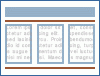
Table
Rodents
Background
[PubMed]
The αυβ3 integrin, also known as the vitronectin receptor, is a heterodimeric transmembrane glycoprotein found on most cells originating from mesenchyme (1). This receptor is often overexpressed in various tumor cells, including osteosarcomas, neuroblastomas, glioblastomas, invasive melanomas, and carcinomas of the lung, breast, prostate, and bladder. Many extracellular matrix proteins such as fibronectin, vitronectin, thrombospondin, fibrinogen, osteopontin, and tenascin are known to be involved in interactions with various subtypes of integrins. These proteins may contain a variety of motifs for potential cell binding; however, one of the most frequent cell-recognition motifs includes an amino acid sequence of Arg-Gly-Asp (RGD), called the "universal cell-recognition site” or a "versatile cell recognition signal” (2). The binding potency of the RGD motif leads to the development of small homing peptides, whose high affinity to the αυβ3 integrin provides a promising alternative to antibodies in targeting tumors (3). As a result, RGD analogs are widely used in tumor imaging, anti-angiogenesis treatment, and tumor-associated radionucleotides or chemotherapeutic drugs. Some RGD analogs are currently being used in phase II clinical trials (4). These αυβ3 integrin–specific probes will help oncologists improve the delineation of tumors and follow up on the progression of anti-angiogenic therapies.
Single-walled carbon nanotubes (SWNTs) are composed of a single graphene sheet rolled into a tubular shape with a diameter of 0.4–2.0 nm and a length of 20–1,000 nm (5). Because of the graphene structure and seeming one-dimensionality, SWNTs exhibit unique thermal, physical, optical, and electrical properties (6). These properties are closely related to two integers (n, m), where n defines the length of the nanotube and m defines the chiral angle of rolling-up. For instance, SWNTs with the difference of n and m (n-m) evenly divisible by 3 belong to semiconductors and can generate a band gap–related fluorescence when suspended in solutions with surfactant micelles (7). The band gap of 1 eV corresponds to the “biological window” (700–1,300 nm) in fluorescence, where absorption, scattering, and autofluorescence by tissues, blood, and water are at a minimum (8). These optical properties make SWNTs appealing as a contrast agent for near-infrared (NIR) bioluminescence imaging and as an optical absorption agent (8) for photoacoustic imaging (9). In photoacoustic imaging, short pulses of stimulating radiation are absorbed by chromophores in tissues, resulting a subsequent thermal expansion and ultrasonic emission that can be detected by highly sensitive piezoelectric devices (10). Photoacoustic imaging as an emerging modality combines the high optical absorption contrast with diffraction-limited resolution of ultrasonic imaging. In addition, the inherent structure of graphene makes SWNTs an excellent contrast agent for Raman imaging (11). The tangential vibrations in the nanotubes generate diameter-selective resonance Raman scattering that is characterized by a major Raman peak located at the G-band (~1,590 cm-1). SWNTs prepared by various methodologies are hydrophobic. The SWNT surface can be modified with surfactants to increase solubility in aqueous solutions or functionalized with bioactive ligands to target specific sites in tissues (12). Cyclic RGD-polyethyleneglycol-SWNTs (RGD-PEG-SWNTs) are functionalized SWNTs used for Raman imaging (11) or photoacoustic imaging (9) of αυβ3 integrin.
Synthesis
[PubMed]
Liu et al. briefly described the synthesis of RGD-SWNTs (13). Initially, SWNTs were prepared with the use of raw gas-phase decomposition of high-pressure carbon monoxide (HiPCO). The obtained SWNTs were sonicated in an aqueous solution of 1,2-distearoyl-sn-glycero-3-phosphoethanolamine-N-(amine(polyethyleneglycol)2000) (DSPE-PEG2000-NH2) or 1,2-distearoyl-sn-glycero-3-phosphoethanolamine-N-(amine(polyethyleneglycol)5400) (DSPE-PEG5400-NH2) for 1 h followed by centrifugation at 24,000 g for 6 h to functionalize SWNTs. The produced SWNT-PEG-NH2 was further conjugated with sulphosuccinimidyl 4-N-maleimidomethyl cyclohexane-1-carboxylateat (sulpho-SMCC) (pH 7.2) for 2 h then reacted with thiolated RGD (pH 7.4) overnight, yielding RGD-PEG2000-SWNTs or RGD-PEG5400-SWNTs.
Animal Studies
Rodents
[PubMed]
Liu et al. used ex vivo biodistribution and in vivo positron emission tomography (PET) to examine the biodistribution of RGD-PEG5400-SWNTs via 64Cu labeling (13). In both studies, nude mice bearing U87MG tumors received injections of ~200–300 mCi 64Cu-labeled RGD-PEG5400-SWNTs. For the ex vivo measurement of biodistribution, the mice were euthanized 24 h post-injection (p.i.), and the tissues were harvested for γ-counting. The tumor uptake increased rapidly, reached a plateau at 6 h p.i., and leveled off in the next 20 h. The measured radioactivity in tumors was 10–15% injected dose (ID)/g for RGD-PEG5400-SWNTs, compared to the 3–4% for PEG5400-SWNTs. The uptake in other tissues was found to be ~20% ID/g in liver, ~8% ID/g in kidney, and <2% ID/g in blood. As a control, 15 mg/kg c(RGDyK) was co-administrated as a block agent with 64Cu-labeled RGD-PEG5400-SWNTs, which significantly reduced the tumor uptake of RGD-PEG5400-SWNTs. In addition, mice bearing integrin αυβ3–negative tumors (HT-29) were injected with 64Cu-labeled RGD-PEG5400-SWNTs. No apparent tumor uptake was found. Liu et al. also examined the biodistribution of RGD-PEG2000-SWNTs using the same approach (13). Compared to 64Cu-labeled RGD-PEG5400-SWNTs, RGD-PEG2000-SWNTs exhibited a lower uptake in U86MG tumors (~5% ID/g) and a higher uptake in the liver (~30% ID/g). To confirm the results, mice bearing U87MG tumors were injected with a high dose of RGD-PEG5400-SWNTs (0.5 mg/kg) and euthanized 8 h p.i. The tumoral and liver tissues were excised for Raman spectroscopic measurement. A high intensity at the G-band (~1,580 cm-1) was found in these tissues, validating the presence of SWNTs. These results demonstrated that the uptake occurred via the reticuloendothelial system (RES), very similar to uptake of nanoparticles 10–100 nm in diameter.
Keren et al. used RGD-PEG-SWNTs to obtain in vivo Raman imaging (11). Mice bearing U87MG tumors were injected intravenously with a 200-μl solution containing 60 pmol RGD-PEG-SWNTs. Raman images were collected 24 h p.i. at 1,593 cm-1. A strong Raman signal was found in the tumoral area. As a control, mice bearing U87MG tumors were intravenously injected with PEG-SWNTs and examined at the same imaging conditions. No apparent accumulation was found in the tumor area. De la Zerda et al. used RGD-PEG-SWNTs to obtain in vivo photoacoustic imaging (9). Mice (n = 4) bearing U87MG tumors (~100 mm3) were injected intravenously with a 200-μl solution at a concentration of 1.2 μM RGD-PEG-SWNTs. Three-dimensional ultrasound and photoacoustic images were collected for the tumors and their surrounding tissues before injection and up to 4 h p.i. A consistent increase in the photoacoustic signal of the tumor was observed. In comparison, the signal increase as a result of PEG-SWNTs was transient. The accumulation of RGD-PEG-SWNTs in the tumors was found three to five times higher than that of PEG-SWNTs, yielding an approximately eight-fold increase in photoacoustic signal.
Human Studies
[PubMed]
No publication is currently available.
NIH Support
CA 119367, EB000312, CA 114747, CA 09695
References
- 1.
- Haubner R. , Finsinger D. , Kessler H. Stereoisomeric peptide libaries and peptidomimetics for designing selective inhibitors of the aυβ3 integrin for a new cancer therapy. Angew. Chem. Int. Ed. Engl. 1997; 36 :1375–1389.
- 2.
- Ruoslahti E. , Pierschbacher M.D. Arg-Gly-Asp: a versatile cell recognition signal. Cell. 1986; 44 (4):517–8. [PubMed: 2418980]
- 3.
- Zitzmann S. , Ehemann V. , Schwab M. Arginine-glycine-aspartic acid (RGD)-peptide binds to both tumor and tumor-endothelial cells in vivo. Cancer Res. 2002; 62 (18):5139–43. [PubMed: 12234975]
- 4.
- Garanger E. , Boturyn D. , Dumy P. Tumor targeting with RGD peptide ligands-design of new molecular conjugates for imaging and therapy of cancers. Anticancer Agents Med Chem. 2007; 7 (5):552–8. [PubMed: 17896915]
- 5.
- Lacerda L. , Bianco A. , Prato M. , Kostarelos K. Carbon nanotubes as nanomedicines: from toxicology to pharmacology. Adv Drug Deliv Rev. 2006; 58 (14):1460–70. [PubMed: 17113677]
- 6.
- Welsher K. , Liu Z. , Daranciang D. , Dai H. Selective probing and imaging of cells with single walled carbon nanotubes as near-infrared fluorescent molecules. Nano Lett. 2008; 8 (2):586–90. [PubMed: 18197719]
- 7.
- Bachilo S.M. , Strano M.S. , Kittrell C. , Hauge R.H. , Smalley R.E. , Weisman R.B. Structure-assigned optical spectra of single-walled carbon nanotubes. Science. 2002; 298 (5602):2361–6. [PubMed: 12459549]
- 8.
- Choi J.H. , Nguyen F.T. , Barone P.W. , Heller D.A. , Moll A.E. , Patel D. , Boppart S.A. , Strano M.S. Multimodal biomedical imaging with asymmetric single-walled carbon nanotube/iron oxide nanoparticle complexes. Nano Lett. 2007; 7 (4):861–7. [PMC free article: PMC6438168] [PubMed: 17335265]
- 9.
- De la Zerda A. , Zavaleta C. , Keren S. , Vaithilingam S. , Bodapati S. , Liu Z. , Levi J. , Smith B.R. , Ma T.J. , Oralkan O. , Cheng Z. , Chen X. , Dai H. , Khuri-Yakub B.T. , Gambhir S.S. Carbon nanotubes as photoacoustic molecular imaging agents in living mice. Nat Nanotechnol. 2008; 3 (9):557–62. [PMC free article: PMC2562547] [PubMed: 18772918]
- 10.
- Wang L. , Xie X. , Oh J.T. , Li M.L. , Ku G. , Ke S. , Similache S. , Li C. , Stoica G. Combined photoacoustic and molecular fluorescence imaging in vivo. Conf Proc IEEE Eng Med Biol Soc. 2005; 1 :190–2. [PubMed: 17282143]
- 11.
- Keren S. , Zavaleta C. , Cheng Z. , de la Zerda A. , Gheysens O. , Gambhir S.S. Noninvasive molecular imaging of small living subjects using Raman spectroscopy. Proc Natl Acad Sci U S A. 2008; 105 (15):5844–9. [PMC free article: PMC2299220] [PubMed: 18378895]
- 12.
- Prato M. , Kostarelos K. , Bianco A. Functionalized carbon nanotubes in drug design and discovery. Acc Chem Res. 2008; 41 (1):60–8. [PubMed: 17867649]
- 13.
- Liu Z. , Cai W. , He L. , Nakayama N. , Chen K. , Sun X. , Chen X. , Dai H. In vivo biodistribution and highly efficient tumour targeting of carbon nanotubes in mice. Nat Nanotechnol. 2007; 2 (1):47–52. [PubMed: 18654207]
Publication Details
Author Information and Affiliations
Publication History
Created: October 6, 2008; Last Update: November 17, 2008.
Copyright
Publisher
National Center for Biotechnology Information (US), Bethesda (MD)
NLM Citation
Zhang H. Cyclic Arg-Gly-Asp-polyethyleneglycol-single-walled carbon nanotubes. 2008 Oct 6 [Updated 2008 Nov 17]. In: Molecular Imaging and Contrast Agent Database (MICAD) [Internet]. Bethesda (MD): National Center for Biotechnology Information (US); 2004-2013.