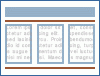
Table
In vitro Rodents
Background
[PubMed]
Many diseases affect the sympathetic nervous system (SNS), and imaging of pathological changes of adrenergic transmission has been an important area of radiopharmaceutical research (1, 2). Most postganglionic sympathetic neurons in the autonomic nervous system release the neurotransmitter norepinephrine (NE), which stimulates adrenergic receptors in various effector organs (3). There are different types and subtypes of adrenergic receptors, and they are characterized as α1a to α1c, α2a to α2c, and β1 to β3 (4). All of the NE adrenergic receptors belong to the G-protein–linked receptor superfamily and mediate slow neuromodulatory postsynaptic responses. The NE transporter (NET) is a transmembrane protein located in the adrenergic nerve terminals, and it is responsible for active reuptake (uptake-1) of NE released from neurons (5). NE is stored in the neuronal vesicles and is released on stimulation. Significant expression of NET is found in major organs of the SNS, such as the heart and brain. There is substantial evidence that aberrations in cardiac SNS function contribute to the morbidity and mortality associated with cardiac diseases (6). Brain NET is involved in various neurological and psychiatric diseases, including depression, attention deficit hyperactivity disorder, drug addiction, and eating disorders (7). NET is also the site of action in the brain for many antidepressant drugs (8).
Molecular probes with structures closely related to NE can be used to assess the integrity of presynaptic sympathetic nerve terminals in various diseases. In vivo NE synthesis is similar to dopamine synthesis, and dopamine is converted to NE by the enzyme dopamine-β-hydroxylase (4). [123I]-meta-Iodobenzylguanidine, [11C]meta-hydroxyephedrine, [11C]norepinephrine, and many other radioligands have been developed and used for peripheral neuronal imaging (9). However, this class of tracers is not suitable for the study of the brain NET system because they are not able to cross the blood–brain barrier (10). In the brain, NET levels are relatively low compared with those of other transporters, such as dopamine transporter (DAT) and serotonin transporter (SERT) (8). Several NET reuptake inhibitors such as [11C]desipramine have been tested, but they showed high nonspecific binding. Reboxetine ((RS)-2-[((RS)-2-ethoxyphenoxy)benzyl]morpholine) is a specific NET inhibitor with a high affinity and selectivity. Reboxetine is available as a racemic mixture of the (R,R) and (S,S) enantiomers. The (S,S) enantiomer has been found to be more potent, with a 50% inhibition concentration (IC50) value of 3.6 nM, for inhibiting NET in rat hypothalamic synaptosomes. Among the different reboxetine derivatives that have been tested, (2S,αS)-2-(α-(2-[125I]iodophenoxy)benzyl)morpholine ((S,S)-[125I]IPBM) is considered a potential candidate to be developed as a single-photon emission computed tomography (SPECT) ligand for studying the brain and heart NET system (11, 12).
Synthesis
[PubMed]
Kanegawa et al. (11) reported the radiosynthesis of (S,S)-[125I]IPBM by a halogen exchange reaction with 125I (2S, 3S). -2-[α-(2-bromophenoxy)benzyl]morpholine was reacted with [125I]NaI in the presence of ammonium sulfate and copper(II) sulfate pentahydrate. The mixture was heated for 45 min at 130ºC. Radiochemical yields were 65%. After purification with high-performance liquid chromatography, the final product had a radiochemical purity of >98%. The specific activity was not reported.
In Vitro Studies: Testing in Cells and Tissues
[PubMed]
Kanegawa et al. (11) showed that inhibition constant (Ki) values of nisoxetine (NET inhibitor), fluoretine (SERT inhibitor), and GBR12909 (DAT inhibitor) were 4.17 ± 1.98, 1,073 ± 437, and >5,000 nM, respectively. The binding assays were performed using the cerebral cortex of male rats and (S,S)-[125I]IPBM. The selectivity ratio of SERT to NET (Ki SERT/Ki NET) was 257 and of DAT to NET (Ki DAT/Ki NET) was >1,000. (S,S)-[125I]IPBM had a binding affinity (Kd) of 1.30 ± 0.46 nM.
Kiyono et al. (12) showed that 50% inhibition concentration (IC50) values of nisoxetine, fluoretine, and GBR12909 were 2.28 ± 0.87, 1,165 ± 204, and >10,000 nM, respectively. The binding assays were performed using rat heart membranes and (S,S)-[125I]IPBM. The selectivity ratio of SERT to NET was 511 and of DAT to NET was >4,000. (S,S)-[125I]IPBM had a Kd value of 1.62 ± 0.22 nM.
Animal Studies
Rodents
[PubMed]
Kanegawa et al. (11) performed ex vivo biodistribution studies in normal rats (n = 4/group) after injection of (S,S)-[125I]IPBM. (S,S)-[125I]IPBM rapidly entered the brain, and the levels of radioactivity in the brain, measured as percent injected dose per gram (% ID/g), was 0.44, 0.51, 0.54, 0.43, and 0.25 at 5, 15, 30, 60, and 180 min after injection, respectively. The radioactivity in blood cleared rapidly. The brain/blood ratio was 14.3 at 60 min after the injection. The organ with the highest initial uptake (5 min after injection) was the lung (5.0% ID/g), followed by the adrenal gland (1.4% ID/g) and kidneys (1.0% ID/g) with gradual to moderate washout rate. The radioactivity that accumulated in the intestines and stomach showed prominent increases at 30–60 min after injection, whereas the radioactivity in the liver was low (<0.3% ID/g). Furthermore, there was low accumulation in the thyroid gland with 0.01% ID/g at 5 min and 0.04% ID/g at 180 min after injection. The radioactivity level in the heart was not measured. Regional brain biodistribution showed a high accumulation in the thalamus, midbrain, cerebellum, and pons (NET-rich regions) and a low accumulation in the striatum. The accumulation of radioactivity in the NET-rich regions peaked at ~30 min after injection. The ratio of radioactivity in the NET-rich regions to the striatum was greatest at 180 min. Ex vivo autoradiographic analyses showed that the highest levels of radioactivity were observed in the locus coeruleus and anteroventricular thalamic nucleus. Co-administration of 10 mg/kg nisoxetine with (S,S)-[125I]IPBM reduced radioactivity levels in the NET-rich regions by >80% in all organs except the striatum. Fluoxetine (10 mg/kg) and GBR12909 (1 mg/kg) exhibited no reduction of radioactivity levels in the NET-rich regions.
Kiyono et al. (12) reported that there was a rapid and moderate uptake of (S,S)-[125I]IPBM by the heart (0.64% ID/g at 60 min) in rats. The heart/blood ratio increased with time, with a maximum value of 31.9 at 180 min after injection. Co-administration of nisoxetine (1 mg/kg) with (S,S)-[125I]IPBM led to a 60% reduction in radioactivity level in the heart, whereas fluoxetine (1 mg/kg) and GBR12909 (1 mg/kg) exhibited little effect.
Non-Human Primates
[PubMed]
Kanegawa et al. (11) evaluated (S,S)-[125I]IPBM as a radioligand for SPECT imaging studies of brain NET in a female common marmoset with 222 MBq (6 mCi) (S,S)-[125I]IPBM. Imaging studies showed high accumulation in the thalamus, cortex, and cerebellum (NET-rich regions) and low accumulation in the striatum. Treatment with nisoxetine (5 mg/kg) at 68 min after (S,S)-[125I]IPBM injection led to reduced radioactivity levels in the NET-rich regions but not in the striatum. The radioactivity levels in the NET-rich regions were reduced to that of the striatum at 211 min after injection.
References
- 1.
- Konishi, J., B.A. Dwamena, M.D. Gross, B. Shapiro, T. Misaki, M. Fukunaga, J.C. Sisson, H.-Y. Oei, M. De Jong, and E. P. Krenning Endocrinology, in Molecular Nuclear Medicine, L.E. Feinendegen, W.W. Shreeve, W.C. Eckelman, Y.-W. Bahk, and H.N. Wagner Jr., Editor. 2003, Springer: New York. p. 357-409.
- 2.
- Antoni, G., T. Kihlberg, and B. Langstrom, Aspects on the synthesis of 11C-Labelled compounds, in Handbook of Radiopharmaceuticals, M.J. Welch, and C.S. Redvanly, Editor. 2003, John Wiley & Sons Ltd.: West Sussex, England. p. 141-194.
- 3.
- Sunderland, P.M., Pathophysiology. The Biologic basis for disease in adults and children, K.L. McCance, and S. E. Huether, Editor. 1994, Mosby-Year Books, Inc.: St, Louiis. p. 397-436.
- 4.
- Frey, K.A., PET study of neurochemical systems, in Positron Emission Tomography, P.E. Valk, D.L. Bailey, D.W. Townsend, and M.N. Maisey, Editors. 2002, Springer London. p. 309-327.
- 5.
- Buursma A.R., Beerens A.M., de Vries E.F., van Waarde A., Rots M.G., Hospers G.A., Vaalburg W., Haisma H.J. The Human Norepinephrine Transporter in Combination with 11C-m-Hydroxyephedrine as a Reporter Gene/Reporter Probe for PET of Gene Therapy. J Nucl Med. 2005;46(12):2068–75. [PubMed: 16330572]
- 6.
- Caldwell J.H., Kroll K., Li Z., Seymour K., Link J.M., Krohn K.A. Quantitation of presynaptic cardiac sympathetic function with carbon-11-meta-hydroxyephedrine. J Nucl Med. 1998;39(8):1327–34. [PubMed: 9708501]
- 7.
- Zahniser N.R., Doolen S. Chronic and acute regulation of Na+/Cl- -dependent neurotransmitter transporters: drugs, substrates, presynaptic receptors, and signaling systems. Pharmacol Ther. 2001;92(1):21–55. [PubMed: 11750035]
- 8.
- Wilson A.A., Johnson D.P., Mozley D., Hussey D., Ginovart N., Nobrega J., Garcia A., Meyer J., Houle S. Synthesis and in vivo evaluation of novel radiotracers for the in vivo imaging of the norepinephrine transporter. Nucl Med Biol. 2003;30(2):85–92. [PubMed: 12623106]
- 9.
- Langer O., Halldin C. PET and SPET tracers for mapping the cardiac nervous system. Eur J Nucl Med Mol Imaging. 2002;29(3):416–34. [PubMed: 12002720]
- 10.
- Ding Y.S., Lin K.S., Logan J., Benveniste H., Carter P. Comparative evaluation of positron emission tomography radiotracers for imaging the norepinephrine transporter: (S,S) and (R,R) enantiomers of reboxetine analogs ([11C]methylreboxetine, 3-Cl-[11C]methylreboxetine and [18F]fluororeboxetine), (R)-[11C]nisoxetine, [11C]oxaprotiline and [11C]lortalamine. J Neurochem. 2005;94(2):337–51. [PubMed: 15998285]
- 11.
- Kanegawa N., Kiyono Y., Kimura H., Sugita T., Kajiyama S., Kawashima H., Ueda M., Kuge Y., Saji H. Synthesis and evaluation of radioiodinated (S,S)-2-(alpha-(2-iodophenoxy)benzyl)morpholine for imaging brain norepinephrine transporter. Eur J Nucl Med Mol Imaging. 2006;33(6):639–47. [PubMed: 16523308]
- 12.
- Kiyono Y., Sugita T., Ueda M., Kawashima H., Kanegawa N., Kuge Y., Fujibayashi Y., Saji H. Evaluation of radioiodinated (2S,alphaS)-2-(alpha-(2-iodophenoxy)benzyl)morpholine as a radioligand for imaging of norepinephrine transporter in the heart. Nucl Med Biol. 2008;35(2):213–8. [PubMed: 18312831]
Publication Details
Author Information and Affiliations
Publication History
Created: February 19, 2009; Last Update: May 15, 2009.
Copyright
Publisher
National Center for Biotechnology Information (US), Bethesda (MD)
NLM Citation
Leung K. (2S,αS)-2-(α-(2-[125I]Iodophenoxy)benzyl)morpholine. 2009 Feb 19 [Updated 2009 May 15]. In: Molecular Imaging and Contrast Agent Database (MICAD) [Internet]. Bethesda (MD): National Center for Biotechnology Information (US); 2004-2013.