene
- A530013C23Rik RIKEN cDNA A530013C23 gene [Mus musculus]A530013C23Rik RIKEN cDNA A530013C23 gene [Mus musculus]Gene ID:329562Gene
- Fndc7 fibronectin type III domain containing 7 [Mus musculus]Fndc7 fibronectin type III domain containing 7 [Mus musculus]Gene ID:320181Gene
Your browsing activity is empty.
Activity recording is turned off.
See more...