By agreement with the publisher, this book is accessible by the search feature, but cannot be browsed.
NCBI Bookshelf. A service of the National Library of Medicine, National Institutes of Health.
Alberts B, Johnson A, Lewis J, et al. Molecular Biology of the Cell. 4th edition. New York: Garland Science; 2002.
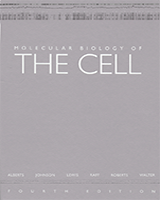
Molecular Biology of the Cell. 4th edition.
Show detailsAs development proceeds, the body becomes more and more complex. In all this growing complexity there is, however, a simplifying feature that puts an understanding of the whole developmental process within our grasp. Again and again, in every species and at every level of organization, we find that complex structures are made by repeating a few basic themes with variations. Thus a limited number of basic differentiated cell types, such as muscle cells or fibroblasts, recur with subtle individual variations in different sites. These cell types are organized into a limited variety of tissue types, such as muscle or tendon, which again are repeated with subtle variations in different regions of the body. From the various tissues, organs such as teeth or digits are built—molars and incisors, fingers and thumbs and toes—a few basic kinds of structure, repeated with variations.
Wherever we find this phenomenon of modulated repetition, we can break down the developmental biologist's problem into two kinds of question: what is the basic construction mechanism common to all the objects of the given class, and how is this mechanism modified to give the observed variations? The embryo uses a combinatorial strategy to generate its complexity, and we can use a combinatorial strategy to understand it.
The segments of the insect body provide a very clear example. We have already sketched the way in which the rudiment of a single typical segment is constructed. We must now consider how one segment is caused to be different from another.
The HOX Code Specifies Anterior-Posterior Differences
The first glimpse of a genetic answer to the question of how each segment acquires its individual identity came over 80 years ago, with the discovery of the first of a set of mutations in Drosophila that cause bizarre disturbances of the organization of the adult fly. In the Antennapedia mutant, for example, legs sprout from the head in place of antennae (Figure 21-41), while in the bithorax mutant, portions of an extra pair of wings appear where normally there should be the much smaller appendages called halteres. These mutations transform parts of the body into structures appropriate to other positions and are called homeotic. A whole set of homeotic selector genes determines the anteroposterior character of the segments of the fly.
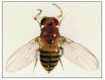
Figure 21-41
A homeotic mutation. The fly shown here is an Antennapedia mutant. Its antennae are converted into leg structures by a mutation in the regulatory region of the Antennapedia gene that causes it to be expressed in the head. Compare with the normal fly shown (more...)
The genes of this set—eight of them in the fly—are all related to one another as members of a multigene family, and they all lie in one or the other of two tight gene clusters known as the bithorax complex and the Antennapedia complex. The genes in the bithorax complex control the differences among the abdominal and thoracic segments of the body, while those in the Antennapedia complex control the differences among thoracic and head segments. Comparisons with other species show that the same genes are present in essentially all animals, including humans. These comparisons also reveal that the Antennapedia and bithorax complexes are the two halves of a single entity, called the Hox complex, that has become split in the course of the fly's evolution, and whose members operate in a coordinated way to exert their control over the head-to-tail pattern of the body.
Homeotic Selector Genes Code for DNA-Binding Proteins That Interact with Other Gene Regulatory Proteins
To a first approximation each homeotic selector gene is normally expressed in just those regions that develop abnormally when the gene is mutated or absent. The products of these genes can thus be viewed as molecular address labels possessed by the cells of each parasegment: they are the physical embodiment of the cells' positional value. If the address labels are changed, the parasegment behaves as though it were located somewhere else, and deletion of the entire complex results in a larva whose body segments are all alike (Figure 21-42).
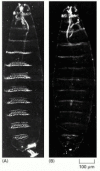
Figure 21-42
The effect of deleting most of the genes of the bithorax complex. (A) A normal Drosophila larva shown in dark-field illumination; (B) the mutant larva with the bithorax complex largely deleted. In the mutant the parasegments posterior to P5 all have the (more...)
A first problem, therefore, is to understand how the homeotic selector gene products act on the basic segment-patterning machinery to give each parasegment its individuality. The products of the homeotic selector genes are gene regulatory proteins, all related to one another by the possession of a highly conserved DNA-binding homeodomain (60 amino acids long), discussed in Chapter 7. The corresponding segment in the DNA sequence is called a homeobox from which, by abbreviation, the Hox complex takes its name.
If the products of the homeotic selector genes are similar in their DNA-binding regions, how do they exert different effects so as to make one parasegment different from the next? The answer seems to lie largely in the parts of the proteins that do not bind directly to DNA but interact with other proteins in DNA-bound complexes. The different partners in these complexes act together with the homeotic selector proteins to dictate which DNA-binding sites will be recognized and whether the effect on transcription at those sites will be activation or repression. In this way, the products of the homeotic selector genes combine with other gene regulatory proteins and modulate their actions so as to give each parasegment its characteristic features.
The Homeotic Selector Genes Are Expressed Sequentially According to Their Order in the Hox Complex
To understand how the Hox complex provides cells with positional values, we also need to consider how the expression of the Hox genes themselves is regulated. The coding sequences of the eight homeotic selector genes in the Antennapedia and bithorax complexes are interspersed amid a much larger quantity—a total of about 650,000 nucleotide pairs—of regulatory DNA. This DNA includes binding sites for the products of egg-polarity and segmentation genes. The regulatory DNA in the Hox complex acts as an interpreter of the multiple items of positional information supplied to it by all these gene regulatory proteins. In response, a particular set of homeotic selector genes is transcribed, appropriate to the location.
In the pattern of control there is a remarkable regularity. The sequence in which the genes are ordered along the chromosome, in both the Antennapedia and the bithorax complexes, corresponds almost exactly to the order in which they are expressed along the axis of the body (Figure 21-43). This suggests that the genes are activated serially by some process that is graded—in duration or intensity—along the axis of the body, and whose action spreads gradually along the chromosome. The most “posterior” of the genes expressed in a cell generally dominates, driving down expression of the previously activated “anterior” genes and dictating the character of the segment. The gene regulatory mechanisms underlying these phenomena are still not well understood, but their consequences are profound. We shall see that the serial organization of gene expression in the Hox complex is a fundamental feature that has been highly conserved in the course of evolution.
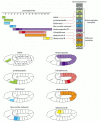
Figure 21-43
The patterns of expression compared to the chromosomal locations of the genes of the Hox complex. The sequence of genes in each of the two subdivisions of the chromosomal complex corresponds to the spatial sequence in which the genes are expressed. Note (more...)
There are hundreds of other homeobox-containing genes in the genome of the fly—and of other animal species—but most of them are scattered and not clustered in complexes such as the Hox complex. They have many different gene regulatory functions, but a substantial proportion of them have roles akin to that of the Hox genes: they control the variations on a basic developmental theme. Different classes of neurons, for example, are often distinguished from one another by expression of specific genes of this large superfamily.
The Hox Complex Carries a Permanent Record of Positional Information
The spatial pattern of expression of the genes in the Hox complex is set up by signals acting early in development, but the consequences are long-lasting. Although the pattern of expression undergoes complex adjustments as development proceeds, the Hox complex behaves in each cell as though stamped with a permanent record of the anteroposterior position that the cell occupied in the early embryo. In this way, the cells of each segment are equipped with a long-term memory of their location along the anteroposterior axis of the body—in other words, with an anteroposterior positional value. As we shall see in the next section, the memory trace imprinted on the Hox complex governs the segment-specific identity not only of the larval segments, but also of the structures of the adult fly, which are generated at a much later stage from the larval imaginal discs and other nests of imaginal precursor cells in the larva.
The molecular mechanism of the cell memory for this positional information relies on two types of regulatory inputs. One is from the homeotic selector genes themselves: many of the Hox proteins autoactivate the transcription of their own genes. Another crucial input is from two large complementary sets of transcriptional regulators called the Polycomb group and the Trithorax group. If these regulators are defective, the pattern of expression of the homeotic selector genes is set up correctly at first but is not correctly maintained as the embryo grows older.
The two sets of regulators act in opposite ways. Trithorax group proteins are needed to maintain the transcription of Hox genes in cells where transcription has already been switched on. In contrast, Polycomb group proteins form stable complexes that bind to the chromatin of the Hox complex and maintain the repressed state in cells where Hox genes have not been activated at the critical time (Figure 21-44). The developmental memory involves acetylation of histone H4 at specific regulatory sites in the chromatin adjacent to the Hox genes; the proteins of the Trithorax and Polycomb groups act somehow to perpetuate the H4 state—hyperacetylated if the target Hox gene has been transiently exposed at embryonic stages to an activator of gene transcription, not acetylated in this way otherwise (see Figure 4-48).
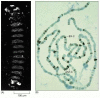
Figure 21-44
Action of genes of the Polycomb group. (A) Photograph of a mutant embryo defective for the gene extra sex combs (esc) and derived from a mother also lacking this gene. The gene belongs to the Polycomb group. Essentially all segments have been transformed (more...)
The Anteroposterior Axis Is Controlled by Hox Selector Genes in Vertebrates Also
Homologs of the Drosophila homeotic selector genes have been found in almost every animal species studied, from cnidarians (hydroids) and nematodes to molluscs and mammals. Remarkably, these genes are often grouped in complexes similar to the insect Hox complex. In the mouse there are four such complexes—called the HoxA, HoxB, HoxC, and HoxD complexes—each on a different chromosome. Individual genes in each complex can be recognized by their sequences as counterparts of specific members of the Drosophila set. Indeed, mammalian Hox genes can function in Drosophila as partial replacements for the corresponding Drosophila Hox genes. It appears that each of the four mammalian Hox complexes is, roughly speaking, the equivalent of a complete insect complex (that is, an Antennapedia complex plus a bithorax complex) (Figure 21-45).
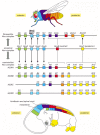
Figure 21-45
The Hox complex of an insect and the Hox complexes of a mammal compared and related to body regions. The genes of the Antennapedia and bithorax complexes of Drosophila are shown in their chromosomal order in the top line; the corresponding genes of the (more...)
The ordering of the genes within each vertebrate Hox complex is essentially the same as in the insect Hox complex, suggesting that all four vertebrate complexes originated by duplications of a single primordial complex and have preserved its basic organization. Most tellingly, when the expression patterns of the Hox genes are examined in the vertebrate embryo by in situ hybridization, it turns out that the members of each complex are expressed in a head-to-tail series along the axis of the body, just as they are in Drosophila (Figure 21-46). The pattern is most clearly seen in the neural tube, but is also visible in other tissues, especially the mesoderm. With minor exceptions this anatomical ordering matches the chromosomal ordering of the genes in each complex, and corresponding genes in the four different Hox complexes have almost identical anteroposterior domains of expression.
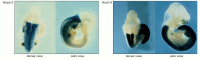
Figure 21-46
Expression domains of Hox genes in a mouse. The photographs show whole embryos displaying the expression domains of two genes of the HoxB complex (blue stain). These domains can be revealed by in situ hybridization or, as in these examples, by constructing (more...)
The gene expression domains define a detailed system of correspondences between insect body regions and vertebrate body regions (see Figure 21-45). The parasegments of the fly correspond to a similarly labeled series of segments in the anterior part of the vertebrate embryo. As shown in Figure 21-47, these are most clearly demarcated in the hindbrain, where they are called rhombomeres. In the tissues lateral to the hindbrain the segmentation is seen in the series of branchial arches, prominent in all vertebrate embryos—the precursors of the system of gills in fish and of the jaws and structures of the neck in mammals; each pair of rhombomeres in the hindbrain corresponds to one branchial arch (Figure 21-47). In the hindbrain, as in Drosophila, the boundaries of the expression domains of many of the Hox genes are aligned with the boundaries of the anatomical segments.
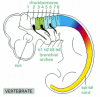
Figure 21-47
Segmentation and Hox gene expression in the hindbrain, as seen in a chick embryo. The pattern of HoxB gene expression is indicated by coloring as in Figure 21-45. For simplicity, the expression in tissues other than the central nervous system is not shown. (more...)
The products of the mammalian Hox genes appear to specify positional values that control the anteroposterior pattern of parts in the hindbrain, neck, and trunk. Eliminating the function of a Hox gene in the mouse leads to a defect in a body region that corresponds to the domain of expression of that gene. Sometimes the affected body region is transformed into a more anterior body region, as in Drosophila homeotic mutants; sometimes the affected body region dies or fails to grow. The transformations observed in mouse Hox mutants are often incomplete, perhaps because of a redundancy between genes in the four Hox gene clusters. But it seems clear that the fly and the mouse use essentially the same molecular machinery to give individual characters to successive regions along at least a part of their anteroposterior axis.
Summary
The complexity of the adult body of an animal is built up by modulated repetition of a few basic types of structure. Thus, superimposed on the pattern of gene expression that repeats itself in every segment, there is a serial pattern of expression of homeotic selector genes that confer on each segment a different identity. The homeotic selector genes code for DNA-binding proteins of the homeodomain family. They are grouped in the Drosophila genome in two clusters, called the Antennapedia and bithorax complexes, believed to be the two parts of a single primordial Hox complex that became split during evolution of the fly. In each complex, the genes are arranged in a sequence that matches their sequence of expression along the axis of the body. Hox gene expression is initiated in the embryo. It is maintained subsequently by the action of DNA-binding proteins of the Polycomb and Trithorax group, which stamp the chromatin of the Hox complex with a heritable record of its embryonic state of activation. Hox complexes homologous to that of Drosophila are found in virtually every type of animal that has been examined, from cnidarians to humans, and they appear to have an evolutionarily conserved role in patterning the anteroposterior axis of the body. Mammals have four Hox complexes, each showing a similar relationship between a serial arrangement of the genes in the chromosome and their serial pattern of expression along the body axis.
- The HOX Code Specifies Anterior-Posterior Differences
- Homeotic Selector Genes Code for DNA-Binding Proteins That Interact with Other Gene Regulatory Proteins
- The Homeotic Selector Genes Are Expressed Sequentially According to Their Order in the Hox Complex
- The Hox Complex Carries a Permanent Record of Positional Information
- The Anteroposterior Axis Is Controlled by Hox Selector Genes in Vertebrates Also
- Summary
- Homeotic Selector Genes and the Patterning of the Anteroposterior Axis - Molecul...Homeotic Selector Genes and the Patterning of the Anteroposterior Axis - Molecular Biology of the Cell
Your browsing activity is empty.
Activity recording is turned off.
See more...