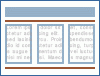
Table
In vitro Rodents
Background
[PubMed]
The vascular endothelial growth factor A isoform 121 (VEGF121)-gelonin fusion protein (VEGF121/rGel)–conjugated manganese ferrite (MnFe2O4) nanoparticle (NP), abbreviated as VEGF121/rGel-MNP, is a VEGF receptor (VEGFR)-targeted contrast agent developed by Cho et al. for monitoring the targeting efficiency and treatment efficacy of the VEGF121/rGel immunotoxin (1).
VEGFs are a group of five potent inducers of cell migration, invasion, vascular permeability, and neovascular formation (2). They act via three receptor tyrosine kinases: VEGFR-1, VEGFR-2, and VEGFR-3 (3). These receptors are overexpressed on the endothelial cells of tumor neovasculature and are almost undetectable in the endothelium of adjacent normal tissues. The critical role of the VEGF/VEGFR signal pathway in tumor angiogenesis has prompted great efforts in the development of antiangiogenic therapies, and agents have been tested by acting on different steps of the pathway, such as by binding to the VEGF ligand, inhibiting VEGFR tyrosine kinase, inhibiting downstream effectors (mammalian target of rapamycin inhibitors), and modulating VEGF production (4-6). These agents are highly effective against tumor growth in animal models when they are used alone; however, they seldom lead to tumor regression and exhibit insufficient efficacy in the clinical setting, although combination with chemotherapy has been shown to improve patient survival in certain tumor types. The most likely explanation for this phenominon is that tumor endothelial cells can adapt to antiangiogenic treatment and form functional vasculature that loses sensitivity to the inhibitors of VEGF/VEGFR (4, 6). It is hypothesized that VEGF/VEGFR-targeted therapy should be administrated before the development of a well-established vascular network.
Molecular imaging provides a means to reveal the mechanism underlying this phenomenon and to monitor the antiangionic therapy (1, 7). VEGF121/rGel has been generated with VEGF121, which is linked with recombinant plant toxin gelonin through a G4S tether (4, 8-10). Gelonin is a member of the ribosome-inactivating protein family, which depurinates rRNA and other polynucleotide substrates and subsequently inhibits protein synthesis (7, 11). A series of preclinical studies showed that VEGF121/rGel could specifically inhibit the growth of tumor endothelial cells (8-10, 12). Like other immunotoxins, VEGF121/rGel is also expected to be effective against tumors resistant to VEGF/VEGFR-targeting inhibitors if the tumor cells express sufficient levels of VEGFR. To monitor the VEGFR-targeting efficiency of VEGF121/rGel with imaging techniques, Hsu et al. and Cho et al. labeled the VEGF121/rGel with 64Cu (64Cu-DOTA-VEGF121/rGel) and with MnFe2O4 NPs (VEGF121/rGel-MNPs), respectively (1, 7). Both imaging studies have concluded that noninvasive imaging with VEGF121/rGel will be useful to monitor the treatment efficacy and to identify patients who may benefit from the VEGF121/rGel therapy. This chapter summarizes data obtained with VEGF121/rGel-MNPs.
Synthesis
[PubMed]
Cho et al. described the synthesis of VEGF121/rGel-MNPs (1). MnFe2O4 NPs (diameter, ~12 nm) were synthesized with the seed-mediated growth method and capped with carboxylated polysorbate 80 to generate carboxylated water-soluble NPs. Characterization showed that the NPs exhibited superparamagnetic behavior without magnetic hysteresis, had a saturation magnetization value of 1.56 emu/g at 0.85 T, and had a relaxivity coefficient of 423 mM−1s−1 at 1.5 T. The size of the NPs was determined with light scattering to be 38.9 ± 0.6 nm. The colloidal stability was maintained for >3 months.
The synthesis, expression, and purification of the VEGF121/rGel immunotoxin were performed as described previously by Veenendaal et al. (8). The molecular weight of VEGF121/rGel was 84 kDa. VEGF121/rGel was conjugated to the carboxyl group of the carboxylated NPs. Conjugation of the VEGF121/rGel to the NPs increased the NP size slightly to 44.5 ± 1.2 nm and changed the surface charge from −19.6 ± 3.1 mV to −1.4 ± 3.9 mV. There were ~17 equivalent VEGF121/rGel molecules per carboxylated NP.
In Vitro Studies: Testing in Cells and Tissues
[PubMed]
Cho et al. first evaluated the expression levels of a set of receptors in a group of cell lines (1). PAE/KDR cells (porcine aortic endothelial cells transfected with cDNA of VEGFR2) exhibited >105 times and >108 times more VEGFR2 expression than did HUVEC cells and 253JB-V cells (a human bladder cancer cell line), respectively. The binding of VEGF121/rGel-MNPs with VEGFR2 was tested using PAE/KDR cells that overexpress VEGFR2, with 253JB-V cells as the control. T2-Weighted magnetic resonance imaging (MRI) at 1.5 T showed that VEGF121/rGel-MNPs exhibited a dose-dependent enhancement of the signal intensity over the concentration range of 0.04–5.0 µg for the PAE/KDR cells but not for the 253JB-V cells. VEGF121/rGel-MNPs were predominantly localized in the cytoplasm.
The binding specificity of VEGF121/rGel-MNPs to PAE/KDR cells was further tested with VEGF121 as a competeting inhibitor (1). PAE/KDR cells were pretreated for 1 h at 4°C with VEGF121 (molar ratios of 0.1, 1.0, and 10.0 compared with equivalent VEGF121/rGel). Cells were then incubated with 3.0 µg VEGF121/rGel-MNPs for 1 h. As the molar ratio of VEGF121:VEGF121/rGel increased, the signal enhancement decreased. At a molar ratio of 10.0, the normalized ΔR2/R2noninhibition value was ~90%.
The biological activity of the VEGF121/rGel-MNPs on VEGFR2 expression after incubation with PAE/KDR cells for 72 h at 37°C was analyzed with immunoblotting (1). The expression of phosphorylated VEGFR2 was slightly reduced with VEGF121/rGel-MNPs compared with VEGF121/rGel, but increased expression was observed at concentrations ≥10 nM. The 50% inhibition concentrations of free VEGF121/rGel, rGel, and VEGF121/rGel-MNPs on PAE/KDR cells after 72 h incubation were determined to be 0.4, 76.1, and 50.3 nM, respectively..
Animal Studies
Rodents
[PubMed]
In vivo MRI was performed in male BALB/c nude mice bearing 253JB-V tumor xenografts in the bladder dome (n = 5 mice) (1). VEGF121/rGel-MNPs (200 µg Fe + Mn/200 µl) were injected via the tail vein, and MRI was performed at 3.0 T. After injection, MRI signal enhancement was identified initially for the vessels surrounding the bladder and later for the intratumoral vessels (for up to 4 h). Pretreatment with VEGF121 (200 µg Fe) for 4 h blocked the contrast enhancement of tumor vessels induced by VEGF121/rGel-MNPs, showing significant enhancement of the tumor vessels immediatelty after VEGF121/rGel-MNPs injection which was then disappeared within 4 h. These results were confirmed with ex vivo images. The targeted delivery of VEGF121/rGel-MNPs to intratumoral vessels was further verified with immunofluorescence staining of the gelonin and endothelial cells.
References
- 1.
- Cho E.J., Yang J., Mohamedali K.A., Lim E.K., Kim E.J., Farhangfar C.J., Suh J.S., Haam S., Rosenblum M.G., Huh Y.M. Sensitive Angiogenesis Imaging of Orthotopic Bladder Tumors in Mice Using a Selective Magnetic Resonance Imaging Contrast Agent Containing VEGF121/rGel. Invest Radiol. 2011;46(7):441–449. [PubMed: 21512397]
- 2.
- Koch S., Tugues S., Li X., Gualandi L., Claesson-Welsh L. Signal transduction by vascular endothelial growth factor receptors. Biochem J. 2011;437(2):169–83. [PubMed: 21711246]
- 3.
- Press M.F., Lenz H.J. EGFR, HER2 and VEGF pathways: validated targets for cancer treatment. Drugs. 2007;67(14):2045–75. [PubMed: 17883287]
- 4.
- Backer M.V., Hamby C.V., Backer J.M. Inhibition of vascular endothelial growth factor receptor signaling in angiogenic tumor vasculature. Adv Genet. 2009;67:1–27. [PubMed: 19914448]
- 5.
- Munagala R., Aqil F., Gupta R.C. Promising molecular targeted therapies in breast cancer. Indian J Pharmacol. 2011;43(3):236–45. [PMC free article: PMC3113372] [PubMed: 21713084]
- 6.
- Thorpe P.E. Vascular targeting agents as cancer therapeutics. Clin Cancer Res. 2004;10(2):415–27. [PubMed: 14760060]
- 7.
- Hsu A.R., Cai W., Veeravagu A., Mohamedali K.A., Chen K., Kim S., Vogel H., Hou L.C., Tse V., Rosenblum M.G., Chen X. Multimodality molecular imaging of glioblastoma growth inhibition with vasculature-targeting fusion toxin VEGF121/rGel. J Nucl Med. 2007;48(3):445–54. [PubMed: 17332623]
- 8.
- Veenendaal L.M., Jin H., Ran S., Cheung L., Navone N., Marks J.W., Waltenberger J., Thorpe P., Rosenblum M.G. In vitro and in vivo studies of a VEGF121/rGelonin chimeric fusion toxin targeting the neovasculature of solid tumors. Proc Natl Acad Sci U S A. 2002;99(12):7866–71. [PMC free article: PMC122986] [PubMed: 12060733]
- 9.
- Mohamedali K.A., Kedar D., Sweeney P., Kamat A., Davis D.W., Eve B.Y., Huang S., Thorpe P.E., Dinney C.P., Rosenblum M.G. The vascular-targeting fusion toxin VEGF121/rGel inhibits the growth of orthotopic human bladder carcinoma tumors. Neoplasia. 2005;7(10):912–20. [PMC free article: PMC1550288] [PubMed: 16242074]
- 10.
- Akiyama H., Mohamedali K.A. E.S. RL, S. Kachi, J. Shen, C. Hatara, N. Umeda, S.F. Hackett, S. Aslam, M. Krause, H. Lai, M.G. Rosenblum, and P.A. Campochiaro, Vascular targeting of ocular neovascularization with a vascular endothelial growth factor121/gelonin chimeric protein. Mol Pharmacol. 2005;68(6):1543–50. [PubMed: 16150930]
- 11.
- Wahl O., Oswald M., Tretzel L., Herres E., Arend J., Efferth T. Inhibition of tumor angiogenesis by antibodies, synthetic small molecules and natural products. Curr Med Chem. 2011;18(21):3136–55. [PubMed: 21671856]
- 12.
- Ran S., Mohamedali K.A., Luster T.A., Thorpe P.E., Rosenblum M.G. The vascular-ablative agent VEGF(121)/rGel inhibits pulmonary metastases of MDA-MB-231 breast tumors. Neoplasia. 2005;7(5):486–96. [PMC free article: PMC1501168] [PubMed: 15967101]
Publication Details
Author Information and Affiliations
Publication History
Created: July 6, 2011; Last Update: August 17, 2011.
Copyright
Publisher
National Center for Biotechnology Information (US), Bethesda (MD)
NLM Citation
Shan L. Vascular endothelial growth factor A isoform 121-gelonin fusion protein–conjugated manganese ferrite nanoparticles. 2011 Jul 6 [Updated 2011 Aug 17]. In: Molecular Imaging and Contrast Agent Database (MICAD) [Internet]. Bethesda (MD): National Center for Biotechnology Information (US); 2004-2013.