NCBI Bookshelf. A service of the National Library of Medicine, National Institutes of Health.
Liedtke WB, Heller S, editors. TRP Ion Channel Function in Sensory Transduction and Cellular Signaling Cascades. Boca Raton (FL): CRC Press/Taylor & Francis; 2007.
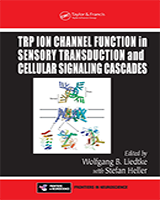
TRP Ion Channel Function in Sensory Transduction and Cellular Signaling Cascades.
Show detailsAbstract
Epithelial layers, such as those lining the airways or the gut, are normally involved in a barrier function as well as in transporting ions and nutrients. Both activities require the polarized distribution of proteins, particularly transport proteins (e.g., ion channels), in luminal and basolateral membranes. Most of the participating transport proteins have already been identified, and newcomers are received with expectation. Transient receptor potential (TRP) channels are good examples of novel cation transport proteins whose relevance in epithelial physiology is just starting to emerge. In this chapter we focus on the role of the TRPV4 channel in ciliated epithelia.
CELL CALCIUM SIGNALING IN EPITHELIA
Many epithelial functions, including absorption, secretion, volume homeostasis and response to pathogens, are linked to changes in intracellular calcium [1]. Ciliated epithelia carry out an additional task, the transport of mucus and trapped particles [2], in which Ca2+ signaling is also a key element of regulation (see below). The calcium signal may originate via the activation of entry pathways at the plasma membrane or the release from intracellular stores. Entry of Ca2+ is mainly driven by a favorable electrochemical gradient and the opening of Ca2+-permeable ion channels. We know the most about voltage-gated Ca2+ channels operating in excitable cells. However, only recently the molecular nature of the channels mediating Ca2+ entry in nonexcitable cells, the family of TRP channels, has been identified. Here we discuss the role of TRP channels, particularly the TRPV4 channel, in generating the Ca2+ signal involved in the regulation of epithelial cell volume and ciliary activity.
TRP CHANNELS IN EPITHELIA
The TRP family of channels contains numerous members (27 to date) that present structural similarities [3]. The general topology of a TRP subunit consists of six predicted transmembrane domains (TM) with a putative pore loop between the fifth and sixth TMs and intracellular N- and C-terminal regions of variable length. The N-terminal region contains multiple ankyrin repeats in the TRPC, TRPV, TRPA, and TRPN subfamilies, and the C-terminal region presents a domain with enzymatic activity in some members of the TRPM subfamily [3]. Several members of the TRP family of channels have been found in epithelial tissues: airways (TRPC1, 4, 6; [4] TRPV2, 4 [5,6]), intestine and pancreas (TRPV5–6 [7]), female reproductive tract (TRPV4 and TRPP1, 2 [8,9]), kidney (TRPM3, 6–7; [10,11] TRPV1, 4–6; [12–14] TRPP1–2 [15]), prostate (TRPM8; [16] TRPV5–6 [7]), inner ear hair cells (TRPA1; [17] TRPV4; [18] TRPN1 [19]), cornea (TRPC4 [20]), and epidermal ciliated cells (TRPN1 [19]). Of particular interest to this chapter is the contribution of the TRPV4 channel to the physiology of ciliated epithelia. TRPV4 was first identified as a nonselective cation channel rapidly activated under hypotonic conditions when expressed heterologously (reviewed in reference [21]). Later studies showed that TRPV4 channels present multiple activating and regulatory sites that allow them to integrate distinct physical and chemical stimuli from the environment, offering a wide range of possible physiological roles. TRPV4 channels also respond to mechanical stress, heat, acidic pH, endogenous ligands, and synthetic agonists such as 4α-phorbol 12,13-didecanoate (4α-PDD) [21]. TRPV4 mRNA and protein have been identified in both native ciliated epithelial cells of female reproductive organs [8,9] and in cell lines derived from human ciliated airway cells [6] where the channel plays key roles in cell volume homeostasis and regulation of ciliary activity. Novel spliced variants of TRPV4 have also been found in airway epithelial cell lines [22]. Some of these variants form functional ion channels while others do not oligomerize and are retained intracellularly.
THE CILIATED EPITHELIUM
Many epithelial cells lining the airways and the reproductive tract contain projections called cilia, whose function is, in the case of the airways, brushing away foreign particles, pathogens, and allergens. Failure of this ciliary action contributes to respiratory pathology. Similarly, the fallopian tube (oviduct), connecting the uterus with the ovaries, transports oocytes and embryos, an essential function for reproduction that also depends on ciliary activity [23]. Motile cilia, such as those present in the airways and oviduct, are membrane-delimited extensions of the cell surface of approximately 5–10 μm in length; the motor is a tubulin-based axoneme. The axoneme of each cilia shows nine outer doublets of microtubules and a single central pair of microtubules (9 + 2 structure) formed by heterodimers of α and β tubulin. An important molecule within the cilium structure is the ATPase dynein, a motor linking and displacing two adjacent external doublets, thereby responsible for the cilium movement [2]. In contrast to motile cilia, inmotile cilia appear as one cilium per cell (e.g., kidney primary cilia), contain a 9 + 0 axonemal structure, and lack dynein arms [23]. An exception is the nodal cilia that express dynein and exhibit a twirling movement.
Airway surface microanatomy has received considerable attention over the last years due to its alteration in diseases such as cystic fibrosis [24]. It consists of at least two layers: an upper mucus layer (a gel-like polymer network of high-molecular weight mucins) and a lower periciliary liquid layer (PCL) that protects the epithelial cell surface from the mucus layer. Ciliated and mucus-secreting cells form a structural and functional unit and work as a conveyor-belt system for particle transport. In this analogy, cilia provide the power, while the mucus serves as the belt. Under normal physiological conditions, ciliated cells should be able to cope with modifications in the composition and volume of both compartments, mucus layer, and PCL. Failure to do so may result in pathology [24].
TRPV4 AND CELL VOLUME REGULATION IN CILIATED EPITHELIA
Cell volume regulation is an important homeostatic mechanism through which cells are able to maintain optimal cell volume in an environment where the extracellular osmotic pressure differs from the intracellular. Cells exposed to a hypotonic extracellular solution (lower relative solute concentration) will initially swell and then recover their original volume. This process is termed regulatory volume decrease (RVD). Conversely, cells exposed to a hypertonic solution (higher relative solute concentration) will initially shrink but then recover their volume to the original size, a process known as regulatory volume increase (RVI). In the absence of extracellular environment modifications, epithelial cell metabolism and transport processes can also unbalance the finely tuned osmotic pressure of the cell through the release or generation of osmolytes [25]. Although the ability to maintain a constant volume in the face of osmotic stress is important for all cells in the body, the process assumes particular significance in epithelial cells. In airways, the luminal face of the epithelial cells is covered by PCL, which modifies its osmolarity under different situations, becoming hyperosmolar (e.g., during cold or dry ventilation) or hypoosmolar (e.g., breathing fog) [26]. Similarly, osmolarity of oviductal fluid also changes with the ovarian cycle [27].
The RVD response typically involves the coordinated activation of Cl− and K+channels, which permit the passive loss of electrolytes and osmotically obliged water. Cell volume regulation following hypotonic or isotonic swelling in epithelial cells is normally associated with changes in intracellular Ca2+ concentrations [28] and subsequent activation of Ca2+-dependent ion channels, most likely Ca2+-dependent K+ channels (references [29] and [30] and references therein), although exceptions exist [31]. The source of Ca2+ has been difficult to track down, and it varies depending on the stimulus (discussed in reference [29]). Most evidence has suggested increased Ca2+ entry via a stretch- or swelling-activated cation channel and subsequent activation of Ca2+-dependent K+ channels. The cloning of the TRPV4 channel provided a molecular candidate to mediate swelling-activated Ca2+ entry, positioning this channel up in the hierarchy of the signaling cascade triggering RVD. We have demonstrated that TRPV4 is expressed and constitutes the only pathway mediating swelling-activated Ca2+ entry required to achieve full RVD in cell lines derived from ciliated human airway epithelia: CFT1–LCFSN tracheal cells [6] and HBE bronchial cells (unpublished data). In both cell lines, Ca2+ entry activates either intermediate- [30] or large-conductance [29] Ca2+-dependent K+ channels, respectively, therefore coupling Ca2+ entry to effective RVD.
Interestingly, swelling-dependent TRPV4 activation is missing in cystic fibrosis human airway epithelia [6], resulting in impaired Ca2+-dependent K+ channel activation and defective RVD [30]. The actual mechanism explaining the relationship between a defective CFTR Cl− channel protein and TRPV4 as well as the relevance of a defective RVD to cystic fibrosis pathophysiology is unknown at present.
TRPV4 AND REGULATION OF CILIARY BEAT FREQUENCY
It has already been mentioned that mucociliary transport plays a fundamental role in the defense against pathogenic agents and, in the case of the oviduct, in the transport of oocytes and embryos to the uterus [23]. The relevance of these processes is revealed by the identification of defective mucociliary transport in several human respiratory diseases, including cystic fibrosis, asthma, bronchiectasis, and chronic bronchitis [32], as well as in infertility [23]. Alterations in the normal functioning of cilia have also been associated to abnormalities in left–right asymmetry [33] and the appearance of cysts in the kidneys and other epithelial organs [15].
Motile cilia are responsible for mucociliary transport, and a critical factor in the maintenance of appropriate velocity of mucociliary transport is the ciliary beat frequency (CBF) [34]. Several intracellular signals have been demonstrated to mediate the changes of CBF in response to different stimuli: cAMP, cGMP, nitric oxide, and Ca2+ (reference [8] and references therein). Among them, the role of Ca2+ in the control of CBF is particularly interesting, as it has been associated with the ciliary response to mechanical stimuli. Mechanically stimulated ciliated cells increase intracellular Ca2+ and CBF, a response that is lost in the absence of extracellular Ca2+ [35]. The hypothesis that mechanical stimulation might be physiologically initiated by changes in mucus viscosity has been present for quite a while, but the cellular mechanism linking the viscous load exerted by the presence of mucus to the control of CBF has just started to be elucidated. Moreover, according to our recent studies [8], a Ca2+signal links the response of CBF to another important factor determining mucociliary transport: the mucus viscosity, which may change under normal [34,36] and pathological conditions [37].
Mucus-transporting cilia appear to present adaptations that allow them to beat under conditions of varying viscosity, thereby preventing the collapse of mucociliary transport, a process known as autoregulation of CBF [8]. CBF decreases with increasing external viscosity until it reaches a plateau (Figure 30.1). However, in the absence of extracellular Ca2+ or in the presence of 100 μM Gd3+, oviductal ciliated cells lose their ability to maintain CBF at the same plateau level when challenged with high viscous solutions, suggesting that Ca2+ entry is an important element in the CBF autoregulation [8]. This observation prompted us to search for the molecular nature of the Ca2+ entry pathway, as it was the TRP channel’s obvious candidate. Ciliated epithelia of the oviduct express TRPV4 [8] as well as TRPP1 and 2 [9] (also known as PKD1, a regulator of the channel-forming TRPP2 protein [PKD2]) [15]. More interestingly, TRPV4 (Figure 30.2) and TRPPs localize to the cilia, and both TRPV4 and the TRPP channel complex respond to mechanical stimuli [15,38]. However, two pieces of evidence favor the participation of TRPV4 rather than the TRPP channel: (1) no whole-cell cationic current in response to high viscous loading was observed in oviductal ciliated cells dialysed with an anti-TRPV4 antibody, and (2) mechanical activation of the TRPV4 channel, unlike TRPP, requires phospholipase A2 (PLA2) activation and generation of arachidonic acid (AA) [8].

FIGURE 30.1
Effect of viscous load on the ciliary beat frequency (CBF). The CBF dropped ~35 percent within the low viscosity range of 2 to 37 cP (2–15 percent dextran solutions), but no further decrease was observed at higher viscosities (37–200 cP; (more...)

FIGURE 30.2
Confocal microscopy localization of TRPV4 to motile cilia in a tissue section of the female hamster oviduct. (Left) Nomarsky image. (Right) Detection of TRPV4 (green) and TOPRO-3 nuclear staining (blue). The antibody used to detect TRPV4 is described (more...)
CONCLUSIONS
TRPV4 has emerged as a candidate to participate in the coupling of fluid viscosity changes to ciliary beat regulation and in cell volume regulation. TRPV4 activation following hypotonic cell swelling [39] and viscous load mechanical stimulation [8] requires PLA2-dependent formation of AA. These results suggest that the TRPV4 channel is not the volume and mechanosensor per se but is an important piece of the signaling cascade providing the Ca2+ entry pathway. Therefore, it will be interesting to identify the sensor upstream of TRPV4 activation and whether this sensor is shared by the swelling and mechanical-transducing machinery.
Another question to evaluate is the association of TRPV4 with the pathophysiology of ciliated epithelia (airways and reproductive organs). Commentaries have already appeared pointing to TRPV4 as a new molecular target to consider in respiratory pathology, but in relation to its presence in airway smooth muscle and immune cells [40,41]. The more recent data on TRPV4 involvement in mucociliary transport [8] support this view. Deficient functioning of TRPV4 may result in reduced mucus clearance in the airways and failure to transport oocytes and embryos through the oviduct. We believe that this issue will be the focus of future research efforts.
ACKNOWLEDGMENT
Work in the authors’ lab has been funded by the Spanish Ministry of Science and Technology (grant number SAF2003-1240), red HERACLES (Fondo de Investigación Sanitaria), and Generalitat de Catalunya (SGR05-266).
REFERENCES
- 1.
- Zhang MI, O’Neil RG. The diversity of calcium channels and their regulation in epithelial cells. Adv Pharmacol. 1999;46:43. [PubMed: 10332501]
- 2.
- Satir P, Sleigh MA. The physiology of cilia and mucociliary interactions. Annu Rev Physiol. 1990;52:137. [PubMed: 2184754]
- 3.
- Montell C. The TRP superfamily of cation channels. Sci STKE. 2005;2005:re3. [PubMed: 15728426]
- 4.
- Corteling RL, et al. Expression of transient receptor potential C6 and related transient receptor potential family members in human airway smooth muscle and lung tissue. Am J Respir Cell Mol Biol. 2004;30:145. [PubMed: 12871853]
- 5.
- Kowase T, et al. Immunohistochemical localization of growth factor–regulated channel (GRC) in human tissues. Endocr J. 2002;49:349. [PubMed: 12201220]
- 6.
- Arniges M, et al. Swelling-activated Ca2+ entry via TRPV4 channel is defective in cystic fibrosis airway epithelia. J Biol Chem. 2004;279:54062. [PubMed: 15489228]
- 7.
- Nijenhuis T, et al. (Patho)physiological implications of the novel epithelial Ca2+ channels TRPV5 and TRPV6. Pflügers Arch. 2003;446:401. [PubMed: 12748856]
- 8.
- Andrade YN, et al. TRPV4 channel is involved in the coupling of fluid viscosity changes to epithelial ciliary activity. J Cell Biol. 2005;168:869. [PMC free article: PMC2171792] [PubMed: 15753126]
- 9.
- Teilmann SC, et al. Localization of transient receptor potential ion channels in primary and motile cilia of the female murine reproductive organs. Mol Reprod Dev. 2005;71:444. [PubMed: 15858826]
- 10.
- Grimm C, et al. Molecular and functional characterization of the melastatin-related cation channel TRPM3. J Biol Chem. 2003;278:21493. [PubMed: 12672799]
- 11.
- Schlingmann KP, et al. Hypomagnesemia with secondary hypocalcemia is caused by mutations in TRPM6, a new member of the TRPM gene family. Nat Genet. 2002;31:166. [PubMed: 12032568]
- 12.
- Hoenderop JG, et al. Epithelial calcium channels: from identification to function and regulation. Pflügers Arch. 2003;446:304. [PubMed: 12684797]
- 13.
- Tian W, et al. Regulation of TRPV1 by a novel renally expressed rat TRPV1 splice variant. Am J Physiol Renal Physiol. 2005;290:F117. [PubMed: 16091583]
- 14.
- Cohen DM. TRPV4 and the mammalian kidney. Pflügers Arch. 2005;451:168. [PubMed: 15988590]
- 15.
- Nauli SM, Zhou J. Polycystins and mechanosensation in renal and nodal cilia. BIOESSAYS. 2004;26:844. [PubMed: 15273987]
- 16.
- Tsavaler L, et al. Trp-p8, a novel prostate-specific gene, is up-regulated in prostate cancer and other malignancies and shares high homology with transient receptor potential calcium channel proteins. Cancer Res. 2001;61:3760. [PubMed: 11325849]
- 17.
- Corey DP, et al. TRPA1 is a candidate for the mechanosensitive transduction channel of vertebrate hair cells. Nature. 2004;432:723. [PubMed: 15483558]
- 18.
- Liedtke W, et al. Vanilloid receptor-related osmotically activated channel (VR-OAC), a candidate vertebrate osmoreceptor. Cell. 2000;103:525. [PMC free article: PMC2211528] [PubMed: 11081638]
- 19.
- Shin JB, et al. Xenopus TRPN1 (NOMPC) localizes to microtubule-based cilia in epithelial cells, including inner-ear hair cells. Proc Natl Acad Sci USA. 2005;102:12572. [PMC free article: PMC1194908] [PubMed: 16116094]
- 20.
- Yang H, et al. TRPC4 knockdown suppresses epidermal growth factor–induced store-operated channel activation and growth in human corneal epithelial cells. J Biol Chem. 2005;280:32230. [PMC free article: PMC4096720] [PubMed: 16033767]
- 21.
- Nilius B, et al. TRPV4 calcium entry channel: a paradigm for gating diversity. Am J Physiol Cell Physiol. 2004;286:C195–205. [PubMed: 14707014]
- 22.
- Arniges M, et al. Human TRPV4 channel splice variants revealed a key role of ankyrin domains in multimerization and trafficking. J Biol Chem. 2005;281:1580. [PubMed: 16293632]
- 23.
- Afzelius BA. Cilia-related diseases. J Pathol. 2004;204:470. [PMC free article: PMC7167937] [PubMed: 15495266]
- 24.
- Knowles MR, Boucher RC. Mucus clearance as a primary innate defense mechanism for mammalian airways. J Clin Invest. 2002;109:571. [PMC free article: PMC150901] [PubMed: 11877463]
- 25.
- Hoffmann EK, Dunham PB. Membrane mechanisms and intracellular signalling in cell volume regulation. Int Rev Cytol. 1995;161:173. [PubMed: 7558691]
- 26.
- Winters SL, Yeates DB. Roles of hydration, sodium, and chloride in regulation of canine mucociliary transport system. J Appl Physiol. 1997;83:1360. [PubMed: 9338447]
- 27.
- Baltz JM. Osmoregulation and cell volume regulation in the preimplantation embryo. Curr Top Dev Biol. 2001;52:55. [PubMed: 11529430]
- 28.
- McCarty NA, O’Neil RG. Calcium signalling in cell volume regulation. Physiol Rev. 1992;72:1037. [PubMed: 1332089]
- 29.
- Fernandez-Fernandez JM, et al. Maxi K+ channel mediates regulatory volume decrease response in a human bronchial epithelial cell line. Am J Physiol Cell Physiol. 2002;283:C1705. [PubMed: 12388065]
- 30.
- Vazquez E, et al. Defective regulatory volume decrease in human cystic fibrosis tracheal cells because of altered regulation of intermediate conductance Ca2+-dependent potassium channels. Proc Natl Acad Sci USA. 2001;98:5329. [PMC free article: PMC33209] [PubMed: 11309505]
- 31.
- Lock H, Valverde MA. Contribution of the IsK (MinK) potassium channel subunit to regulatory volume decrease in murine tracheal epithelial cells. J Biol Chem. 2000;275:34849. [PubMed: 10995738]
- 32.
- Houtmeyers E, et al. Regulation of mucociliary clearance in health and disease. Eur Respir J. 1999;13:1177. [PubMed: 10414423]
- 33.
- McGrath J, et al. Two populations of node monocilia initiate left–right asymmetry in the mouse. Cell. 2003;114:61. [PubMed: 12859898]
- 34.
- Puchelle E, et al. Rheological properties controlling mucociliary frequency and respiratory mucus transport. Biorheology. 1987;24:557. [PubMed: 3502756]
- 35.
- Sanderson MJ, Dirksen ER. Mechanosensitivity of cultured ciliated cells from the mammalian respiratory tract: implications for the regulation of mucociliary transport. Proc Natl Acad Sci USA. 1986;83:7302. [PMC free article: PMC386704] [PubMed: 3463968]
- 36.
- Rutllant J, et al. Rheological and ultrastructural properties of bovine vaginal fluid obtained at oestrus. J Anat. 2002;201:53. [PMC free article: PMC1570897] [PubMed: 12171476]
- 37.
- Jayaraman S, et al. Submucosal gland secretions in airways from cystic fibrosis patients have normal [Na(+)] and pH but elevated viscosity. Proc Natl Acad Sci USA. 2001;98:8119. [PMC free article: PMC35477] [PubMed: 11427704]
- 38.
- Gao X, et al. Temperature-modulated diversity of TRPV4 channel gating: activation by physical stresses and phorbol ester derivatives through protein kinase C–dependent and –independent pathways. J Biol Chem. 2003;278:27129. [PubMed: 12738791]
- 39.
- Vriens J, et al. Cell swelling, heat, and chemical agonists use distinct pathways for the activation of the cation channel TRPV4. Proc Natl Acad Sci USA. 2004;101:396. [PMC free article: PMC314196] [PubMed: 14691263]
- 40.
- Li S, et al. Transient receptor potential (TRP) channels as potential drug targets in respiratory disease. Cell Calcium. 2003;33:551. [PubMed: 12765700]
- 41.
- Liedtke W, Simon SA. A possible role for TRPV4 receptors in asthma. Am J Physiol Lung Cell Mol Physiol. 2004;287:L269–271. [PubMed: 15246981]
- Review TRP channels activated by extracellular hypo-osmoticity in epithelia.[Biochem Soc Trans. 2007]Review TRP channels activated by extracellular hypo-osmoticity in epithelia.Harteneck C, Reiter B. Biochem Soc Trans. 2007 Feb; 35(Pt 1):91-5.
- TRPV4 channel is involved in the coupling of fluid viscosity changes to epithelial ciliary activity.[J Cell Biol. 2005]TRPV4 channel is involved in the coupling of fluid viscosity changes to epithelial ciliary activity.Andrade YN, Fernandes J, Vázquez E, Fernández-Fernández JM, Arniges M, Sánchez TM, Villalón M, Valverde MA. J Cell Biol. 2005 Mar 14; 168(6):869-74. Epub 2005 Mar 7.
- Transient receptor potential vanilloid type 4 channel expression in chronic rhinosinusitis.[Am J Rhinol. 2008]Transient receptor potential vanilloid type 4 channel expression in chronic rhinosinusitis.Bhargave G, Woodworth BA, Xiong G, Wolfe SG, Antunes MB, Cohen NA. Am J Rhinol. 2008 Jan-Feb; 22(1):7-12.
- Functional expression of transient receptor potential channels in human endometrial stromal cells during the luteal phase of the menstrual cycle.[Hum Reprod. 2015]Functional expression of transient receptor potential channels in human endometrial stromal cells during the luteal phase of the menstrual cycle.De Clercq K, Held K, Van Bree R, Meuleman C, Peeraer K, Tomassetti C, Voets T, D'Hooghe T, Vriens J. Hum Reprod. 2015 Jun; 30(6):1421-36. Epub 2015 Mar 27.
- Review Control of epithelial transport via luminal P2 receptors.[Am J Physiol Renal Physiol. 2003]Review Control of epithelial transport via luminal P2 receptors.Leipziger J. Am J Physiol Renal Physiol. 2003 Mar; 284(3):F419-32.
- The TRPV4 Channel in Ciliated Epithelia - TRP Ion Channel Function in Sensory Tr...The TRPV4 Channel in Ciliated Epithelia - TRP Ion Channel Function in Sensory Transduction and Cellular Signaling Cascades
Your browsing activity is empty.
Activity recording is turned off.
See more...